Abstract
Background
Methods
Results
Supplementary Materials
Notes
Ethics Statement
All procedures performed in the current study were approved by the Research Ethics Committee of the University of Kufa (IRB No.2019-01- 500) in accordance with the 1964 Helsinki declaration and its later amendments and revision in 2013. Formal written informed consent was not required after a waiver by the University of Kufa Research Ethics Committee.
Availability of Data and Material
All data generated or analyzed during the study are included in this published article (and its supplementary information files).
Author contributions
CConceptualization: ASJ, MMS. Data curation: ASJ. Formal analysis: ASJ, MMS. Methodology: ASJ, HSAH, MMS. Project administration: HSAH, MMS. Resources: HSAH. Software: ASJ, MMS. Supervision: ASJ, AAY. Validation: MMS, HSAH. Visualization: HSAH, MMS. Writing—original draft: ASJ, AAY, KAM. Writing—review & editing: AAY, ASJ, KAM, HSAH. Approval of final manuscript: all authors.
References







































Fig. 1.
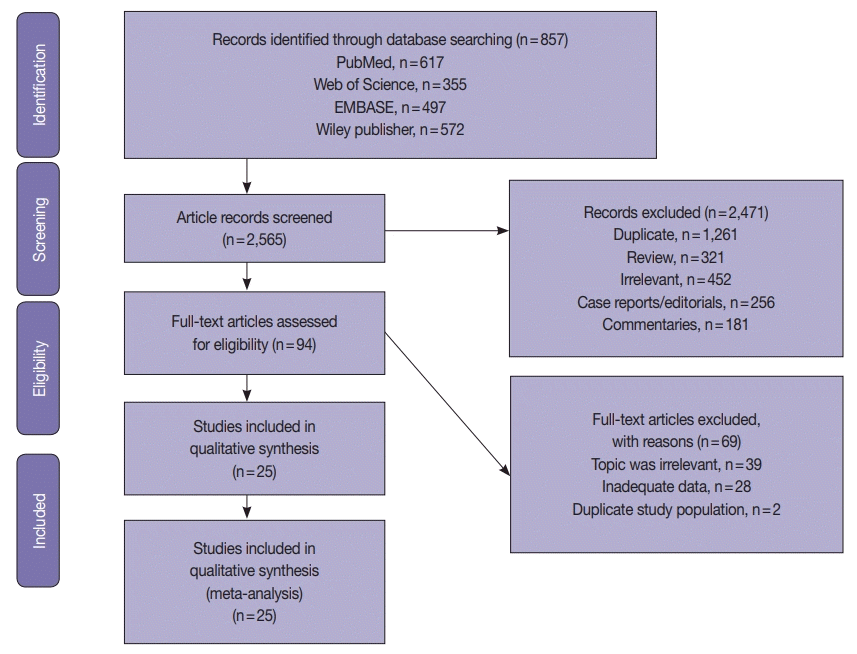
Fig. 2.
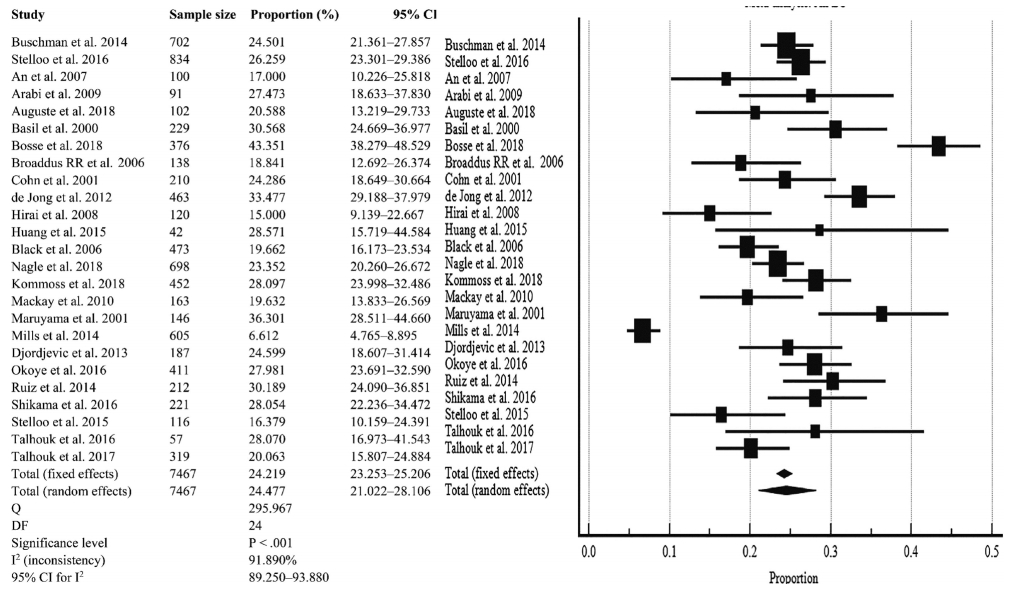
Fig. 3.
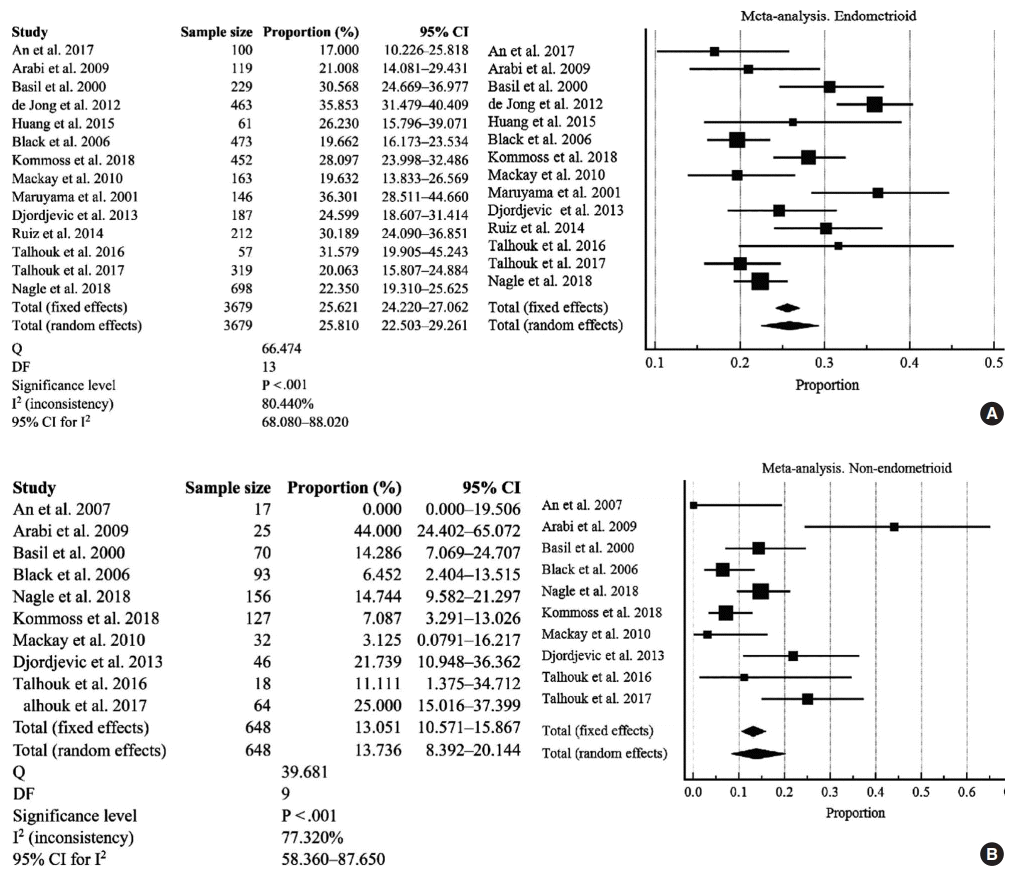
Table 1.
Study | Sample size | D-MMR EC (%) | Study site | Detection method and D-MMR marker |
Clinicopathologic characteristics |
||||||
---|---|---|---|---|---|---|---|---|---|---|---|
D-MMR subjects Grade I-II EC | D-MMR Grade III EC | D-MMR Stage I-II EC | D-MMR Stage III-VI EC | D-MMR subjects with MI < 50%a | D-MMR subjects with MI > 50%b | D-MMR EC with positive LVI | |||||
Buchanan et al. (2014) [6] | 702 | 24.501 | Australia | IHC amarkers: MLH1, MSH2, MSH6, PMS2 (protein loss or presence) | NR | NR | NR | NR | NR | NR | NR |
Stelloo et al. (2016) [7] | 834 | 26.259 | Nether-lands | IHC markers: MLH1, MSH2, MSH6, PMS2 proteins | NR | NR | NR | NR | 148 | 71 | 19 |
An et al. (2007) [8] | 93 | 18.280 | South Korea | PCR | 12 | 5 | NR | NR | 7 | 10 | 7 |
Arabi et al. (2009) [9] | 91 | 27.473 | USA | IHC markers: MLH1, MSH2, MSH6 proteins | NR | NR | 13 | 12 | 15 | 10 | 14 |
Auguste et al. (2018) [10] | 102 | 20.588 | USA, Canada | IHC markers: MLH1, MLH2, MSH6, PMS2 | NR | NR | NR | NR | NR | NR | NR |
Basil et al. (2000) [11] | 229 | 30.568 | USA | PCR markers: BAT26, BAT25, D5S346, D2S123, D17S250 | NR | NR | 61 | 9 | NR | NR | NR |
Bosse et al. (2018) [12] | 376 | 43.351 | USA, Canada, UK | IHC markers: MLH1, MSH2, MSH6, PMSU proteins | NR | NR | NR | NR | NR | NR | NR |
Broaddus et al. (2006) [13] | 138 | 18.841 | USA | IHC markers: MLH1 and MSH2 (n = 50) sequencing of MLH1 and MSH2 genes | NR | NR | 16 | 10 | NR | NR | 14 |
Cohn et al. (2001) [14] | 210 | 24.286 | USA | PCR markers: BAT-25, BAT-26, D5S346, D2S123, D17S250 | NR | NR | NR | NR | NR | NR | NR |
de Jong et al. (2012) [15] | 463 | 33.477 | Netherlands | IHC markers: MLH1, MSH2, MSH6 proteins | NR | NR | NR | NR | NR | NR | 98 |
Hirai et al. (2008) [16] | 120 | 15.000 | Japan | Sequencing of MLH1, MLH2, MSH6 genes | NR | NR | NR | NR | NR | NR | NR |
Huang et al. (2015) [17] | 42 | 28.571 | Taiwan | IHC (MLH1, PMS2, MSH6, and MSH2), PCR (BAT-25, BAT-26, NR-21, NR-24, and MONO-27) | NR | NR | NR | NR | NR | NR | NR |
Black et al. (2006) [18] | 473 | 19.662 | USA | PCR markers: BAT25, BAT26, D2S123M, D5S346, D17S250 | 72 | 21 | 77 | 16 | 67 | 25 | 30 |
Nagle et al. (2018) [19] | 698 | 19.198 | Australia | IHC (MLH1, MSH2, MSH6, PMS2), MLH1 methylation testing was conducted for all cases with MLH1/PMS2 loss | NR | NR | 125 | 17 | NR | NR | 47 |
Kommoss et al. (2018) [20] | 452 | 28.097 | USA, Canada, Germany | IHC to detect MMR protein loss in markers PMS2 and MSH6 | 102 | 25 | NR | NR | 44 | 58 | 25 |
Mackay et al. (2010) [21] | 163 | 19.632 | Canada | PCR testing of MSI, markers are BAT25/26 | 21 | 11 | NR | NR | NR | NR | NR |
Maruyama et al. (2001) [22] | 146 | 36.301 | Japan | IHC marker MSH2, MLH1 | NR | NR | NR | NR | NR | NR | NR |
Mills et al. (2014) [23] | 605 | 6.612 | USA | IHC of MLH1, MSHU, MSH6, PMS2 proteins, PCR confirmation of MLH1/PMS2 DNA methylation | 26 | 9 | NR | NR | NR | NR | NR |
Djordjevic et al. (2013) [24] | 186 | 24.731 | USA | IHC detection of MLH1, MSH2, MSH6, PMS2 proteins, PCR confirmation of MLH1 methylation to confirm loss of MLH1 protein | 28 | 8 | NR | NR | NR | NR | NR |
Okoye et al. (2016) [25] | 411 | 27.981 | USA | IHC to detect loss of MLH1, MSH2, MSH6, PMS2, PCR confirmation of MLH1 methylation | NR | NR | NR | NR | NR | NR | NR |
Ruiz et al. (2014) [26] | 212 | 30.189 | Spain | IHC of MLH1, MSH2, MSH6, PMS2 markers | 58 | 16 | 50 | 10 | 50 | 14 | 6 |
Shikama et al. (2016) [27] | 221 | 28.054 | Japan | IHC of MMR proteins MLH1, MSH2, MSH6, PMS2 | NR | NR | 39 | 18 | NR | NR | 27 |
PCR confirmation of MLH1 methylation | |||||||||||
Stelloo et al. (2015) [28] | 116 | 16.379 | Europe | IHC of MLH1, MSH2, MSH6, PMS2, PCR MLH1 methylation confirmation | NR | NR | NR | NR | NR | NR | NR |
Promega (ver. 1.2) PCR testing of MSI markers | |||||||||||
Talhouk et al. (2016) [29] | 57 | 31.579 | Canada, USA | IHC of MMR proteins MLH1, MSH2, MSH6, PMS2 | 10 | 6 | 9 | 3 | 6 | 5 | 7 |
Talhouk et al. (2017) [30] | 319 | 9.718 | Canada | IHC for presence or absence MMR proteins MLH1, MSH2, MSH6, PMS2 | NR | NR | NR | NR | NR | NR | 28 |