Abstract
Background
Methods
Results
Conclusions
ACKNOWLEDGEMENTS
Notes
AUTHOR CONTRIBUTIONS
Lee J was instrumental in conceptualizing the methodologies employed in the research and took a leading role in drafting and revising the manuscript, ensuring a comprehensive presentation of the ideas and findings. Choi JH was responsible for the provision and execution of the Quadrupole Time-of-Flight (qTOF) method, adding a crucial dimension to the multifaceted methodological approach adopted in the study. Kim EH and Im JH executed the vital task of sample preparation for the MALDI-TOF method. Yang SJ contributed to optimizing all the methods explored in this research. Hwang HY contributed ideas and assistance throughout the developmental phases of the research using MS. Lee JH, Lee KH, and Song JH offered insights and counsel on the methodologies and contributed to identifying the clinical implications of detecting M-protein using MS. Park SM shared expertise and provided counsel on the effective utilization of sample preparation methods, a contribution that helped fine-tune the method to achieve optimal results. Song SH, as the chief and main supervisor, held responsibility for the research, guiding the project to completion through careful supervision and ensuring high scientific standards.
Appendix
SUPPLEMENTARY MATERIALS
References
Fig. 1
Negative results found with IFE (A) and NB-MALDI-TOF (B). The m/z ranges of single- and double-charged light chains are presented in the red boxes. The X-axis represents the mass-to-charge ratio (m/z), and the Y-axis shows the summed signal intensity for 500 shots using MALDI-TOF.
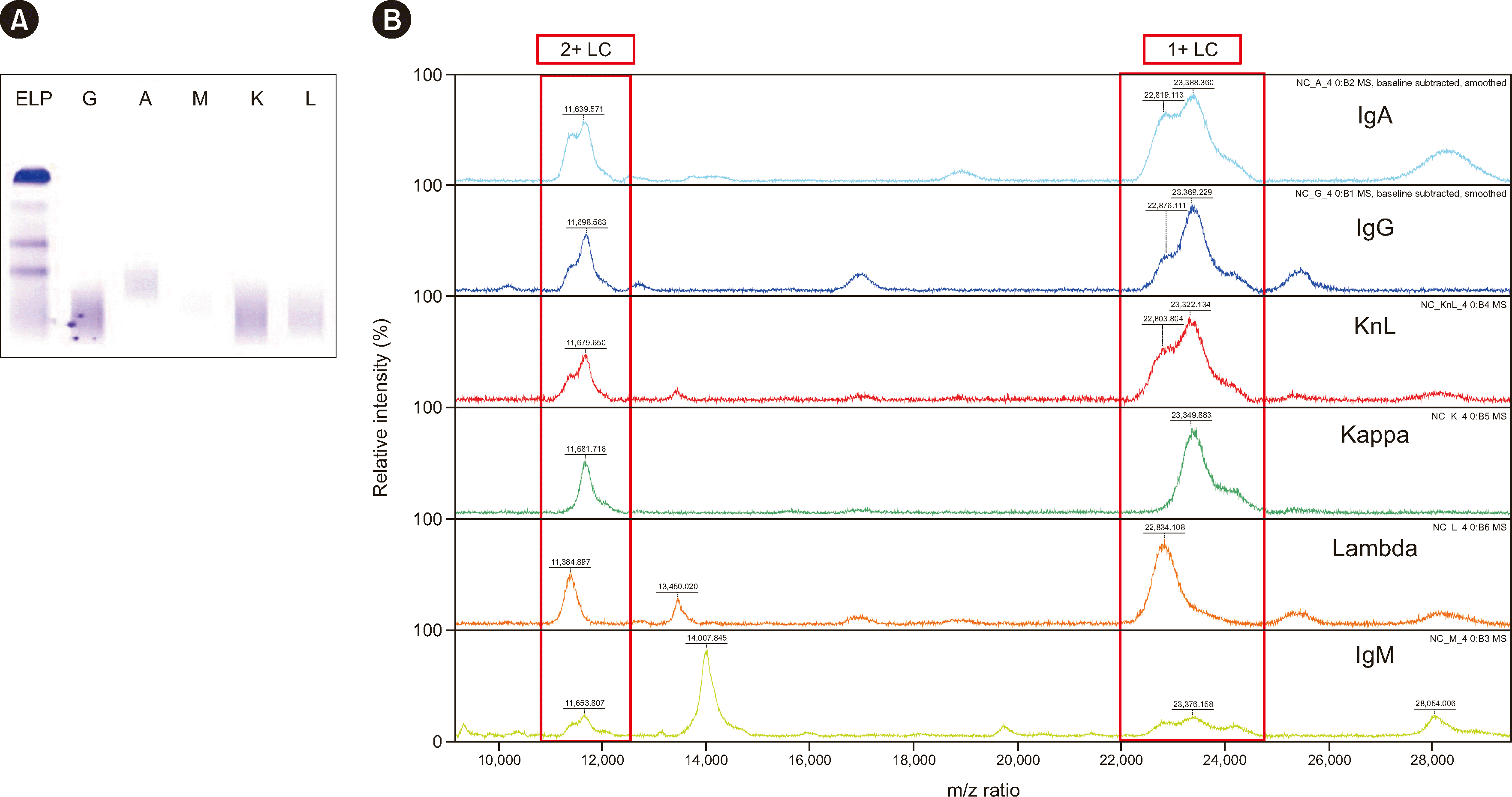
Fig. 2
Examples of NB-MALDI-TOF spectra found with normal and abnormal samples. (A) Polyclonal peaks observed with IgG beads. (B) Polyclonal peaks observed with mixed kappa and lambda. (C) Monoclonal peaks observed with IgG beads. (D) Monoclonal peaks observed with an abnormal IFE sample identified as having the IgG/kappa subtype.
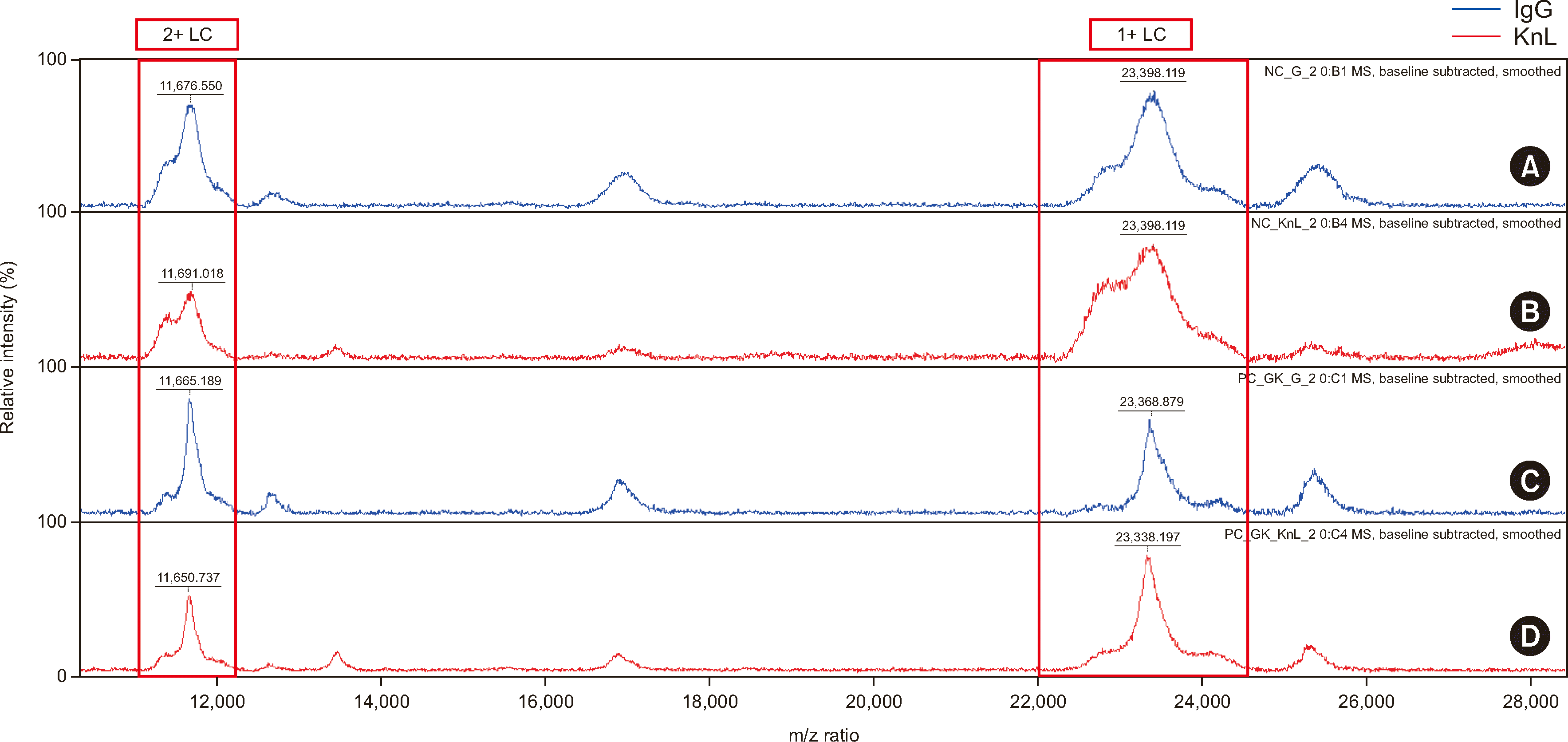
Fig. 3
Evaluation of limit of detection of NB-MALDI-TOF method following IgG purification using normal serum sample (A) and samples spiked with daratumumab at concentrations of 0.05 (B), 0.1 (C), 0.2 (D), 0.5 (E), or 1.0 g/dL (F).
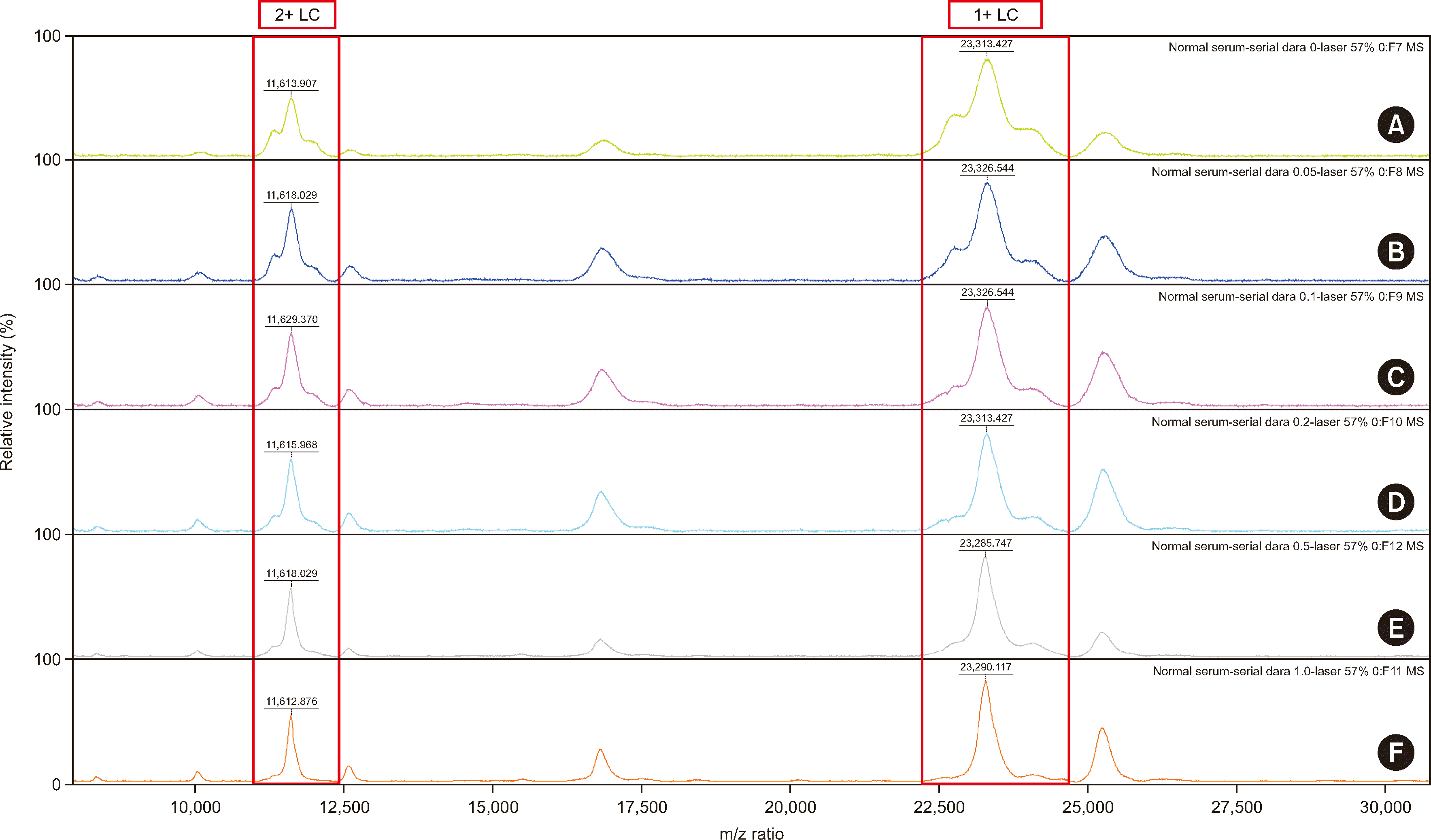
Fig. 4
NB-LC-ESI-qTOF results after purification with IgG, IgA, IgM, mixed kappa and lambda, kappa, and lambda beads. Mass spectra of samples after NB purification with IgG (A), IgA (B), IgM (C), KnL (D), kappa (E), and lambda (F) beads. Normal pooled serum spiked with daratumumab (0.1 g/dL) showed positive results with monoclonal peaks in the mass spectra after purification with IgG, KnL, and kappa beads (A, D, E) and negative results after purification with IgA, IgM, and lambda beads (B, C, F). The X-axis represents the mass values after deconvolution, and the Y-axis shows the relative intensity observed using NB-LC-ESI-qTOF. The intensity is annotated in the top right corner of each spectrum at 100% relative intensity.
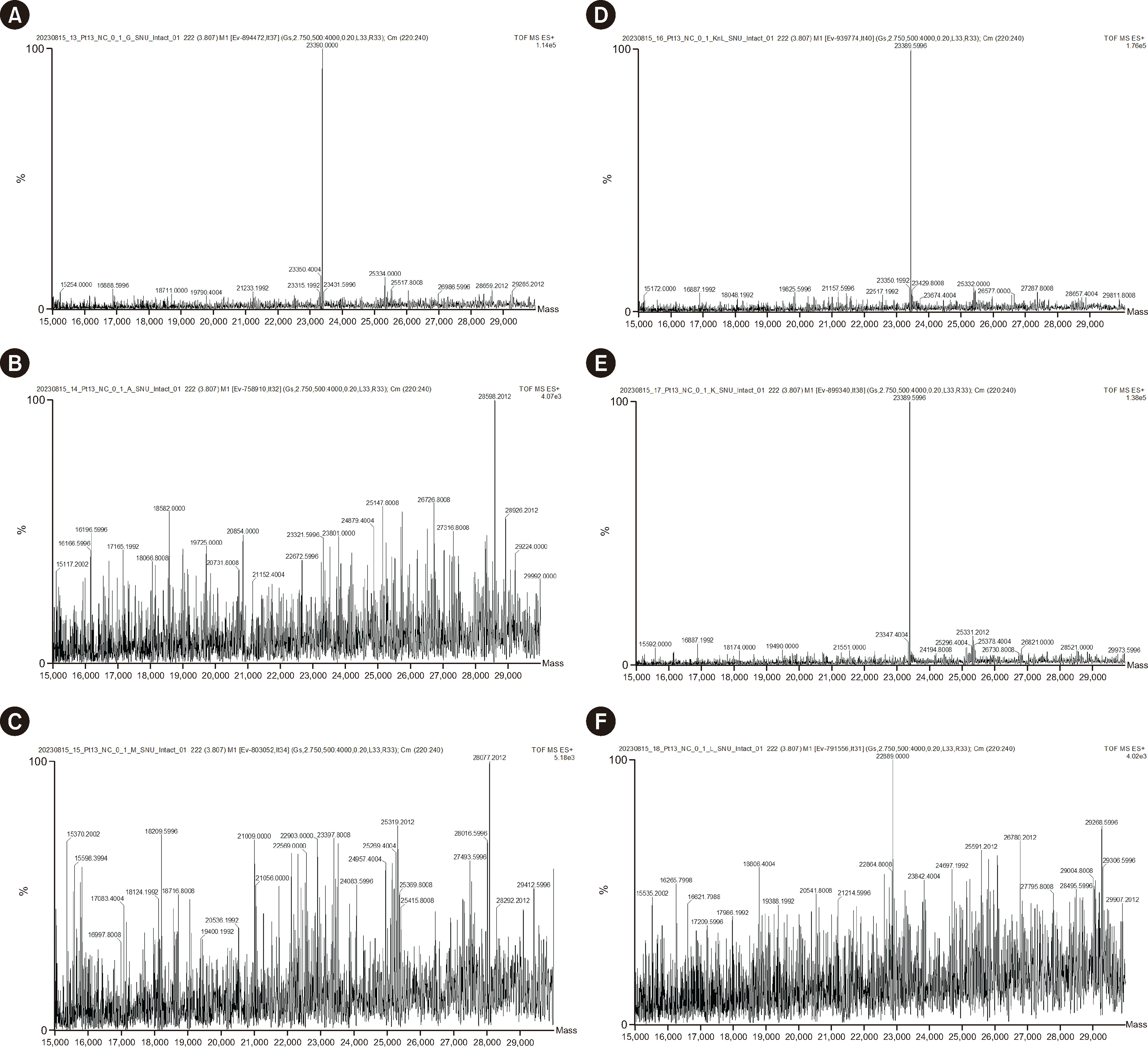
Table 1
Results obtained with normal serum samples via IFE versus those obtained with NB-MALDI-TOF and PEP
Table 2
Comparison of abnormal samples from IFE with results from NB-MALDI-TOF, PEP, and FLC assay.
No. case | IFE | NB-MALDI-TOF | PEP | M-protein, g/dL | M-protein, % |
K/L ratio (0.26–1.65)* |
Kappa, mg/L (3.3–19.4)* |
Lambda, mg/L (5.71–26.30)* |
---|---|---|---|---|---|---|---|---|
1 | IgG/kappa | IgG/kappa | Positive | 0.03 | 0.5 | N/A | <0.54 | <1.40 |
2 | IgG/kappa | IgG/kappa | Positive | 1.14 | 14.2 | 4.92 | 452.53 | 92.06 |
3 | IgG/kappa | IgG/kappa | Positive | 0.30 | 5.2 | 1.12 | 21.19 | 19.00 |
4 | IgG/kappa | IgG/kappa | Positive | 0.26 | 4.3 | 1.10 | 18.25 | 16.52 |
5 | IgG/kappa | IgG/kappa | Positive | 0.08 | 1.3 | 1.57 | 4.53 | 2.88 |
6 | IgG/kappa | Negative | Positive | 0.17 | 2.5 | 1.56 | 25.39 | 16.25 |
7 | IgG/kappa | IgG/kappa | Positive | 0.88 | 12.4 | 2.21 | 47.47 | 21.52 |
8 | s/o IgG/kappa | Negative | Positive | 0.18 | 2.9 | 34.16 | 496.39 | 14.53 |
9 | s/o IgG/lambda | IgG/lambda | Positive | 0.60 | 7.2 | 1.28 | 40.52 | 31.60 |
10 | IgG/lambda | IgG/lambda | Positive | 1.36 | 18.2 | 0.40 | 19.13 | 47.67 |
11 | IgG/lambda | IgG/lambda | Positive | 0.72 | 11.0 | 0.22 | 27.34 | 124.43 |
12 | IgG/lambda | IgG/lambda | Positive | 0.28 | 4.0 | 1.15 | 37.14 | 32.25 |
13 | IgG/lambda | IgG/lambda | Positive | 1.51 | 24.8 | 0.02 | 6.00 | 256.49 |
14 | IgG/lambda | IgG/lambda | Positive | 0.80 | 10.9 | 10.95 | 357.89 | 32.69 |
15 | IgA/kappa | IgA/kappa | Positive | 0.63 | 9.4 | 26.72 | 196.12 | 7.34 |
16 | IgA/kappa | IgA/kappa | Positive | 0.30 | 4.4 | 1.78 | 11.23 | 6.31 |
17 | IgA/lambda | IgA/lambda | Positive | 4.49 | 49.9 | 0.02 | 4.02 | 221.48 |
18 | IgA/lambda | IgA/lambda | Positive | 0.31 | 4.3 | 0.98 | 55.73 | 56.89 |
19 | IgA/lambda | IgA/lambda | Positive | 0.40 | 6.4 | 0.50 | 97.23 | 195.41 |
20 | IgM/kappa | IgM/kappa | Positive | 0.58 | 8.6 | 3.63 | 24.47 | 6.75 |
21 | IgM/kappa | IgM/kappa | Positive | 0.63 | 9.5 | 5.02 | 26.39 | 5.26 |
22 | s/o IgM/kappa | IgM/kappa | Negative | N/D | N/D | 1.41 | 21.24 | 15.05 |
23 | IgM/lambda | IgM/lambda | Negative | N/D | N/D | 2.09 | 54.93 | 26.27 |
24 | Lambda | Lambda | Positive | 0.08 | 1.7 | 0.01 | 24.69 | 2,050.12 |
25 | IgG/lambda +IgG/kappa (s/o daratumumab) | IgG/lambda +IgG/kappa (s/o daratumumab) | Positive | 0.38 | 5.9 | N/A | <0.54 | <1.40 |
Abbreviations: CHCA, α-cyano-4-hydroxycinnamic acid; DTT, dithiothreitol; DW, distilled water; FLC, free light chain; HC, heavy chain; IAA, iodoacetamide; PEP, protein electrophoresis; IFE, immunofixation electrophoresis; LC, light chain; LC-MS, liquid chromatography-mass spectrometry; LoD, limit of detection; MALDI-TOF, matrix-assisted laser desorption/ionization-time-of-flight; MB, magnetic beads; MM, multiple myeloma; M-protein, monoclonal protein; MRD, minimal residual disease; MS, mass spectrometry; N/A, not available; N/D, not detected; NB, nanobody affinity beads; NB-MALDI-TOF, nanobody affinity beads combined with matrix-assisted laser desorption/ionization-time-of-flight; PBS, phosphate-buffered saline; PCD, plasma cell disorder; PEP, protein electrophoresis; QE Orbitrap, Q-Exactive quadrupole Orbitrap; qTOF, quadrupole time-of-flight; s/o, suspected of; TCEP, tris-2-carboxyethl phosphine hydrochloride; t-mAb, therapy-related monoclonal antibody.