Abstract
Background
Methods
Results
Supplementary Material
Supplemental Table S1.
Supplemental Table S2.
Supplemental Table S3.
Supplemental Fig. S1.
Supplemental Fig. S2.
Supplemental Fig. S3.
Supplemental Fig. S4.
ACKNOWLEDGMENTS
REFERENCES


















Fig. 1.
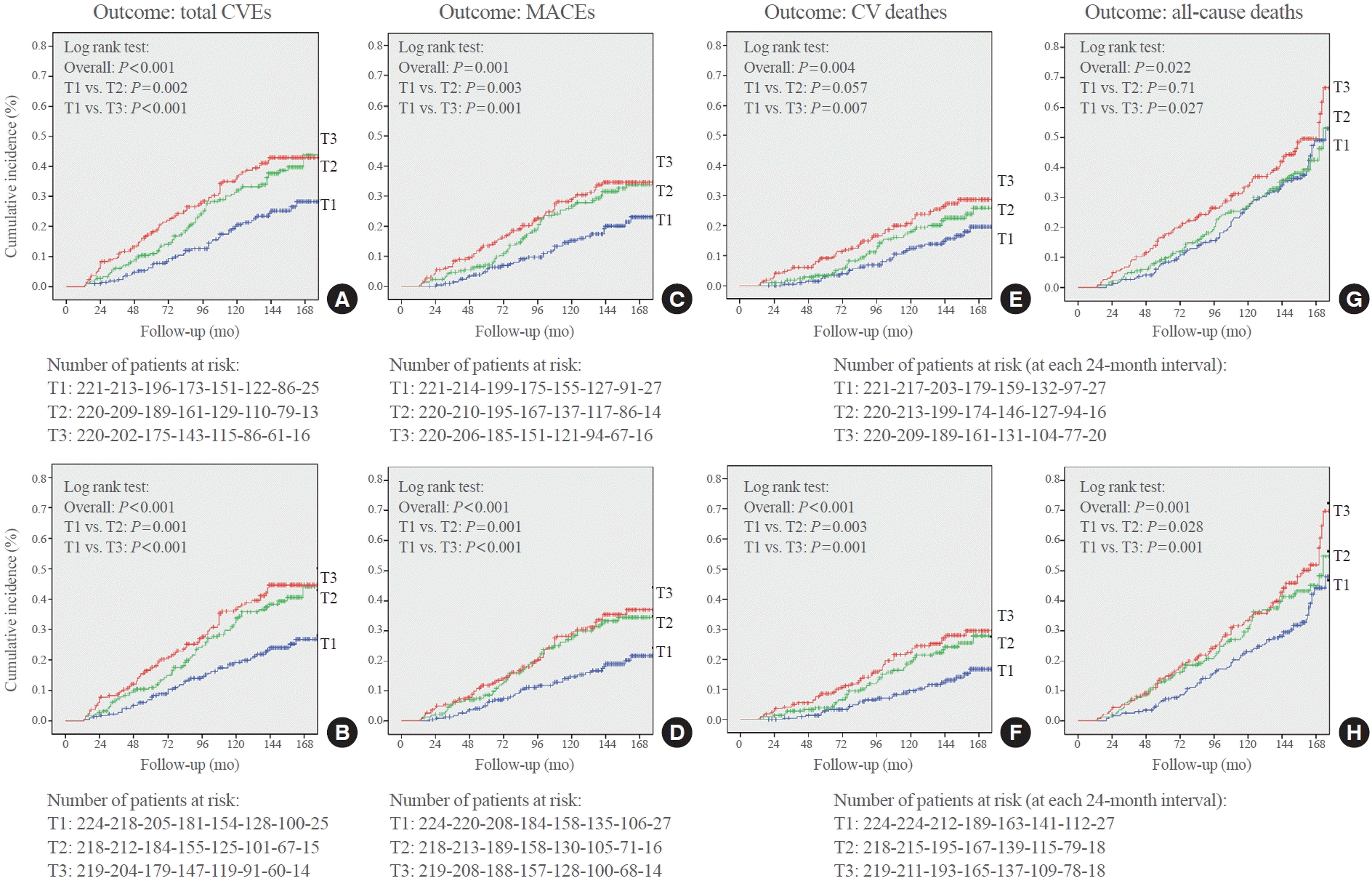
Fig. 2.
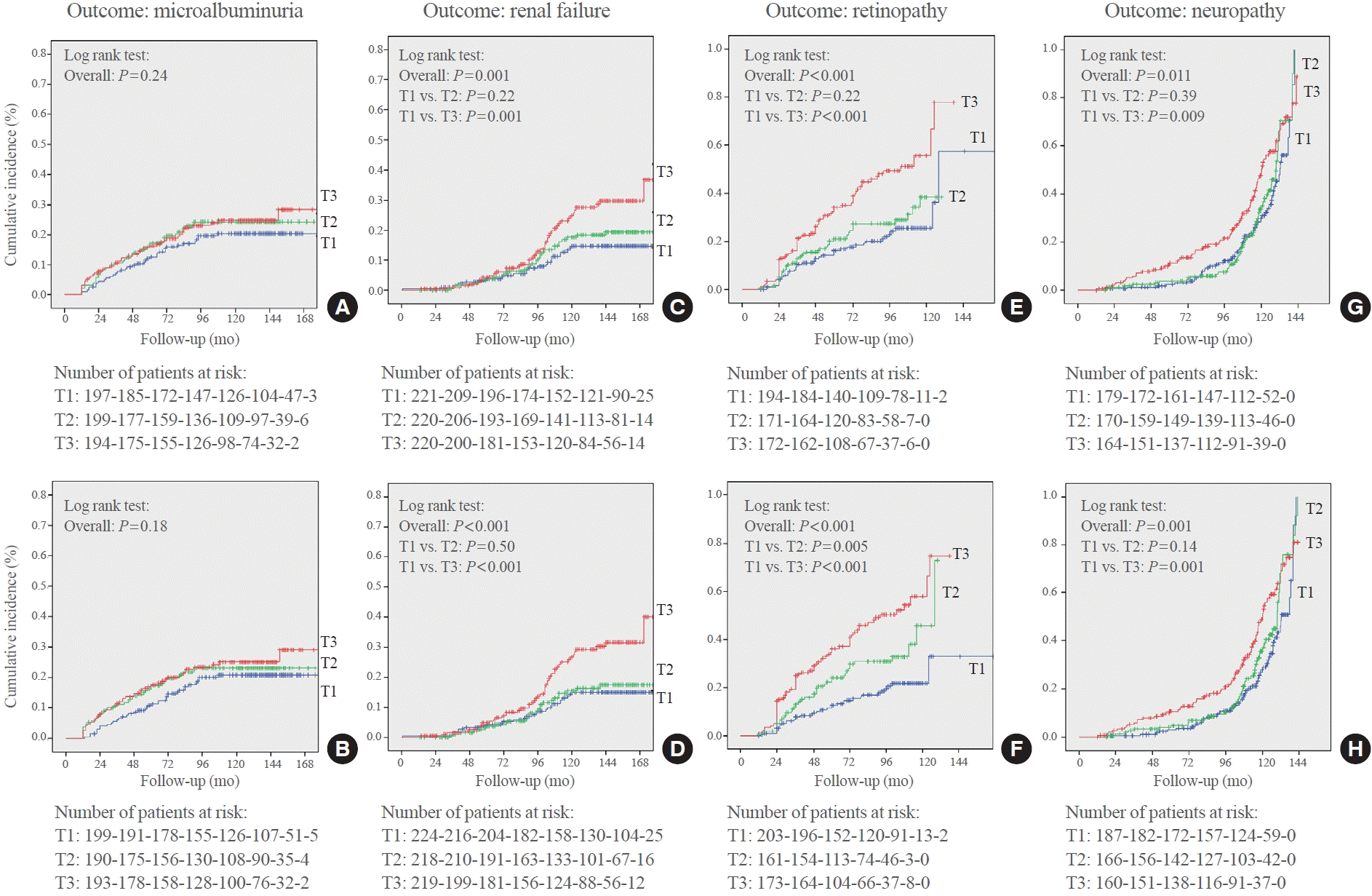
Table 1.
Characteristic | All patients (n=687) | Lower tertile mean HGI <–0.7% (n=229) | Middle tertile mean HGI –0.7% to 0.2% (n=229) | Upper tertile mean HGI >0.2% (n=229) | P value |
---|---|---|---|---|---|
Age, yr | 60.1±9.5 | 61.2±10.0 | 60.6±9.6 | 58.7±8.8b | 0.014 |
Male sex, % | 38.4 | 45.5 | 37.3 | 32.3b | 0.015 |
Body mass index, kg/m2 | 29.7±4.9 | 29.6±4.7 | 29.9±5.1 | 29.6±5.0 | 0.735 |
Abdominal circumference, cm | 102±11 | 103±11 | 103±11 | 101±12 | 0.194 |
Smoking, current/past, % | 44.9 | 46.0 | 44.9 | 43.9 | 0.910 |
Physical activity, % active | 21.9 | 23.2 | 24.0 | 18.4 | 0.298 |
Diabetes duration, yr | 8 (3–15) | 5 (1–11) | 8 (3–15)b | 10 (6–18)b | <0.001 |
Chronic diabetic complications, % | |||||
Cerebrovascular disease | 9.4 | 10.3 | 9.3 | 8.5 | 0.818 |
Coronary artery disease | 15.6 | 14.3 | 16.0 | 16.6 | 0.784 |
Peripheral artery disease | 17.3 | 12.9 | 18.8 | 20.3 | 0.097 |
Retinopathy | 32.5 | 20.5 | 31.3 | 45.5b | <0.001 |
Nephropathy | 32.1 | 23.3 | 27.5 | 45.7b | <0.001 |
Peripheral neuropathy | 29.2 | 26.7 | 29.5 | 31.4 | 0.555 |
Diabetes treatment, % | |||||
Metformin | 87.8 | 89.7 | 88.0 | 85.7 | 0.417 |
Sulfonylureas | 43.0 | 48.7 | 49.3 | 30.9b | <0.001 |
Insulin | 48.4 | 25.9 | 44.0b | 75.3b | <0.001 |
Other medicationsa | 5.3 | 5.1 | 5.5 | 5.3 | 0.975 |
Dyslipidemia, % | 87.2 | 86.2 | 86.2 | 89.2 | 0.538 |
Statin use | 77.4 | 75.4 | 76.0 | 80.7 | 0.343 |
Arterial hypertension, % | 86.7 | 83.9 | 88.4 | 87.8 | 0.310 |
No. of antihypertensive drugs | 3 (1–3) | 2 (1–3) | 3 (1–3) | 3 (2–3) | 0.053 |
SBP, mm Hg | 140±19 | 139±19 | 139±19 | 143±20 | 0.072 |
DBP, mm Hg | 79±10 | 79±10 | 78±10 | 80±11 | 0.057 |
Glycemic parameters | |||||
Baseline HbA1c, % | 8.0±1.9 | 7.0±1.7 | 7.8±1.4b | 9.3±1.8b | <0.001 |
Baseline FPG, mg/dL | 161±69 | 139±48 | 157±60 | 187±86 | <0.001 |
Baseline HGI, % | 0±1.6 | –0.6±1.4 | –0.2±1.4b | 1.0±1.6b | <0.001 |
Mean first-year HbA1c, % | 7.8±1.5 | 6.5±0.6 | 7.5±0.6b | 9.5±1.3b | <0.001 |
Mean first-year FPG, mg/dL | 147±47 | 132±36 | 144±45b | 163±53b | <0.001 |
Mean first-year HGI, % | 0±1.3 | –1.2±0.4 | –0.3±0.3b | 1.4±1.0b | <0.001 |
First-year HbA1c variability, % | 0.8±0.6 | 0.4±0.3 | 0.6±0.4 | 1.2±0.8b | <0.001 |
First-year HGI variability, % | 0.8±0.7 | 0.5±0.3 | 0.7±0.5 | 1.3±0.9b | <0.001 |
Other laboratory variables | |||||
Triacylglycerol, mg/dL | 169±136 | 168±136 | 169±160 | 170±106 | 0.976 |
Total cholesterol, mg/dL | 185±41 | 182±39 | 184±40 | 189±42 | 0.196 |
HDL-C, mg/dL | 44±12 | 44±11 | 43±11 | 44±12 | 0.823 |
LDL-C, mg/dL | 108±33 | 106±32 | 109±33 | 110±34 | 0.380 |
eGFR, mL/min/1.73 m2 | 81±20 | 80±19 | 81±21 | 81±22 | 0.827 |
Albuminuria, mg/day | 13 (7–41) | 11 (6–22) | 12 (7–33) | 21 (9–100)b | <0.001 |
Outcomes incidence, absolute number (incidence per 1,000 person-years) | |||||
Total cardiovascular events | 215 (35.3) | 52 (24.2) | 79 (39.4)b | 84 (46.3)b | <0.001 |
Major adverse cardiovascular events | 176 (27.9) | 40 (18.1) | 66 (31.6)b | 70 (37.0)b | 0.001 |
Cardiovascular mortality | 131 (19.9) | 33 (14.6) | 48 (22.1) | 50 (24.7)b | 0.004 |
All-cause mortality | 269 (40.8) | 87 (38.5) | 84 (38.6) | 98 (48.5)b | 0.022 |
Microalbuminuria (n=593) | 126 (25.3) | 38 (21.5) | 44 (27.1) | 44 (28.5) | 0.236 |
Advanced renal failure | 104 (16.9) | 25 (11.6) | 33 (16.0) | 46 (24.7)b | 0.001 |
Retinopathy (n=549) | 161 (50.7) | 40 (32.3) | 45 (44.4) | 76 (84.0)b | <0.001 |
Peripheral neuropathy (n=524) | 177 (42.4) | 53 (35.9) | 53 (37.9) | 71 (56.8)b | 0.011 |
Symptomatic hypoglycemia (n=496) | 90 (18.1) | 22 (13.3) | 31 (18.6) | 37 (23.9) | 0.050 |
Values are expressed as mean±standard deviation, percentage, median (interquartile range), or number (%).
HGI, hemoglobin glycation index; SBP, systolic blood pressure; DBP, diastolic blood pressure; HbA1c, glycated hemoglobin; FPG, fasting plasma glucose; HDL-C, high-density lipoprotein cholesterol; LDL-C, low-density lipoprotein cholesterol; eGFR, estimated glomerular filtration rate.
Table 2.
HGI and HbA1c parameter |
HR (95% CI) |
||
---|---|---|---|
Model 1d | Model 2d | Model 3d | |
Total cardiovascular events (n=215) | |||
Baseline HGI, continuouse | 1.25 (1.11–1.40)a | 1.13 (1.00–1.28)c | 1.13 (0.95–1.33) |
Baseline HbA1c, continuouse | 1.26 (1.11–1.42)a | 1.12 (0.98–1.28) | 1.16 (1.00–1.34) |
Baseline HGI, upper tertilef | 1.77 (1.26–2.48)a | 1.46 (1.02–2.10)c | 1.40 (0.84–2.32) |
Baseline HbA1c, upper tertilef | 1.76 (1.25–2.48)a | 1.37 (0.94–1.99) | 1.07 (0.63–1.82) |
Mean first-year HGI, continuouse | 1.43 (1.25–1.64)a | 1.23 (1.05–1.44)b | 1.36 (1.14–1.63)b |
Mean first-year HbA1c, continuouse | 1.55 (1.35–1.77)a | 1.31 (1.12–1.53)a | 1.28 (1.08–1.52)b |
Mean first-year HGI, upper tertilef | 2.51 (1.71–3.67)a | 1.75 (1.16–2.65)b | 1.48 (0.92–2.36) |
Mean first-year HbA1c, upper tertilef | 2.76 (1.90–4.03)a | 1.75 (1.14–2.70)c | 1.52 (0.78–2.97) |
First-year HGI variability, continuouse | 1.22 (1.09–1.36)a | 1.10 (0.97–1.25) | 1.11 (0.95–1.29) |
First-year HbA1c variability, continuouse | 1.18 (1.06–1.32)b | 1.11 (0.98–1.26) | 1.10 (0.95–1.27) |
First-year HGI variability, upper tertilef | 2.07 (1.45–2.94)a | 1.45 (1.00–2.12)c | 1.34 (0.81–2.22) |
First-year HbA1c variability, upper tertilef | 1.97 (1.38–2.81)a | 1.42 (0.98–2.06) | 1.13 (0.68–1.87) |
Major adverse cardiovascular events (n=176) | |||
Baseline HGI, continuouse | 1.22 (1.06–1.39)b | 1.12 (0.97–1.29) | 1.10 (0.91–1.33) |
Baseline HbA1c, continuouse | 1.22 (1.06–1.40)b | 1.10 (0.94–1.28) | 1.14 (0.96–1.35) |
Baseline HGI, upper tertilef | 1.81 (1.23–2.65)b | 1.51 (1.01–2.26)c | 1.51 (0.86–2.66) |
Baseline HbA1c, upper tertilef | 1.71 (1.16–2.52)b | 1.41 (0.96–2.09) | 1.19 (0.75–1.91) |
Mean first-year HGI, continuouse | 1.36 (1.17–1.59)a | 1.17 (0.98–1.40) | 1.28 (1.04–1.57)c |
Mean first-year HbA1c, continuouse | 1.45 (1.25–1.68)a | 1.24 (1.04–1.48)c | 1.21 (1.01–1.46)c |
Mean first-year HGI, upper tertilef | 2.70 (1.75–4.16)a | 1.90 (1.19–3.03)b | 1.42 (0.84–2.39) |
Mean first-year HbA1c, upper tertilef | 2.87 (1.88–4.40)a | 1.93 (1.20–3.12)b | 1.76 (0.83–3.74) |
First-year HGI variability, continuouse | 1.27 (1.13–1.43)a | 1.19 (1.04–1.36)b | 1.14 (0.97–1.34) |
First-year HbA1c variability, continuouse | 1.22 (1.09–1.38)a | 1.17 (1.02–1.34)c | 1.16 (0.99–1.35) |
First-year HGI variability, upper tertilef | 2.19 (1.48–3.24)a | 1.64 (1.08–2.49)c | 1.67 (0.94–2.97) |
First-year HbA1c variability, upper tertilef | 1.89 (1.28–2.78)a | 1.41 (0.94–2.12) | 0.97 (0.55–1.70) |
Cardiovascular mortality (n=131) | |||
Baseline HGI, continuouse | 1.22 (1.04–1.43)c | 1.13 (0.96–1.34) | 1.13 (0.91–1.41) |
Baseline HbA1c, continuouse | 1.21 (1.03–1.43)c | 1.12 (0.93–1.35) | 1.16 (0.95–1.42) |
Baseline HGI, upper tertilef | 1.79 (1.15–2.80)a | 1.57 (0.98–2.54) | 1.58 (0.81–3.08) |
Baseline HbA1c, upper tertilef | 1.59 (1.02–2.48)c | 1.38 (0.84–2.24) | 1.04 (0.60–1.79) |
Mean first-year HGI, continuouse | 1.41 (1.18–1.69)a | 1.25 (1.02–1.54)c | 1.39 (1.10–1.75)b |
Mean first-year HbA1c, continuouse | 1.55 (1.30–1.84)a | 1.35 (1.10–1.66)b | 1.28 (1.03–1.60)c |
Mean first-year HGI, upper tertilef | 2.69 (1.64–4.41)a | 1.98 (1.16–3.39)c | 1.24 (0.68–2.27) |
Mean first-year HbA1c, upper tertilef | 3.56 (2.15–5.91)a | 2.53 (1.44–4.47)a | 2.53 (1.05–6.06)c |
First-year HGI variability, continuouse | 1.26 (1.09–1.46)b | 1.14 (0.96–1.34) | 1.19 (0.98–1.44) |
First-year HbA1c variability, continuouse | 1.24 (1.07–1.43)b | 1.15 (0.98–1.36) | 1.17 (0.98–1.41) |
First-year HGI variability, upper tertilef | 2.20 (1.40–3.46)a | 1.55 (0.95–2.51) | 1.61 (0.84–3.10) |
First-year HbA1c variability, upper tertilef | 1.90 (1.21–2.96)b | 1.32 (0.82–2.11) | 0.92 (0.49–1.75) |
All-cause mortality (n=269) | |||
Baseline HGI, continuouse | 1.22 (1.09–1.37)a | 1.14 (1.01–1.28)c | 1.18 (1.01–1.38)c |
Baseline HbA1c, continuouse | 1.25 (1.11–1.40)a | 1.15 (1.01–1.31)c | 1.17 (1.02–1.35)c |
Baseline HGI, upper tertilef | 1.73 (1.28–2.35)a | 1.48 (1.06–2.05)c | 1.29 (0.82–2.03) |
Baseline HbA1c, upper tertilef | 1.72 (1.26–2.36)a | 1.44 (1.02–2.04)c | 1.26 (0.86–1.85) |
Mean first-year HGI, continuouse | 1.37 (1.20–1.55)a | 1.20 (1.03–1.38)c | 1.32 (1.12–1.55)b |
Mean first-year HbA1c, continuouse | 1.46 (1.29–1.66)a | 1.28 (1.11–1.48)a | 1.23 (1.05–1.43)b |
Mean first-year HGI, upper tertilef | 1.95 (1.41–2.70)a | 1.46 (1.03–2.08)c | 0.88 (0.49–1.57) |
Mean first-year HbA1c, upper tertilef | 2.50 (1.80–3.48)a | 1.78 (1.23–2.58)b | 1.98 (1.07–3.67)c |
First-year HGI variability, continuouse | 1.20 (1.07–1.33)a | 1.08 (0.96–1.23) | 1.12 (0.97–1.29) |
First-year HbA1c variability, continuouse | 1.18 (1.06–1.31)b | 1.09 (0.97–1.24) | 1.11 (0.96–1.27) |
First-year HGI variability, upper tertilef | 1.75 (1.28–2.39)a | 1.25 (0.90–1.76) | 1.18 (0.76–1.85) |
First-year HbA1c variability, upper tertilef | 1.66 (1.21–2.27)b | 1.23 (0.88–1.71) | 1.08 (0.69–1.69) |
New microalbuminuria development or progression to macroalbuminuria (n=126) | |||
Baseline HGI, continuouse | 1.08 (0.91–1.28) | 1.07 (0.89–1.29) | 1.01 (0.80–1.28) |
Baseline HbA1c, continuouse | 1.05 (0.88–1.25) | 1.01 (0.83–1.23) | 1.08 (0.86–1.35) |
Baseline HGI, upper tertilef | 0.86 (0.56–1.32) | 0.79 (0.49–1.26) | 0.80 (0.42–1.53) |
Baseline HbA1c, upper tertilef | 0.90 (0.58–1.41) | 0.83 (0.50–1.37) | 0.98 (0.50–1.96) |
Mean first-year HGI, continuouse | 1.19 (1.00–1.41) | 1.21 (0.99–1.47) | 1.30 (1.04–1.62)c |
Mean first-year HbA1c, continuouse | 1.24 (1.05–1.47)c | 1.26 (1.03–1.53)c | 1.24 (1.01–1.52)c |
Mean first-year HGI, upper tertilef | 1.28 (0.82–2.01) | 1.27 (0.77–2.11) | 0.90 (0.38–2.16) |
Mean first-year HbA1c, upper tertilef | 1.31 (0.84–2.04) | 1.36 (0.81–2.27) | 1.55 (0.63–3.78) |
First-year HGI variability, continuouse | 1.12 (0.96–1.30) | 1.12 (0.95–1.33) | 1.12 (0.92–1.35) |
First-year HbA1c variability, continuouse | 1.11 (0.95–1.29) | 1.11 (0.94–1.30) | 1.13 (0.93–1.38) |
First-year HGI variability, upper tertilef | 1.13 (0.74–1.72) | 1.10 (0.70–1.74) | 1.37 (0.72–2.61) |
First-year HbA1c variability, upper tertilef | 0.98 (0.64–1.48) | 0.87 (0.56–1.36) | 0.70 (0.38–1.32) |
Advanced renal failure development (n=104) | |||
Baseline HGI, continuouse | 1.42 (1.20–1.67)a | 1.28 (1.08–1.53)b | 1.35 (1.09–1.68)b |
Baseline HbA1c, continuouse | 1.47 (1.24–1.73)a | 1.30 (1.08–1.56)b | 1.35 (1.10–1.65)b |
Baseline HGI, upper tertilef | 2.48 (1.51–4.05)a | 2.05 (1.20–3.49)b | 1.72 (0.82–3.60) |
Baseline HbA1c, upper tertilef | 2.53 (1.53–4.20)a | 1.90 (1.08–3.34)c | 1.30 (0.59–2.84) |
Mean first-year HGI, continuouse | 1.55 (1.30–1.85)a | 1.36 (1.11–1.67)b | 1.44 (1.14–1.81)b |
Mean first-year HbA1c, continuouse | 1.61 (1.35–1.91)a | 1.36 (1.11–1.67)b | 1.40 (1.13–1.74)b |
Mean first-year HGI, upper tertilef | 2.53 (1.51–4.22)a | 1.65 (0.95–2.85) | 1.41 (0.61–3.26) |
Mean first-year HbA1c, upper tertilef | 2.69 (1.64–4.44)a | 1.47 (0.82–2.62) | 1.16 (0.48–2.84) |
First-year HGI variability, continuouse | 1.28 (1.11–1.48)a | 1.17 (0.99–1.40) | 1.27 (1.04–1.55)c |
First-year HbA1c variability, continuouse | 1.27 (1.11–1.45)a | 1.22 (1.03–1.44)c | 1.20 (0.99–1.46) |
First-year HGI variability, upper tertilef | 1.87 (1.14–3.05)c | 1.22 (0.72–2.07) | 0.91 (0.46–1.81) |
First-year HbA1c variability, upper tertilef | 2.29 (1.37–3.82)a | 1.51 (0.88–2.60) | 1.60 (0.79–3.24) |
Retinopathy development/progression (n=161) | |||
Baseline HGI, continuouse | 1.37 (1.20–1.57)a | 1.19 (1.02–1.37)c | 1.26 (1.05–1.52)c |
Baseline HbA1c, continuouse | 1.46 (1.28–1.67)a | 1.22 (1.04–1.42)c | 1.23 (1.03–1.46)c |
Baseline HGI, upper tertilef | 2.57 (1.73–3.82)a | 1.85 (1.19–2.86)b | 1.72 (0.91–3.25) |
Baseline HbA1c, upper tertilef | 2.61 (1.77–3.87)a | 1.62 (1.04–2.52)c | 1.09 (0.57–2.06) |
Mean first-year HGI, continuouse | 1.47 (1.29–1.67)a | 1.28 (1.09–1.51)b | 1.37 (1.15–1.63)a |
Mean first-year HbA1c, continuouse | 1.50 (1.33–1.70)a | 1.33 (1.14–1.55)a | 1.29 (1.09–1.53)b |
Mean first-year HGI, upper tertilef | 2.50 (1.67–3.73)a | 1.47 (0.94–2.31) | 0.65 (0.30–1.39) |
Mean first-year HbA1c, upper tertilef | 3.40 (2.25–5.15)a | 2.01 (1.25–3.22)b | 2.93 (1.30–6.59)b |
First-year HGI variability, continuouse | 1.39 (1.24–1.56)a | 1.28 (1.12–1.45)a | 1.26 (1.09–1.46)b |
First-year HbA1c variability, continuouse | 1.31 (1.17–1.48)a | 1.23 (1.09–1.40)b | 1.32 (1.14–1.53)a |
First-year HGI variability, upper tertilef | 2.85 (1.89–4.32)a | 1.86 (1.20–2.89)b | 1.37 (0.77–2.46) |
First-year HbA1c variability, upper tertilef | 2.81 (1.84–4.29)a | 2.13 (1.36–3.33)a | 1.62 (0.90–2.93) |
Peripheral neuropathy development/progression (n=177) | |||
Baseline HGI, continuouse | 1.31 (1.14–1.50)a | 1.28 (1.10–1.47)b | 1.48 (1.23–1.79)a |
Baseline HbA1c, continuouse | 1.46 (1.27–1.68)a | 1.39 (1.19–1.62)a | 1.38 (1.17–1.63)a |
Baseline HGI, upper tertilef | 1.82 (1.26–2.62)b | 1.64 (1.11–2.43)c | 1.21 (0.68–2.14) |
Baseline HbA1c, upper tertilef | 2.22 (1.53–3.20)a | 1.79 (1.19–2.70)b | 1.57 (0.86–2.86) |
Mean first-year HGI, continuouse | 1.41 (1.22–1.64)a | 1.29 (1.09–1.54)b | 1.33 (1.09–1.61)b |
Mean first-year HbA1c, continuouse | 1.42 (1.23–1.64)a | 1.29 (1.09–1.53)b | 1.31 (1.09–1.58)b |
Mean first-year HGI, upper tertilef | 1.90 (1.29–2.79)b | 1.36 (0.89–2.08) | 0.89 (0.45–1.81) |
Mean first-year HbA1c, upper tertilef | 2.31 (1.56–3.40)a | 1.59 (1.01–2.50)c | 1.78 (0.82–3.87) |
First-year HGI variability, continuouse | 1.20 (1.07–1.36)b | 1.12 (0.98–1.28) | 1.16 (0.99–1.37) |
First-year HbA1c variability, continuouse | 1.20 (1.07–1.36)b | 1.14 (1.00–1.31)c | 1.14 (0.98–1.33) |
First-year HGI variability, upper tertilef | 1.44 (0.98–2.12) | 1.04 (0.69–1.57) | 0.79 (0.47–1.35) |
First-year HbA1c variability, upper tertilef | 2.10 (1.44–3.06)a | 1.74 (1.15–2.62)b | 1.84 (1.18–2.87)b |
Symptomatic hypoglycemia (n=90) | |||
Baseline HGI, continuouse | 1.23 (0.99–1.53) | 1.04 (0.81–1.33) | 1.26 (0.93–1.72) |
Baseline HbA1c, continuouse | 1.45 (1.17–1.81)b | 1.21 (0.94–1.57) | 1.10 (0.82–1.48) |
Baseline HGI, upper tertilef | 1.37 (0.78–2.42) | 0.86 (0.46–1.61) | 0.80 (0.38–1.68) |
Baseline HbA1c, upper tertilef | 2.06 (1.16–3.67)c | 1.11 (0.57–2.15) | 1.59 (0.65–3.90) |
Mean first-year HGI, continuouse | 1.29 (1.03–1.61)c | 0.96 (0.74–1.25) | 1.00 (0.75–1.34) |
Mean first-year HbA1c, continuouse | 1.34 (1.07–1.67)c | 1.00 (0.77–1.29) | 0.97 (0.73–1.27) |
Mean first-year HGI, upper tertilef | 1.82 (1.01–3.30)c | 1.01 (0.53–1.93) | 0.69 (0.31–1.56) |
Mean first-year HbA1c, upper tertilef | 2.70 (1.45–5.03)b | 1.42 (0.72–2.80) | 2.50 (0.77–8.14) |
First-year HGI variability, continuouse | 1.24 (1.00–1.53) | 1.05 (0.83–1.33) | 0.97 (0.72–1.31) |
First-year HbA1c variability, continuouse | 1.13 (0.91–1.42) | 0.98 (0.76–1.27) | 1.05 (0.79–1.38) |
First-year HGI variability, upper tertilef | 2.48 (1.38–4.44)b | 1.59 (0.84–3.00) | 1.68 (0.74–3.83) |
First-year HbA1c variability, upper tertilef | 2.46 (1.32–4.59)b | 1.91 (0.99–3.69) | 1.88 (0.89–3.96) |
Values are expressed as adjusted HR and their respective 95% CI, except for hypoglycemia outcome, which were adjusted odds ratios.
HGI, hemoglobin glycation index; HbA1c, glycated hemoglobin; HR, hazard ratio; CI, confidence interval.
d Model 1 was adjusted for age and sex. Model 2 was further adjusted for body mass index (body height in neuropathy analyses), physical activity, smoking status, diabetes duration, pre-existent macrovascular and microvascular complications, systolic blood pressure, serum low-density lipoprotein cholesterol, use of insulin, aspirin and statins, and number of antihypertensive drugs in use. Model 3 was further simultaneously adjusted for HGI and HbA1c parameters. Analyses of renal outcomes were further adjusted for baseline albuminuria and estimated glomerular filtration rate;
Table 3.
HGI and HbA1c parameters | C-statisticsa, AUC (95% CI) |
---|---|
Total cardiovascular events (n=215) | |
Baseline HGI, continuous | 0.721 (0.679–0.762) |
Baseline HbA1c, continuous | 0.720 (0.678–0.761) |
Both baseline HGI and HbA1c, continuous | 0.721 (0.679–0.762) |
Baseline HGI, tertiles | 0.722 (0.680–0.763) |
Baseline HbA1c, tertiles | 0.720 (0.678–0.761) |
Both baseline HGI and HbA1c, tertiles | 0.722 (0.681–0.763) |
Mean 1-year HGI, continuous | 0.725 (0.683–0.766) |
Mean 1-year HbA1c, continuous | 0.727 (0.686–0.768) |
Both mean 1-year HGI and HbA1c, continuous | 0.728 (0.687–0.769) |
Mean 1-year HGI, tertiles | 0.731 (0.690–0.772) |
Mean 1-year HbA1c, tertiles | 0.728 (0.686–0.769) |
Both mean 1-year HGI and HbA1c, tertiles | 0.730 (0.689–0.771) |
1-year HGI variability, continuous | 0.719 (0.676–0.761) |
1-year HbA1c variability, continuous | 0.718 (0.676–0.760) |
Both 1-year HGI and HbA1c variabilities, continuous | 0.718 (0.676–0.761) |
1-year HGI variability, tertiles | 0.723 (0.680–0.765) |
1-year HbA1c variability, tertiles | 0.719 (0.677–0.762) |
Both 1-year HGI and HbA1c variabilities, tertiles | 0.722 (0.680–0.764) |
Major adverse cardiovascular events (n=176) | |
Baseline HGI, continuous | 0.716 (0.671–0.760) |
Baseline HbA1c, continuous | 0.716 (0.671–0.760) |
Both baseline HGI and HbA1c, continuous | 0.717 (0.673–0.761) |
Baseline HGI, tertiles | 0.719 (0.675–0.763) |
Baseline HbA1c, tertiles | 0.717 (0.673–0.762) |
Both baseline HGI and HbA1c, tertiles | 0.719 (0.674–0.763) |
Mean 1-year HGI, continuous | 0.720 (0.676–0.764) |
Mean 1-year HbA1c, continuous | 0.721 (0.677–0.765) |
Both mean 1-year HGI and HbA1c, continuous | 0.722 (0.678–0.767) |
Mean 1-year HGI, tertiles | 0.730 (0.686–0.774) |
Mean 1-year HbA1c, tertiles | 0.729 (0.685–0.773) |
Both mean 1-year HGI and HbA1c, tertiles | 0.730 (0.686–0.774) |
1-year HGI variability, continuous | 0.721 (0.676–0.766) |
1-year HbA1c variability, continuous | 0.719 (0.674–0.764) |
Both 1-year HGI and HbA1c variabilities, continuous | 0.721 (0.676–0.766) |
1-year HGI variability, tertiles | 0.726 (0.682–0.771) |
1-year HbA1c variability, tertiles | 0.720 (0.675–0.764) |
Both 1-year HGI and HbA1c variabilities, tertiles | 0.726 (0.682–0.771) |
Cardiovascular mortality (n=131) | |
Baseline HGI, continuous | 0.720 (0.672–0.767) |
Baseline HbA1c, continuous | 0.721 (0.673–0.768) |
Both baseline HGI and HbA1c, continuous | 0.722 (0.675–0.768) |
Baseline HGI, tertiles | 0.722 (0.674–0.769) |
Baseline HbA1c, tertiles | 0.721 (0.674–0.768) |
Both baseline HGI and HbA1c, tertiles | 0.724 (0.677–0.771) |
Mean 1-year HGI, continuous | 0.722 (0.674–0.769) |
Mean 1-year HbA1c, continuous | 0.723 (0.675–0.771) |
Both mean 1-year HGI and HbA1c, continuous | 0.724 (0.676–0.772) |
Mean 1-year HGI, tertiles | 0.725 (0.677–0.773) |
Mean 1-year HbA1c, tertiles | 0.732 (0.684–0.780) |
Both mean 1-year HGI and HbA1c, tertiles | 0.733 (0.686–0.781) |
1-year HGI variability, continuous | 0.717 (0.668–0.767) |
1-year HbA1c variability, continuous | 0.717 (0.668–0.766) |
Both 1-year HGI and HbA1c variabilities, continuous | 0.717 (0.668–0.767) |
1-year HGI variability, tertiles | 0.724 (0.675–0.773) |
1-year HbA1c variability, tertiles | 0.720 (0.671–0.768) |
Both 1-year HGI and HbA1c variabilities, tertiles | 0.724 (0.675–0.773) |
All-cause mortality (n=269) | |
Baseline HGI, continuous | 0.750 (0.712–0.788) |
Baseline HbA1c, continuous | 0.750 (0.712–0.788) |
Both baseline HGI and HbA1c, continuous | 0.750 (0.713–0.788) |
Baseline HGI, tertiles | 0.750 (0.712–0.788) |
Baseline HbA1c, tertiles | 0.750 (0.713–0.788) |
Both baseline HGI and HbA1c, tertiles | 0.750 (0.712–0.788) |
Mean 1-year HGI, continuous | 0.750 (0.711–0.788) |
Mean 1-year HbA1c, continuous | 0.750 (0.712–0.788) |
Both mean 1-year HGI and HbA1c, continuous | 0.754 (0.716–0.792) |
Mean 1-year HGI, tertiles | 0.751 (0.713–0.789) |
Mean 1-year HbA1c, tertiles | 0.751 (0.713–0.789) |
Both mean 1-year HGI and HbA1c, tertiles | 0.755 (0.717–0.793) |
1-year HGI variability, continuous | 0.750 (0.711–0.788) |
1-year HbA1c variability, continuous | 0.749 (0.710–0.787) |
Both 1-year HGI and HbA1c variabilities, continuous | 0.749 (0.711–0.788) |
1-year HGI variability, tertiles | 0.752 (0.713–0.790) |
1-year HbA1c variability, tertiles | 0.748 (0.710–0.787) |
Both 1-year HGI and HbA1c variabilities, tertiles | 0.752 (0.713–0.790) |
New microalbuminuria development or progression to macroalbuminuria (n=126) | |
Baseline HGI, continuous | 0.663 (0.611–0.715) |
Baseline HbA1c, continuous | 0.664 (0.612–0.716) |
Both baseline HGI and HbA1c, continuous | 0.666 (0.614–0.718) |
Baseline HGI, tertiles | 0.667 (0.614–0.719) |
Baseline HbA1c, tertiles | 0.664 (0.612–0.715) |
Both baseline HGI and HbA1c, tertiles | 0.666 (0.614–0.717) |
Mean 1-year HGI, continuous | 0.667 (0.614–0.720) |
Mean 1-year HbA1c, continuous | 0.666 (0.613–0.719) |
Both mean 1-year HGI and HbA1c, continuous | 0.670 (0.616–0.723) |
Mean 1-year HGI, tertiles | 0.666 (0.613–0.718) |
Mean 1-year HbA1c, tertiles | 0.661 (0.609–0.714) |
Both mean 1-year HGI and HbA1c, tertiles | 0.663 (0.610–0.715) |
1-year HGI variability, continuous | 0.661 (0.609–0.713) |
1-year HbA1c variability, continuous | 0.659 (0.606–0.711) |
Both 1-year HGI and HbA1c variabilities, continuous | 0.661 (0.609–0.713) |
1-year HGI variability, tertiles | 0.660 (0.607–0.712) |
1-year HbA1c variability, tertiles | 0.668 (0.616–0.721) |
Both 1-year HGI and HbA1c variabilities, tertiles | 0.668 (0.614–0.721) |
Advanced renal failure development (n=104) | |
Baseline HGI, continuous | 0.712 (0.658–0.767) |
Baseline HbA1c, continuous | 0.712 (0.657–0.766) |
Both baseline HGI and HbA1c, continuous | 0.713 (0.658–0.768) |
Baseline HGI, tertiles | 0.712 (0.658–0.767) |
Baseline HbA1c, tertiles | 0.711 (0.657–0.766) |
Both baseline HGI and HbA1c, tertiles | 0.713 (0.659–0.767) |
Mean 1-year HGI, continuous | 0.715 (0.658–0.772) |
Mean 1-year HbA1c, continuous | 0.716 (0.660–0.772) |
Both mean 1-year HGI and HbA1c, continuous | 0.715 (0.659–0.771) |
Mean 1-year HGI, tertiles | 0.712 (0.655–0.768) |
Mean 1-year HbA1c, tertiles | 0.712 (0.655–0.769) |
Both mean 1-year HGI and HbA1c, tertiles | 0.712 (0.655–0.769) |
1-year HGI variability, continuous | 0.713 (0.657–0.770) |
1-year HbA1c variability, continuous | 0.715 (0.658–0.772) |
Both 1-year HGI and HbA1c variabilities, continuous | 0.715 (0.657–0.772) |
1-year HGI variability, tertiles | 0.711 (0.656–0.767) |
1-year HbA1c variability, tertiles | 0.714 (0.657–0.770) |
Both 1-year HGI and HbA1c variabilities, tertiles | 0.713 (0.657–0.769) |
Retinopathy development/progression (n=161) | |
Baseline HGI, continuous | 0.740 (0.694–0.786) |
Baseline HbA1c, continuous | 0.742 (0.696–0.787) |
Both baseline HGI and HbA1c, continuous | 0.742 (0.696–0.787) |
Baseline HGI, tertiles | 0.743 (0.697–0.788) |
Baseline HbA1c, tertiles | 0.742 (0.697–0.787) |
Both baseline HGI and HbA1c, tertiles | 0.746 (0.701–0.791) |
Mean 1-year HGI, continuous | 0.747 (0.701–0.793) |
Mean 1-year HbA1c, continuous | 0.756 (0.711–0.801) |
Both mean 1-year HGI and HbA1c, continuous | 0.760 (0.716–0.804) |
Mean 1-year HGI, tertiles | 0.744 (0.697–0.790) |
Mean 1-year HbA1c, tertiles | 0.749 (0.703–0.794) |
Both mean 1-year HGI and HbA1c, tertiles | 0.754 (0.708–0.799) |
1-year HGI variability, continuous | 0.753 (0.707–0.798) |
1-year HbA1c variability, continuous | 0.745 (0.699–0.791) |
Both 1-year HGI and HbA1c variabilities, continuous | 0.752 (0.706–0.797) |
1-year HGI variability, tertiles | 0.755 (0.710–0.799) |
1-year HbA1c variability, tertiles | 0.748 (0.703–0.793) |
Both 1-year HGI and HbA1c variabilities, tertiles | 0.755 (0.712–0.799) |
Peripheral neuropathy development/progression (n=177) | |
Baseline HGI, continuous | 0.674 (0.625–0.723) |
Baseline HbA1c, continuous | 0.679 (0.631–0.728) |
Both baseline HGI and HbA1c, continuous | 0.681 (0.633–0.730) |
Baseline HGI, tertiles | 0.679 (0.631–0.728) |
Baseline HbA1c, tertiles | 0.683 (0.635–0.732) |
Both baseline HGI and HbA1c, tertiles | 0.685 (0.636–0.733) |
Mean 1-year HGI, continuous | 0.686 (0.638–0.735) |
Mean 1-year HbA1c, continuous | 0.687 (0.639–0.735) |
Both mean 1-year HGI and HbA1c, continuous | 0.688 (0.639–0.736) |
Mean 1-year HGI, tertiles | 0.681 (0.633–0.730) |
Mean 1-year HbA1c, tertiles | 0.682 (0.634–0.731) |
Both mean 1-year HGI and HbA1c, tertiles | 0.683 (0.634–0.731) |
1-year HGI variability, continuous | 0.682 (0.633–0.730) |
1-year HbA1c variability, continuous | 0.680 (0.631–0.729) |
Both 1-year HGI and HbA1c variabilities, continuous | 0.682 (0.633–0.730) |
1-year HGI variability, tertiles | 0.676 (0.627–0.725) |
1-year HbA1c variability, tertiles | 0.683 (0.635–0.732) |
Both 1-year HGI and HbA1c variabilities, tertiles | 0.683 (0.634–0.731) |
Symptomatic hypoglycemia (n=90) | |
Baseline HGI, continuous | 0.721 (0.668–0.775) |
Baseline HbA1c, continuous | 0.730 (0.677–0.784) |
Both baseline HGI and HbA1c, continuous | 0.731 (0.677–0.784) |
Baseline HGI, tertiles | 0.723 (0.670–0.776) |
Baseline HbA1c, tertiles | 0.725 (0.672–0.779) |
Both baseline HGI and HbA1c, tertiles | 0.730 (0.678–0.783) |
Mean 1-year HGI, continuous | 0.720 (0.666–0.773) |
Mean 1-year HbA1c, continuous | 0.720 (0.666–0.774) |
Both mean 1-year HGI and HbA1c, continuous | 0.721 (0.667–0.774) |
Mean 1-year HGI, tertiles | 0.720 (0.667–0.774) |
Mean 1-year HbA1c, tertiles | 0.723 (0.669–0.778) |
Both mean 1-year HGI and HbA1c, tertiles | 0.723 (0.669–0.778) |
1-year HGI variability, continuous | 0.722 (0.668–0.776) |
1-year HbA1c variability, continuous | 0.721 (0.667–0.775) |
Both 1-year HGI and HbA1c variabilities, continuous | 0.724 (0.671–0.777) |
1-year HGI variability, tertiles | 0.728 (0.676–0.781) |
1-year HbA1c variability, tertiles | 0.728 (0.675–0.781) |
Both 1-year HGI and HbA1c variabilities, tertiles | 0.732 (0.681–0.783) |