Abstract
Background
Aging leads to sarcopenia, which is characterized by reduced muscle mass and strength. Many factors, including altered muscle protein turnover, diminished neuromuscular function, hormonal changes, systemic inflammation, and the structure and composition of muscle fibers, play a crucial role in age-related muscle decline. This study explored differences in muscle fiber types contributing to overall muscle function decline in aging, focusing on individuals with hip fractures from falls.
Methods
A pilot study at Chungnam National University Hospital collected muscle biopsies from hip fracture patients aged 20 to 80 undergoing surgical treatment. Muscle biopsies from the vastus lateralis and gluteus maximus were obtained during hip arthroplasty or internal fixation. Handgrip strength, calf and thigh circumference, and bone mineral density were evaluated in individuals with hip fractures from falls. We analyzed the relationships between each clinical characteristic and muscle fiber type.
Results
In total, 26 participants (mean age 67.9 years, 69.2% male) were included in this study. The prevalence of sarcopenia was 53.8%, and that of femoral and lumbar osteoporosis was 19.2% and 11.5%, respectively. Vastus lateralis analysis revealed an age-related decrease in type IIx fibers, a higher proportion of type IIa fibers in women, and an association between handgrip strength and type IIx fibers in men. The gluteus maximus showed no significant correlations with clinical parameters.
The aging process is accompanied by a multitude of physiological changes, some of which have been well-documented in the context of skeletal muscle. One of the most notable changes is a reduction in muscle mass and strength, a condition often referred to as sarcopenia [1]. The etiology of age-related muscle loss is multifactorial, involving changes in muscle protein turnover, neuromuscular function, hormonal balance, and systemic inflammation [1-7]. Within the intricate network of factors that influence age-related muscle changes, the structure and composition of muscle fibers have emerged as a focal point of investigation. Thus, it is important to understand how alterations in muscle fiber types, size, and quality contribute to the overall decline in muscle function with advancing age.
Muscle fibers are composed of contractile units called sarcomeres, which contain thin and thick filaments, as well as other structural proteins. These filaments interact to generate muscle contractions through adenosine triphosphate (ATP)-dependent processes. According to the myosin heavy chain isoform expressed, muscle fiber types are classified as type I, IIA, IIB, or IIX. Aging leads to a transition in muscle fiber types, characterized by a gradual decrease in the number and size of type II fibers [8].
The decline in muscle mass becomes more significant after the age of 60 [1]. This reduction in muscle mass with aging is primarily attributed to a simultaneous decline in both slow-twitch (type I) and fast-twitch (type II) muscle fibers, with a particularly notable decrease in the size of type II fibers [8]. The diminishing presence of type II muscle fibers as age advances is closely linked to a significant reduction in the population of satellite cells within these fibers [9]. Fast-twitch type II muscle fibers are further classified into type IIa and type IIx in humans [10]. Type IIa fibers display characteristics of both type I and type IIx fibers, whereas type IIx fibers generate the highest force but are inefficient, characterized by their high myosin ATPase activity, low oxidative capacity, and dependence on anaerobic metabolism [11]. Further research indicates that the age-related decline in muscle mass is predominantly due to a reduction in the size of type IIx fibers [12]. However, it has yet to be established how muscle fiber type composition changes in young and old patients who have experienced hip fractures due to falls.
Sexual dimorphism in human skeletal muscle has been extensively documented and is a subject of fascination in various fields, including physiology, sports science, and medicine [13-15]. Recent research has highlighted significant differences in the structure, function, and composition of muscles between males and females [13-15]. Notably, these marked variations in muscle fiber composition are a key aspect of this dimorphism, significantly affecting muscular performance and the risk of developing musculoskeletal and metabolic disorders. Therefore, a thorough examination of the differences in muscle fiber composition between sexes, especially in relation to age-related muscle loss, is of considerable importance. This study aimed to elucidate the complex relationships between sex, muscle fiber attributes, and the physiological processes that drive muscle deterioration with age.
In the current study, we collected muscle biopsies from patients who were admitted to the hospital due to hip fractures caused by falls. These biopsies were used to assess skeletal muscle fiber characteristics in samples from two distinct muscle types: the vastus lateralis and gluteus maximus. The aim was to explore differences in fiber composition based on age and sex within these two muscle groups.
This pilot study was conducted at Chungnam National University Hospital in South Korea between 2019 and 2023. The study followed a single-center, prospective, single-arm design. Participants 20 to 80 years of age from both sexes were recruited. Patients admitted to the Orthopaedic Surgery Department of the hospital for surgical treatment of femoral neck fracture, intertrochanteric fracture, femoral fracture, or hip fracture were eligible for inclusion in the study. Individuals being treated for cancer, experiencing current psychiatric symptoms, or planning to become pregnant were excluded.
All study participants were fully informed about the study goals and methods, and provided their informed consent by signing a consent form. The study was conducted in accordance with the Declaration of Helsinki, and the Institutional Review Board of Chungnam National University Hospital (2019-06-063) reviewed and approved the protocol.
A digital handgrip dynamometer (TKK-5401, Takei Scientific Instruments Co. Ltd., Niigata, Japan) was utilized to assess handgrip strength. The device was calibrated to position the fingers at a 90° angle during flexion. Due to discomfort from femoral fractures, subjects were measured while reclining in bed. They were instructed to elevate their upper body and extend their arms forward with elbows fully straightened for the assessment. The dynamometer was operated with one hand, and subjects were asked to exert maximum effort for a duration of three seconds during the squeeze. Measurements were taken for both hands, and the higher value of the two was recorded, precise to one decimal place (0.1 kg). Measurements excluded any arm that had sustained an injury or had undergone a procedure, such as the creation of an arteriovenous fistula.
The circumference was measured on the non-operated leg. The participant was instructed to fully extend the leg to be measured. The calf circumference was taken at its most prominent point with a tape measure. The thigh circumference was measured 10 cm above the knee using a tape measure. All measurements were recorded in centimeters and rounded to one decimal place (0.1 cm).
Imaging was conducted at the bone densitometry facility of the Rehabilitation Center at Chungnam National University Hospital on the day preceding surgery. The equipment used was a HOLOGIC Discovery W (serial number 87133, software version 13.4.1, Hologic, Santa Clara, CA, USA). Participants were asked to remove all metallic items and lie down on the scanning table in a supine position with their arms at their sides. A large sponge was placed between their legs to separate them to the required angle. We measured the bone mineral density (BMD) of the non-operated leg and manually identified the neck, trochanter, intertrochanteric region, and total femur, as well as L1, L2, L3, L4, and the total lumbar spine before starting the scan. Following the acquisition, we confirmed the total BMD and T-scores for the femur and lumbar spine for inclusion in the study analysis.
During the orthopedic surgery, tissue samples were taken from both the vastus lateralis and the gluteus maximus. Each sample measured approximately 0.2 cm×0.2 cm×0.2 cm. The tissue was harvested from areas that were less likely to be affected by electric cautery, using a scalpel, pituitary rongeur, and forceps. The collected muscle tissue was promptly transported to the laboratory, where it was frozen with FSC22 (Leica Biosystems, Wetzlar, Germany) frozen section medium and stored at –80°C in a deep freezer. Subsequently, frozen sections were obtained and the components within were identified using electron microscopy after appropriate staining procedures (Fig. 1).
Muscle tissue was embedded in Tissue-Tek OCT compound (Leica Biosystems), and serial 5-μm-thick sections of the tissue were cut using a cryomicrotome. Immunofluorescence staining for myosin heavy chain in muscle tissue was carried out sequentially, beginning with fixation, followed by incubation with primary antibodies against Myh IIa (SC-71, 1:200) and Myh IIx (6H1, 1:200) from the Developmental Studies Hybridoma Bank, in a 5% goat serum blocking solution.
All continuous variables are reported as the mean±standard error of the mean. Statistical significance was defined as P<0.05 and obtained using the two-tailed Student t test or two-way analysis of variance, as appropriate. Categorical variables were presented as percentages. Pearson correlation coefficients were employed to assess the strength of the relationship between continuous variables. The calculation of statistical significance was predicated on the assumption that the values follow a Gaussian distribution. Statistical analyses were conducted using GraphPad Prism version 10 (GraphPad LLC, La Jolla, CA, USA).
A total of 26 patients were enrolled to evaluate muscle fiber types in the vastus lateralis and gluteus maximus. The patients ranged in age from 40 to 88 years, with an average of 67.9 years. Among the participants, 18 out of 26 were men, accounting for 69.2% of total subjects (Table 1). The mean values of the body mass index, calf circumference, and thigh circumference were 21.82 kg/cm2, 30.60 cm, and 41.39 cm, respectively. In the handgrip strength test, five female and eight male participants had low muscle strength (<18 kg for female; <28 kg for male). According to the results obtained from handgrip strength assessments, approximately 53.8% of the participants exhibited signs of sarcopenia. The prevalence of osteoporosis, as determined by BMD measurements of the femur, was 19.2%, while the prevalence based on BMD measurements of the lumbar spine was approximately 11.5%.
To identify correlations between muscle fiber types and the clinical characteristics of participants, we initially employed immunostaining to quantify the fast-twitch muscle fibers in the vastus lateralis and gluteus maximus. We analyzed the correlations between fiber type and age, as well as handgrip strength, among participants with femur fractures (Fig. 1). In the vastus lateralis, type IIa fibers exhibited no correlation with age or handgrip strength (Fig. 2A, B). Conversely, the frequency of type IIx fibers demonstrated a negative correlation with age and a statistically significant positive correlation with handgrip strength (Fig. 2C, D). There was no significant correlation between the ratio of type IIx to type IIa fiber frequency and either age or handgrip strength (Fig. 2E, F). In the gluteus maximus, neither type IIa nor IIx fibers demonstrated statistically significant correlations with age, sex, or handgrip strength (Supplemental Fig. S1).
We conducted further analyses by dividing the subjects into subgroups based on age (young and old), sex (male and female), and handgrip strength levels (low and high). The proportion of type IIa slow muscle fibers did not differ between the young and old age groups (Fig. 3A). However, the elderly group exhibited a significant reduction in type IIx fast muscle fibers compared to the younger group (Fig. 3A). An analysis of muscle fiber types in relation to sex revealed that in women, there were significantly more type IIa fibers and significantly fewer (Fig. 3B). In terms of handgrip strength, no difference was observed in the distribution of type IIa fibers. Nonetheless, participants with higher handgrip strength, particularly among male patients, demonstrated a greater proportion of type IIx fibers (Fig. 3C, Supplemental Table S1).
We then analyzed the relationship between body weight and muscle fiber type. The proportion of type IIa fibers was similar in both the higher and lower body weight groups (Fig. 4A). However, the presence of type IIx fibers was significantly different between these groups (Fig. 4A). No significant differences were observed in muscle fiber types when comparing body mass index, calf circumference, and thigh circumference (Fig. 4B-D). Subsequently, we explored the relationship between bone density and muscle composition. The proportions of type IIa and IIx fibers were consistent across patients with varying levels of BMD (Supplemental Fig. S2). Furthermore, differences in serum calcium, vitamin D, C-telopeptide, procollagen type 1 N-terminal propeptide, alkaline phosphatase, and glucose levels did not influence muscle fiber composition (Supplemental Fig. S3).
Contrary to the findings from the vastus lateralis, our analysis of the gluteus maximus showed no significant correlation between muscle fiber composition and either age or handgrip strength test outcomes (Supplemental Fig. S4). Furthermore, type I muscle fibers, unlike type II fibers in the vastus lateralis, did not exhibit a significant correlation with age, sex, or handgrip strength test results in either the vastus lateralis or the gluteus maximus (Supplemental Figs. S5, S6).
The current study revealed substantially lower type IIx muscle fiber composition among older adults who sustained hip fractures due to low-energy falls than among young adults with hip fractures. In patients with hip fractures, women showed a higher prevalence of type IIa fiber composition in the vastus lateralis muscle, yet a lower type IIx fiber composition than men. Furthermore, we found that hip fracture patients with lower handgrip strength had a diminished type IIx fiber composition in the vastus lateralis, as illustrated in Fig. 3C.
According to data from the Centers for Disease Control and Prevention, more than 300,000 individuals aged 65 years and older are hospitalized each year in the United States due to hip fractures [16]. Falls—in particular, sideways falls—account for over 95% of hip fractures [17]. Notably, about three-quarters of all hip fractures occur in women, who are more prone to falls than men [18]. Reduced muscle mass and strength are likely underlying factors for most hip fracture risk factors and are conceptually linked to falls. Given that the reduction in muscle fiber size and composition is specific to fiber type, with type II fibers in the elderly being 10% to 40% smaller than those in young control subjects [19], we hypothesized that older adults hospitalized for hip surgery after a fall would show significant changes in type II muscle fiber composition. Our research demonstrated that type IIx muscle fibers were 10.0%±3.1% smaller in older adults with hip fractures compared to young adults with hip fractures. We also observed a marked shift in the frequency distribution of muscle fibers with age. Additionally, there was a significant reduction in type IIx fibers among female hip fracture patients and those with lower handgrip strength. Based on these findings, we propose that the atrophy and decreased frequency of type IIx muscle fibers contribute to the accelerated muscle loss associated with falls in clinically compromised and frail elderly individuals, such as those who have experienced a hip fracture.
Aging brings about a gradual decline in muscle mass, typically beginning in the third or fourth decade, with an estimated loss of 3% to 8% per decade [20,21]. This decline becomes more pronounced after the sixth decade [1,20]. The reduction in muscle mass that accompanies aging can be attributed to two main factors: a simultaneous decrease in both type I (slow-twitch) and type II (fast-twitch) muscle fibers, along with a more significant reduction in the size of type II fibers [8]. Notably, studies on older women with hip fractures have highlighted considerable atrophy in type II muscle fibers [22]. Type II fast-twitch muscle fibers are further divided into type IIa and type IIx [10]. Type IIa fibers display characteristics of both type I and type IIx fibers, whereas type IIx fibers generate the highest force but are highly inefficient due to their elevated myosin ATPase activity, low oxidative capacity, and strong dependence on anaerobic metabolism [11]. Further research has shown that the age-related decline in muscle mass is primarily due to a reduction in the size of type IIx fibers [12]. Consistent with these findings, our research also revealed significantly lower type IIx muscle fiber composition in older adults who sustained hip fractures from falls than in young adults with similar injuries. However, an important question remains regarding changes in fiber types in the context of clinically diagnosed sarcopenia versus general aging.
While it is well-established that sex-based differences exist in skeletal muscle fiber composition [13-15], the precise reasons for these disparities in muscle fiber types are not fully understood. Previous studies have indicated that men typically have larger cross-sectional areas for all muscle fiber types and a higher percentage of type II muscle fibers [23]. In contrast, women tend to have a greater distribution and higher percentage of type I fibers [23]. As a result, women usually have higher type I/II fiber area ratios compared to men, whereas men have higher type II/I fiber ratios than women. In our current study, we observed a higher prevalence of type IIa fiber composition in the vastus lateralis among female patients, but a lower type IIx fiber composition compared to male patients. Although sexspecific hormones such as estrogen and testosterone are known to play roles in skeletal muscle growth, fiber size, and to some extent, contractile function, more research is necessary to clarify sex-specific differences in human sarcopenia.
The study has several limitations. First, its descriptive nature is reflected in the relatively small sample size, which includes individuals from a single ethnicity. Second, measurements of handgrip strength and muscle mass were taken after the occurrence of hip fractures. Third, since systemic factors and myokines can influence muscle fiber composition or contribute to sarcopenia [24-27], we cannot draw definitive conclusions about the relationship between muscle fiber composition and specific myokines. Fourth, we were unable to provide a definitive explanation for the more pronounced reduction in type IIx muscle fibers in the vastus lateralis compared to the gluteus maximus. Additionally, the most critical and practical limitation of this study is that we were unable to compare the distribution of muscle fibers in older patients hospitalized for hip surgery with that of healthy individuals who had not experienced falls or fractures.
In summary, frail elderly individuals and women at risk of fall-related hip fractures experience significant reductions in both the size and composition of type IIx muscle fibers. These observations indicate that the pronounced loss of type IIx muscle fibers may be linked to decreased muscle strength and functional capacity, thereby heightening the risk of falls and fractures. Lastly, further research is needed to determine whether the extent of muscle atrophy acts as a predictive factor for post-surgery recovery at both the muscle fiber and whole-muscle levels. Such investigations could improve the precision of intervention strategies aimed at preventing fall-related hip fractures and facilitating post-fracture recovery. Ultimately, these findings could help reduce the burden of illness associated with hip fractures.
Supplementary Material
Supplemental Table S1.
Clinical Characteristics of the Study Subjects Divided by Age, Gender, and Result of Handgrip Strength
Supplemental Fig. S1.
Distribution and quantitative analysis of type IIa and type IIx muscle fibers of gluteus maximus. (A) Age-related differences in muscle fiber composition: young vs. old. (B) Gender-related variations in muscle fiber composition: male vs. female. (C) Association between handgrip strength and muscle fiber composition: high vs. low grip strength. Data are expressed as mean±standard error of the mean.
Supplemental Fig. S2.
Relationship between bone mineral density (BMD) and muscle fiber characteristics of vastus lateralis. (A) Comparison of type IIa and IIx muscle fiber proportions by femur bone mineral density. (B) Comparison of type IIa and IIx muscle fiber proportions by lumbar spine bone mineral density. Data are expressed as mean±standard error of the mean.
Supplemental Fig. S3.
Relationship between serum chemistry and muscle fiber characteristics of vastus lateralis. Comparison of type IIa and IIx muscle fiber proportions by (A) serum calcium, (B) vitamin D, (C) C-telopeptide (CTX), (D) procollagen type 1 N-terminal propeptide (P1NP), (E) alkaline phosphatase (ALP), and (F) glucose levels. Data are expressed as mean±standard error of the mean.
Supplemental Fig. S4.
Relationship between fiber type in gluteus maximus and age or handgrip strength in patients with femur fracture. (A) Correlation analysis of fiber type IIa proportion and age. (B) Correlation analysis of fiber type IIa proportion and handgrip strength. (C) Correlation analysis of fiber type IIx proportion and age. (D) Correlation analysis of fiber type IIx proportion and handgrip strength. The data were evaluated by Spearman’s correlation analysis.
Supplemental Fig. S5.
Differences in type I fibers in vastus lateralis based on age, gender, and handgrip strength. (A) The proportion of type I fiber was compared between young and old group. (B) The proportion of type I fiber was compared between men and women. (C) The proportion of type I fiber was compared between the top 50% and bottom 50% of handgrip strength. Data are expressed as mean±standard error of the mean.
Supplemental Fig. S6.
Differences in type I fibers in gluteus maximus based on age, gender, and handgrip strength. (A) The proportion of type I fiber was compared between young and old group. (B) The proportion of type I fiber was compared between men and women. (C) The proportion of type I fiber was compared between the top 50% and bottom 50% of handgrip strength. Data are expressed as mean±standard error of the mean.
ACKNOWLEDGMENTS
This work was supported by a grant from the Korea Health Technology R&D Project, through the Korea Health Industry Development Institute (KHIDI), funded by the Ministry of Health & Welfare, Republic of Korea (grant number: HR22C1734 and HI23C153400). Hyon-Seung Yi was supported by the Basic Science Research Program, through the National Research Foundation of Korea (NRF), funded by the Ministry of Science, ICT, and Future Planning, Korea (NRF-2023R1A2C3006220, NRF-2021R1A5A8029876), the Korean Endocrine Society of KES Research Award 2023 and the Chungnam National University Hospital Research Fund (2023).
REFERENCES
1. Larsson L, Degens H, Li M, Salviati L, Lee YI, Thompson W, et al. Sarcopenia: aging-related loss of muscle mass and function. Physiol Rev. 2019; 99:427–511.


2. Liguori I, Russo G, Aran L, Bulli G, Curcio F, Della-Morte D, et al. Sarcopenia: assessment of disease burden and strategies to improve outcomes. Clin Interv Aging. 2018; 13:913–27.


3. Ju SH, Yi HS. Clinical features and molecular mechanism of muscle wasting in end stage renal disease. BMB Rep. 2023; 56:426–38.


4. Setoyama D, Lee HY, Moon JS, Tian J, Kang YE, Lee JH, et al. Immunometabolic signatures predict recovery from thyrotoxic myopathy in patients with Graves’ disease. J Cachexia Sarcopenia Muscle. 2022; 13:355–67.


5. Lee JC, Song BS, Kang YM, Kim YR, Kang YE, Lee JH, et al. Effect of thyroid-stimulating hormone suppression on muscle function after total thyroidectomy in patients with thyroid cancer. Front Endocrinol (Lausanne). 2021; 12:769074.


6. Park BS, Yoon JS. Relative skeletal muscle mass is associated with development of metabolic syndrome. Diabetes Metab J. 2013; 37:458–64.


7. Tian J, Chung HK, Moon JS, Nga HT, Lee HY, Kim JT, et al. Skeletal muscle mitoribosomal defects are linked to low bone mass caused by bone marrow inflammation in male mice. J Cachexia Sarcopenia Muscle. 2022; 13:1785–99.


8. Nilwik R, Snijders T, Leenders M, Groen BB, van Kranenburg J, Verdijk LB, et al. The decline in skeletal muscle mass with aging is mainly attributed to a reduction in type II muscle fiber size. Exp Gerontol. 2013; 48:492–8.


9. Verdijk LB, Koopman R, Schaart G, Meijer K, Savelberg HH, van Loon LJ. Satellite cell content is specifically reduced in type II skeletal muscle fibers in the elderly. Am J Physiol Endocrinol Metab. 2007; 292:E151–7.


10. Smerdu V, Karsch-Mizrachi I, Campione M, Leinwand L, Schiaffino S. Type IIx myosin heavy chain transcripts are expressed in type IIb fibers of human skeletal muscle. Am J Physiol. 1994; 267(6 Pt 1):C1723–8.


11. Casey A, Constantin-Teodosiu D, Howell S, Hultman E, Greenhaff PL. Metabolic response of type I and II muscle fibers during repeated bouts of maximal exercise in humans. Am J Physiol. 1996; 271(1 Pt 1):E38–43.


12. Wang Y, Pessin JE. Mechanisms for fiber-type specificity of skeletal muscle atrophy. Curr Opin Clin Nutr Metab Care. 2013; 16:243–50.


13. Li S, Schonke M, Buurstede JC, Moll TJ, Gentenaar M, Schilperoort M, et al. Sexual dimorphism in transcriptional and functional glucocorticoid effects on mouse skeletal muscle. Front Endocrinol (Lausanne). 2022; 13:907908.


14. Monaco CM, Bellissimo CA, Hughes MC, Ramos SV, Laham R, Perry CG, et al. Sexual dimorphism in human skeletal muscle mitochondrial bioenergetics in response to type 1 diabetes. Am J Physiol Endocrinol Metab. 2020; 318:E44–51.


15. Smith GI, Mittendorfer B. Sexual dimorphism in skeletal muscle protein turnover. J Appl Physiol (1985). 2016; 120:674–82.


16. Schneider AM, Mucharraz C, Denyer S, Brown NM. Prolonged hospital stay after arthroplasty for geriatric femoral neck fractures is associated with increased early mortality risk after discharge. J Clin Orthop Trauma. 2022; 26:101785.


17. Hayes WC, Myers ER, Morris JN, Gerhart TN, Yett HS, Lipsitz LA. Impact near the hip dominates fracture risk in elderly nursing home residents who fall. Calcif Tissue Int. 1993; 52:192–8.


18. Banks E, Reeves GK, Beral V, Balkwill A, Liu B, Roddam A, et al. Hip fracture incidence in relation to age, menopausal status, and age at menopause: prospective analysis. PLoS Med. 2009; 6:e1000181.


19. Frontera WR, Suh D, Krivickas LS, Hughes VA, Goldstein R, Roubenoff R. Skeletal muscle fiber quality in older men and women. Am J Physiol Cell Physiol. 2000; 279:C611–8.


20. Melton LJ 3rd, Khosla S, Crowson CS, O’Connor MK, O’Fallon WM, Riggs BL. Epidemiology of sarcopenia. J Am Geriatr Soc. 2000; 48:625–30.


21. Dao T, Green AE, Kim YA, Bae SJ, Ha KT, Gariani K, et al. Sarcopenia and muscle aging: a brief overview. Endocrinol Metab (Seoul). 2020; 35:716–32.


22. Kramer IF, Snijders T, Smeets JS, Leenders M, van Kranenburg J, den Hoed M, et al. Extensive type II muscle fiber atrophy in elderly female hip fracture patients. J Gerontol A Biol Sci Med Sci. 2017; 72:1369–75.


23. Nuzzo JL. Sex differences in skeletal muscle fiber types: a meta-analysis. Clin Anat. 2024; 37:81–91.


24. Ahn SH, Jung HW, Lee E, Baek JY, Jang IY, Park SJ, et al. Decreased serum level of sclerostin in older adults with sarcopenia. Endocrinol Metab (Seoul). 2022; 37:487–96.


25. Choi JH, Seo JW, Lee MY, Lee YT, Yoon KJ, Park CH. Association between elevated plasma homocysteine and low skeletal muscle mass in asymptomatic adults. Endocrinol Metab (Seoul). 2022; 37:333–43.


Fig. 1.
Study design. We obtained muscle tissue samples from the vastus lateralis and gluteus maximus of patients with hip fractures, analyzed the composition of muscle fiber types, and endeavored to establish correlations between fiber types and clinical indices. BMD, bone mineral density.
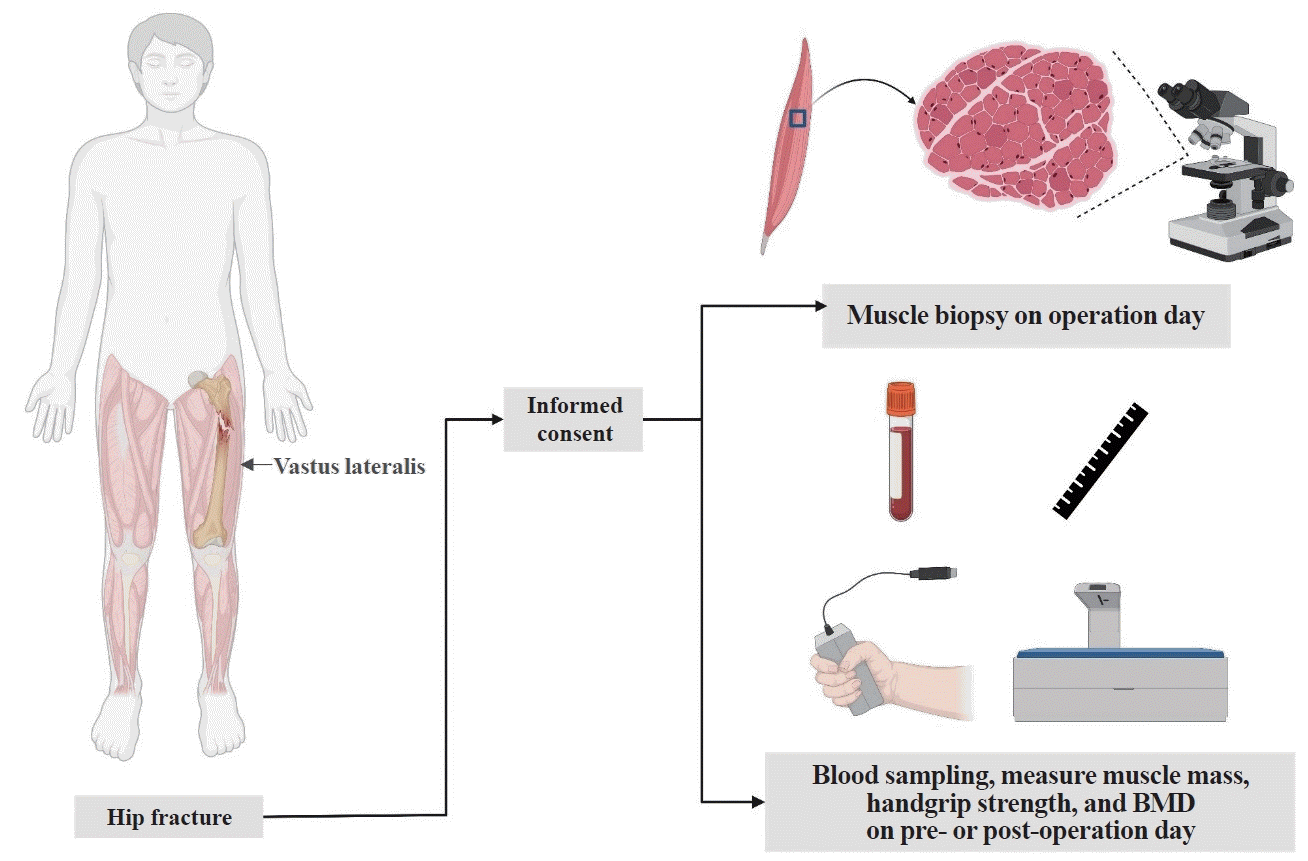
Fig. 2.
Relationship between fiber types in the vastus lateralis and age or handgrip strength in patients with femur fractures. (A) Correlation analysis of the proportion of type IIa fibers and age. (B) Correlation analysis of the proportion of type IIa fibers and handgrip strength. (C) Correlation analysis of the proportion of type IIx fibers and age. (D) Correlation analysis of the proportion of type IIx fibers and handgrip strength. (E) Correlation analysis of the proportion of type IIx/IIa fibers and age. (F) Correlation analysis of the proportion of type IIx/IIa fibers and handgrip strength. The data were evaluated using Pearson and Spearman correlation analysis. rp, Pearson correlation coefficient; rs, Spearman rank correlation coefficient.
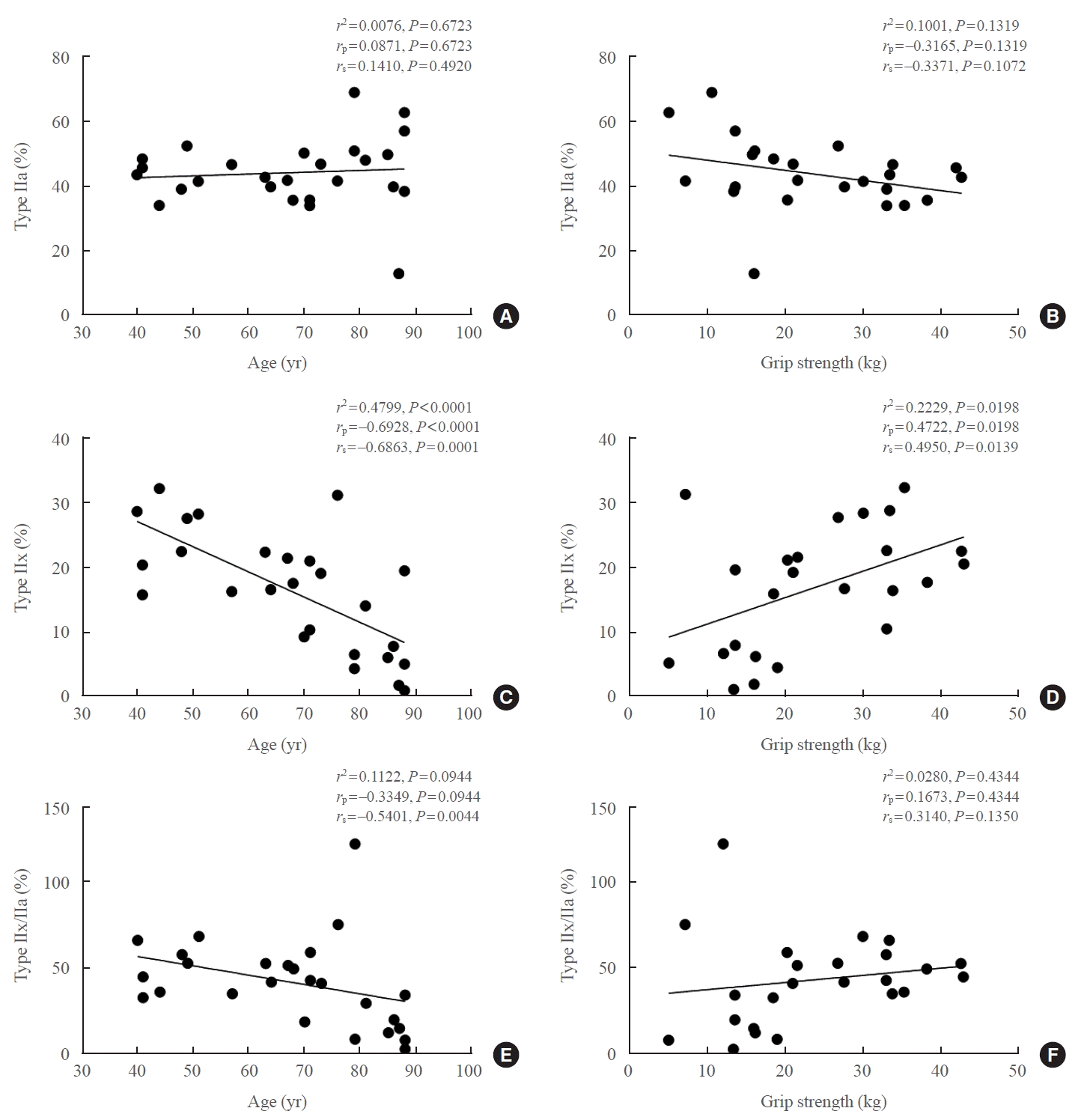
Fig. 3.
Differences in fiber types in the vastus lateralis based on age, sex, and handgrip strength in patients with femur fracture. The fasttwitch muscle fibers were evaluated using immunostaining in the vastus lateralis. (A) The proportion of type IIa and IIx fibers was compared between the young and old groups. (B) The proportion of type IIa and IIx fibers was compared between men and women. (C) The proportion of type IIa and IIx fibers was compared between the top 50% and bottom 50% of handgrip strength. Data are expressed as mean±standard error of the mean. aP<0.05 compared with the corresponding controls.
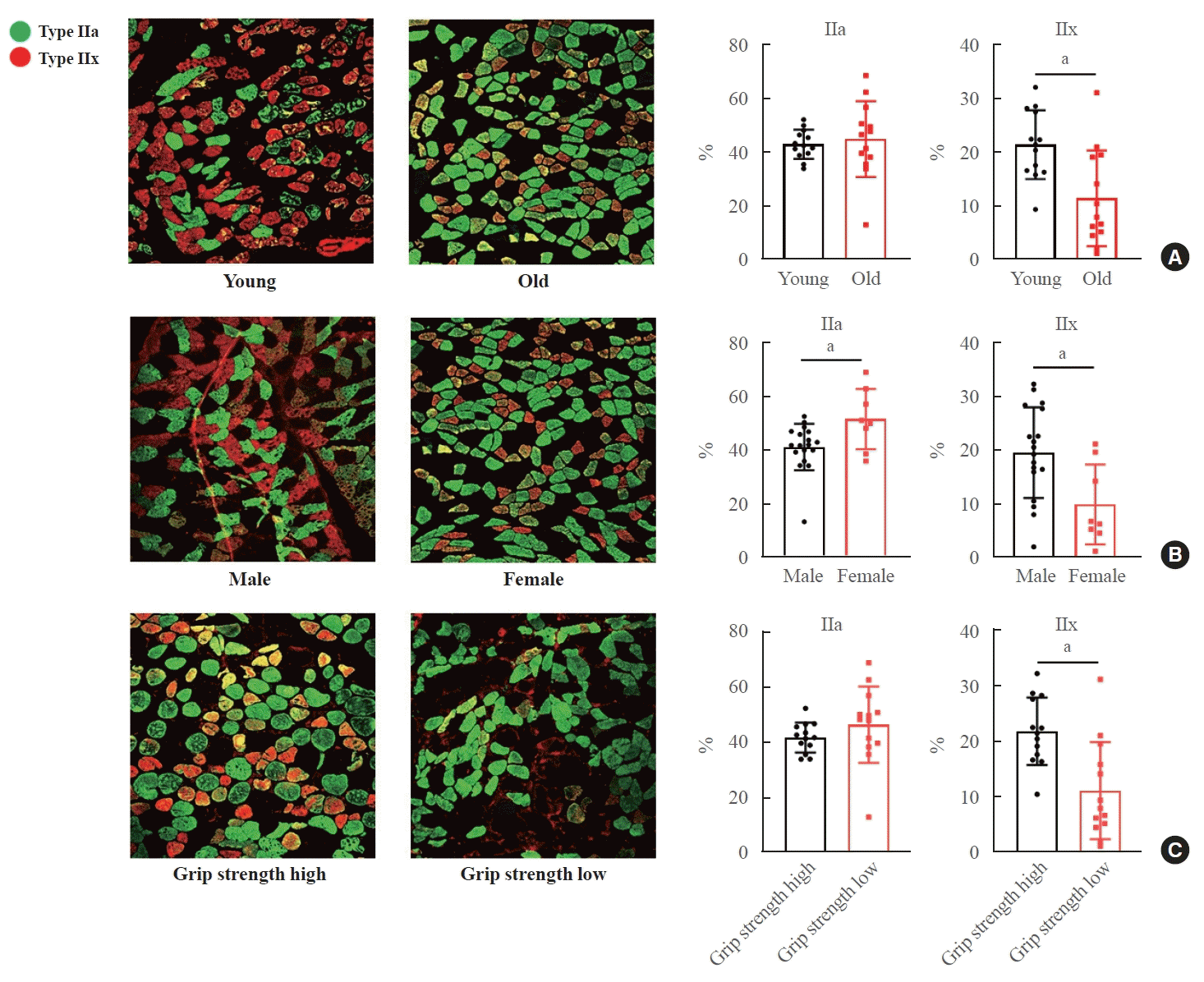
Fig. 4.
Differences in fiber types in the vastus lateralis based on body weight, body mass index, and calf or thigh circumference in patients with femur fractures. The disparities in the proportions of type IIa and IIx fibers according to (A) body weight, (B) body mass index (BMI), (C) calf circumference, and (D) thigh circumference were assessed. Data are expressed as mean±standard error of the mean. aP<0.05 compared with the corresponding controls.
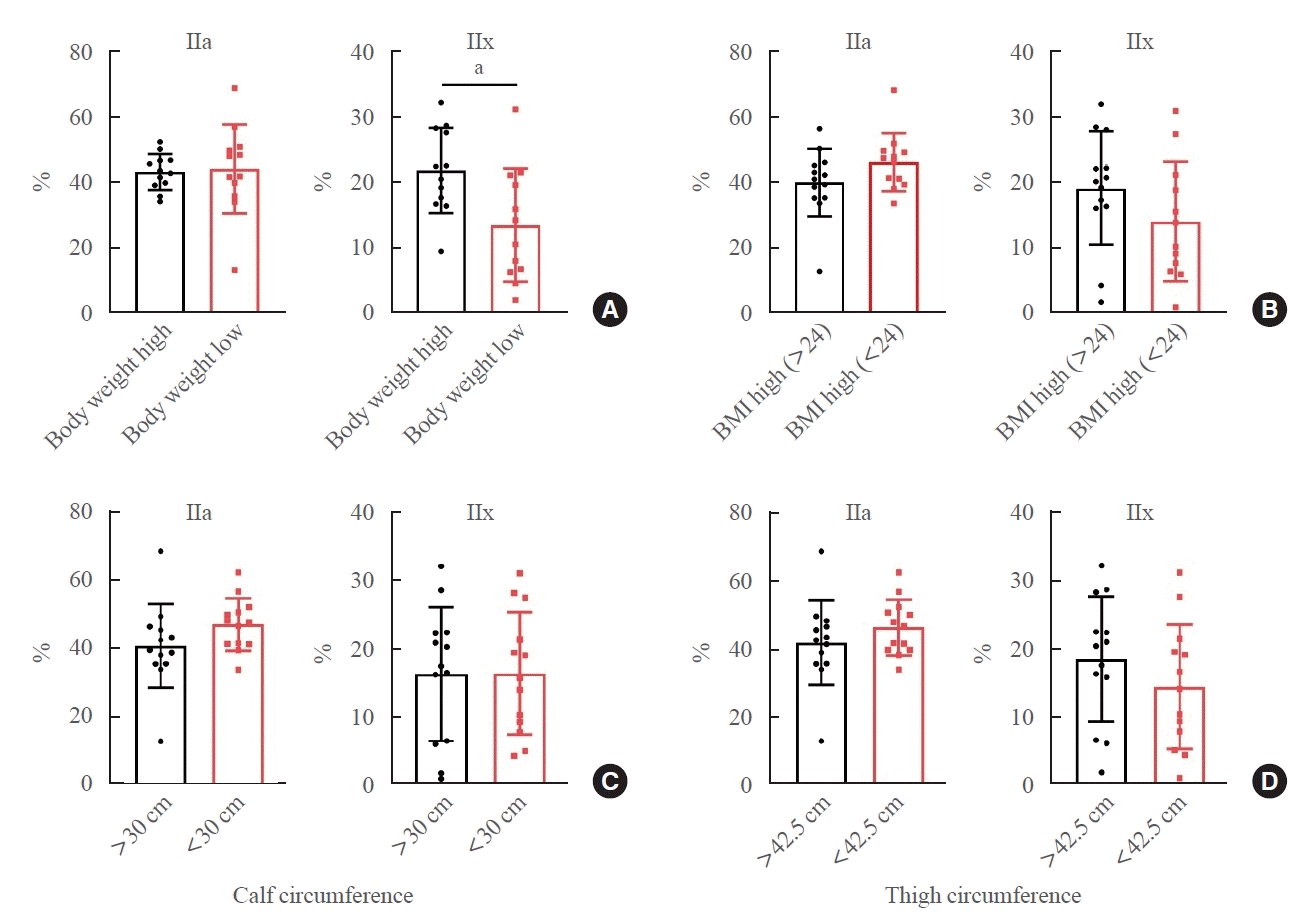
Table 1.
Clinical Characteristics of the Study Participants by Sex