Abstract
Background and Objectives
We evaluated the effect of adipose-derived stem cell-derived conditioned medium (ADSC-CM) on the renal function of rats with renal ischemia-reperfusion injury (IRI)-induced acute kidney injury.
Methods and Results
Forty male Sprague‐Dawley rats were randomly divided into four groups: sham, nephrectomy control, IRI control, ADSC-CM. The ADSC-CM was prepared using the three-dimensional spheroid culture system and injected into renal parenchyme. The renal function of the rats was evaluated 28 days before and 1, 2, 3, 4, 7, and 14 days after surgical procedures. The rats were sacrificed 14 days after surgical procedures, and kidney tissues were collected for histological examination. The renal parenchymal injection of ADSC-CM significantly reduced the serum blood urea nitrogen and creatinine levels compared with the IRI control group on days 1, 2, 3, and 4 after IRI. The renal parenchymal injection of ADSC-CM significantly increased the level of creatinine clearance compared with the IRI control group 1 day after IRI. Collagen content was significantly lower in the ADSC-CM group than in the IRI control group in the cortex and medulla. Apoptosis was significantly decreased, and proliferation was significantly increased in the ADSC-CM group compared to the IRI control group in the cortex and medulla. The expressions of anti-oxidative makers were higher in the ADSC-CM group than in the IRI control group in the cortex and medulla.
Acute kidney injury (AKI) is an abrupt loss of kidney function that develops within 7 days (1). Renal ischemia-reperfusion injury (IRI) is a leading cause of perioperative AKI (2). In urologic practices, renal ischemia with reperfusion is induced during kidney transplants, partial nephrectomy, and nephrolithotomy with early renal dysfunction. Current treatments are primarily supportive to reduce the damage of renal IRI during these surgeries. AKI treatments are comprised of a series of supportive measures without an insult-oriented therapy. However, timely reperfusion of the ischemic area at risk is still the major concern in clinical practices, strategies such as ischemic preconditioning and controlled reperfusion along with antioxidant, complement, or neutrophil therapies may significantly prevent or limit IRI damage in humans (3).
Meta-analysis studies evaluating the therapeutic effect of mesenchymal stem cells (MSCs) in small animals with chronic or acute renal injury have shown the beneficial effect of MSC therapy on renal regeneration and functional recovery (4). In preclinical studies treating AKI, MSC therapy has shown to be beneficial through acting as an anti-inflammatory, antiapoptotic, anti-oxidative stress, antifibrotic, immunomodulatory, and proangiogenice. MSC treatment benefits have also been observed in clinical trials (5). There are some safety issues that need to be explored before MSC can be used in humans, including tumorigenicity (6), microbiological contamination (7), putative profibrogenic potential (8), and heterogeneity and functional diversity (9).
Adipose tissue-derived stromal vascular fraction (SVF) might be an attractive cell source for cell-based therapies on account of the real-time isolation without in vitro expansion to avoid the risks of MSC treatments. We found that renal function is effectively protected from renal IRI damage through the renal parenchymal injection of SVF (10). However, some issues limit the use of SVF in humans, including decreased cell viability during freezing and thawing, immune rejection when using allogeneic cells, and putative thrombogenic potential (11).
Conditioned medium (CM) therapy using soluble factors is emerging to avoid the various adverse effects of cell-based therapies, including MSC and SVF (12). Several preclinical studies have focused on using stem cell-derived CM in the treatment of various AKI models (13-15). Other preclinical studies have compared the effects of CM and cell-based therapies in AKI but have reported contradic-tory results (16-18). Therefore, we evaluated the effect of autologous adipose-derived stem cell (ADSC)-CM on the renal function in a rat model of renal IRI-induced AKI. We were interested in using the three-dimensional (3D) spheroid culture system to attain more growth factors and cytokines in the ADSC-CM. The ADSC in the 3D spheroid culture system secretes more angiogenic and anti-apoptotic factors compared to the conventional two-dimensional (2D) culture system (19).
All aspects of animal care, treatment, and surgical procedures followed the eighth edition of the Guide for the Care and Use of Laboratory Animals, published in 2011. All animal experiments were approved by the Institutional Animal Care and Use Committee of the Asan Medical Center (2020-12-037). Forty 8-week-old male Sprague-Dawley rats were purchased from Orient Bio Inc. (Seong-nam, Korea) and housed for 1 week for acclimatization. During experiments, rats were maintained under conditions identical to those used in our previous study (10).
Forty 9-week-old rats were randomly divided into four groups with ten animals per group: sham, nephrectomy control, IRI control, ADSC-CM. Rats in the ADSC-CM group underwent bilateral paratesticular fat excision via a scrotal incision to culture ADSC and acquire CM. At 13 weeks old, surgical procedures were performed to induce renal IRI. Vehicle (IRI control) and ADSC-CM were injected into the renal parenchyme. The renal function of all the rats was evaluated 28 days before and 1, 2, 3, 4, 7, and 14 days after surgical procedures. The rats were sacrificed 14 days after surgical procedures, and kidney tissues were then collected for histological examination.
ADSC was cultured as previously described using paratesticular fat harvested from each rat (20). ADSC was cultured in the conventional 2D culture system until passage number three.
Flow cytometry was performed with a FACScan argon laser cytometer (BD Biosciences, San Jose, CA) to determine whether the processed lipoaspirate cells were characteristic of ADSC according to a previous study (21). Briefly, cells were harvested in 0.25% trypsin/EDTA and fixed for 30 minutes in ice-cold 2% for-maldehyde. The fixed ADSCs were washed in flow cytometry buffer and incubated for 30 minutes in flow cytometry buffer containing anti-CD29Pacific Blue, CD44PE, CD45APC, and CD34FITC (BD Biosciences). Differentiation of ADSC was confirmed as described previously (20).
The ADSC-CM was prepared using ADSC spheroids as previously described (22). A 12-series micro-mold (The 3D Petri DishⓇ; Merck KGaA, Darmstadt, Germany) was used to prepare microwells following the manufacturer’s instructions. After the third passage, ADSCs were plated at 1×106 per well, and the microwells were incubated at 37℃ and 5% CO2 for 3 days to induce cell spheroids. After aspirating the media, 2 ml of serum-free DMEM were added, and ADSC-CM was collected after 48 hours of incubation. The collected ADSC-CM samples were concentrated using 3 kDa cut-off centrifugal filter units (Mer-ck KGaA) following the manufacturer’s instructions. The Bradford assay reagent (Sigma Aldrich) was used to measure protein concentration in the CM. The ADSC-CM was stored at −80℃ until use.
A laparotomy was performed in the sham group, and a right nephrectomy was performed in the nephrectomy control group. In the other two groups, a right nephrec-tomy was performed, and IRI of the left kidney was induced. IRI induction was performed in the left kidney as previously described (10, 23). ADSC-CM was injected via the renal parenchymal route based on the results of our previous study (10). A Hamilton syringe needle containing 100 μl of vehicle or ADSC-CM was pierced from the lower pole to the upper pole of the kidney. While pulling out the needle, the contents were gradually released from the syringe.
All rats were observed daily for mortality. Body weights were measured 28 days before and 1, 2, 3, 4, 7, and 14 days after surgical procedures. The food consumption was measured as previously described (10).
Serum blood urea nitrogen (BUN) and serum and urine creatinine levels were measured to evaluate the renal function. Urine was collected from the rats in a metabolic cage to estimate the 24-hour urine volume. The creatinine clearance (CrCl) was computed using the previously described formula (10).
The left kidney of all groups was harvested for histological examination. The weight of the left kidney was measured immediately after harvest. The kidney was cut in half, and the cross-section was visually observed. The renal index was computed using the previously described formula (10).
Kidney tissues were sectioned at 4 μm intervals, and hematoxylin & eosin staining was performed by standard methods. Histopathological scores were blindly estimated based on a previous study (24). Histopathological scores were quantitated by calculating the degree of interstitial fibrosis, interstitial inflammation, tubular dilation, tubular basophilia, intratubular eosinophilic granules, and glomerulus degeneration in ten randomly chosen non-overlapping fields.
Sirius red staining was performed as previously described (10, 23). Whole kidney slides of each rat were digitized with a Panoramic SCAN digital slide scanner (3D-HISTECH Ltd., Budapest, Hungary), and the histological images were subsequently analyzed by panoramic case viewer software (3DHISTECH Ltd.) at 20 times magni-fication. Four randomly selected kidney cortex and medulla fields from each rat were photographed using 3D-HISTECH’s CaseViewer. All images were analyzed using Adobe Photoshop to quantify signals. The results of Sirius red staining were presented as the percentage of collagen-positive areas in the total area of the cortex or medulla of each field.
Terminal deoxynucleotidyl transferase (TdT)-mediated dUTP nick end labeling (TUNEL) for the detection of apoptotic cells was performed using an in situ cell death detection kit according to the manufacturer’s protocol (Roche Molecular Biochemicals, Mannheim, Germany). The TUNEL assay measured the degree of apoptosis in tissues due to renal IRI and was used to clarify the effect of ADSC-CM. TUNEL staining was performed as previously described (25). After staining, the slides were analyzed like the Sirius red staining. The results of the TUNEL assay were presented as the percentage of TUNEL-positive areas in the cortex or medulla of each field.
Histological sections were labelled with anti-Ki67 (1:200, Abcam, Cambridge, MA), anti-glutathione reductase (GR; 1:2000, Abcam), or anti-glutathione peroxidase (GPx; 1:2000, Abcam) antibodies as described previously (10, 23). After staining, the slides were analyzed like the Sirius red staining. The immunohistochemistry results were presented as the percentage of antibody-positive areas in the cortex or medulla of each field.
Continuous variables are expressed as mean±standard error. We used a one-way analysis of variance followed by Tukey’s honest significant difference test for post hoc comparisons of multiple groups analyses. Statistical significance was defined as p<0.05, p<0.01, or p<0.001; p≥0.05 was not considered significant. All statistical analyses were performed using SPSS Statistics, version 21 (IBM Corporation, Armonk, NY).
To characterize ADSC, CD marker profiles of ADSC were examined using flow cytometry. ADSC flow cytometric analysis showed that 99.2% of the cells expressed CD29, 63.9% expressed CD44, 1.7% expressed CD45, and 3.1% expressed CD34. The multilineage differentiation capacity was confirmed by observation of adipogenic, osteogenic, and chondrogenic cells 3 weeks after culture in the appropriate induction mediums (Fig. 1).
One case in the ADSC-CM group experienced intraoperative death due to anesthesia (Supplementary Fig. S1A). The groups had no significant differences in body weight and food consumption (Supplementary Fig. S1B and S1C).
The renal parenchymal injection of ADSC-CM significantly reduced the levels of serum BUN compared with the IRI control group on days 1, 2, 3, and 4 after IRI (30.17 mg/dl vs. 53.96 mg/dl, p<0.001; 25.77 mg/dl vs. 49.43 mg/dl, p<0.001; 25.53 mg/dl vs. 38.44 mg/dl, p<0.001; 22.03 mg/dl vs. 28.53 mg/dl, p<0.01) (Fig. 2A). The renal parenchymal injection of ADSC-CM significantly reduced the levels of serum creatinine compared with the IRI control group on days 1, 2, 3, and 4 after IRI (0.85 mg/dl vs. 1.24 mg/dl, p<0.001; 0.78 mg/dl vs. 1.05 mg/dl, p<0.001; 0.77 mg/dl vs. 0.91 mg/dl, p<0.001; 0.70 mg/dl vs. 0.82 mg/dl, p<0.001) (Fig. 2B). The renal parenchymal injection of ADSC-CM significantly increased the level of CrCl compared with the IRI control group 1 day after IRI (0.22 ml/min/100 g vs. 0.16 ml/min/100 g, p<0.01) (Fig. 2C).
The renal index of the harvested kidneys was significantly increased in all the groups compared with the sham group (Supplementary Fig. S2A). No gross differences in kidney cross-sections were observed (Supplementary Fig. S2B).
Histopathological scores were significantly higher in the IRI control group than in the sham and nephrectomy control groups. There were no significant differences in histopathological scores between the IRI control and ADSC-CM groups (Fig. 3).
Sirius red staining revealed that collagen content was significantly higher in the IRI control group than in the sham and nephrectomy control groups in both cortex and medulla. Collagen content was significantly lower in the ADSC-CM group than in the IRI control group in both cortex and medulla (Fig. 4).
The TUNEL assay revealed a significant increase in apoptosis in the cortex and medulla in the IRI control group compared with the sham and nephrectomy control groups. Apoptosis in the cortex and medulla was significantly decreased in the ADSC-CM group compared with the IRI control group (Fig. 5).
Ki67 staining revealed a significant increase in proliferation in the cortex and medulla in the IRI control group compared with the sham and nephrectomy control groups. Proliferation in the cortex and medulla was significantly increased in the ADSC-CM groups compared with the IRI control group (Fig. 6).
The GR-positive areas in the cortex and medulla were significantly higher in the IRI control group than in the sham and nephrectomy control groups. The GR-positive staining was significantly higher in the ADSC-CM groups than in the IRI control group in the cortex and medulla (Fig. 7).
The GPx-positive areas in the cortex and medulla were significantly higher in the IRI control group than in the sham and nephrectomy control groups. The GPx-positive staining was significantly higher in the ADSC-CM groups than in the IRI control group in the cortex and medulla (Fig. 8).
Preclinical and clinical studies have investigated the effects of MSC in the treatment of AKI (4, 5). However, MSC treatment has several limitations, including tumorigenicity (6), microbiological contamination (7), putative profibrogenic potential (8), and heterogeneity and functional diversity (9). Preclinical studies using adipose tissue-derived SVF to treat AKI have increased because of the limitations in MSC treatments (10). However, SVF also has several limitations, including decreased cell viability during freezing and thawing, immune rejection when using allogeneic cells, and putative thrombogenic potential (11). In order to compensate for the shortcomings in cell-based therapies including MSC and SVF, CM therapy uses trophic factors and cytokines secreted from cells and has become increasingly attractive for the treatment of AKI (12). The CM which stem cells were cultured in contains many secretions called secretomes, microvesicles, or exosomes secreted from stem cells (26). Several preclinical studies have focused using stem cell-CM to treat AKI induced by cisplatin, renal IRI, and gentamicin (13-15). However, the studies comparing stem cells with their CM have questioned the effectiveness of stem cell-CM (16, 18).
Meanwhile, various methods, including culture conditions, drugs, and external stimuli, have been studied to attain more of the valuable secretions from stem cells (19, 26-31). One interesting method is stem cells cultured in hypoxic conditions and produces more angiogenic factors than in normoxic conditions (32-34). There are several methods to induce hypoxic culture conditions, including gas-controlled incubators, glove boxes, and biochemical induction of pseudo-hypoxic states (35). However, these methods require purchasing expensive equipment or treatment with drugs that must be removed later and cause cell damage. Several researchers have found that cells at the core of the spheroid are naturally exposed to mild hypoxia and limited nutrient diffusion (36). This method can induce hypoxic culture conditions cheaply and easily compared with other methods. The 3D Petri DishⓇ is a natural, scaffold-free 3D spheroid culture system to maximize cell-to-cell interactions. We found that human BMSC spheroids cultured using the 3D Petri DishⓇ showed a marked increase in cytokine secretion compared with the secretions in a 2D culture (22). This led to this study evaluating autologous ADSC-CM prepared using a 3D spheroid culture system and injecting via the renal parenchymal route to explore the effectiveness of treating renal IRI-induced AKI.
We found that renal parenchymal injections of ADSC-CM effectively restored renal function from renal IRI-induced AKI in terms of renal functions and histological examinations except histopathological scores. Tarng et al. (14) examined whether induced pluripotent stem cell (iPSC)-CM via intraperitoneal administration exhibited a systemic effect against renal IRI-induced AKI. They found that administration of iPSC-CM significantly improved renal function and survival rates in rats and protected tubular cells against apoptosis. The level of serum BUN and creatinine were significantly lower in iPSC-CM-treated rats than in normal control medium (NM)-treated rats 2 days after IRI. Similar to our study, no differences in serum BUN and creatinine levels were observed between the iPSC-CM- and NM-treated rats 7 days after IRI. Meanwhile, about 70% of PBS or NM-treated rats died within 7 days, whereas the mortality rate of iPSC-CM-treated rats was 30%. However, in our study, there was no mortality related to the AKI. The lack of mortality of our study might be attributed to our shorter clamping time of the renal artery than in their study (40 min vs. 45 min).
Similar reno-protective effects of stem cell-CM were also confirmed in other types of AKI models. Bi et al. (13) found that mice with intraperitoneal administration of bone marrow–derived MSC (BMSC)-CM or ADSC-CM limited the renal injury, increased survival, and diminished tubular cell apoptosis after cisplatin injection. The levels of serum BUN were markedly improved in both BMSC-CM and ADSC-CM-treated mice on days 3 and 5 after cisplatin injection. Six of the 15 mice in the cisplatin control group had died by day 6, whereas no mice died in either CM-treated group. Abedi et al. (15) found that intraperitoneal administration of human BMSC-CM in a gentamicin-induced AKI rat model showed relative improvement in some indicators of tissue injury. Although human BMSC-CM did not improve serum BUN and creatinine levels in all treatment groups, BMSC-CM injection one day before gentamicin injection diminished tissue da-mage. The authors assumed that the injection time of human BMSC-CM had a role in injury recovery. Similarly, our previous study found that injections 2 days before or concurrently with IRI had beneficial effects, whereas injections 2 days later did not have any benefit (23).
Meanwhile, some researchers have compared the effects of stem cells with their CM. Abouelkheir et al. (17) compared the efficacy of subcapsular injections of BMSC with intraperitoneal injections of BMSC-CM in a model of cisplatin-induced AKI. Both BMSC and BMSC-CM equally ameliorated kidney function deteriorations, tubular cell apoptosis, and reduced interstitial fibrosis after 2 months. However, adipocytes and osteoblast-like cells were detected at the BMSC injection site. The authors concluded that BMSC-CM might be an acceptable alternative to BMSC therapy as it attained comparable efficacy without the development of unwanted cells.
However, other researchers have questioned the effectiveness of CM. Abd El Zaher et al. (18) compared the effect of intravenous injections of BMSC versus BMSC-CM in minimizing cisplatin-induced AKI damage. The BMSC injection showed noticeable improvement of renal structures with a significant increase in Proliferating Cell Nuclear Antigen, but the BMSC-CM was less effective. Xing et al. (16) investigated the therapeutic potential of BMSC and BMSC-CM intravenously administered 1 day after IRI-induced AKI and found that BMSC injections improved renal function and histological alterations, leading to significantly reduced mortality. Although BMSC-CM contained proangiogenic factors, including hepatocyte growth factor, vascular endothelial growth factor-A, and insulin-like growth factor-1, beneficial effects were not detected.
Our study had some limitations. First, the exact composition of ADSC-CM was not investigated. Their mode of action was also not investigated. We found that human BMSC-CM prepared using a 3D spheroid culture system contained the highest concentrations of angiotrophic factors such as vascular endothelial growth factor and angiogenin (22). Second, the rats were followed for only 2 weeks. Abouelkheir et al. (17) reported that intraperitoneal injection of BMSC-CM reduced interstitial fibrosis 2 months after cisplatin-induced AKI. Third, the rats were only injected once with a fixed-dose applied concurrently with the induction of IRI. ADSC-CM is suitable for repeated or divided dosing because, unlike cell-based therapies, it can be frozen without significantly compromising the cell viability. Lastly, there are reports that female rats were more resistant to IRI than male rats, which raises questions about whether the results from male-only studies can be equally applied to females (37). Nevertheless, the findings from our study suggest that ADSC-CM prepared using a 3D spheroid culture system holds a promise as a safe therapeutic approach for the treatment of predictable injuries that might be induced by kidney surgeries and avoid the various adverse effects of cell-based therapy.
In conclusion, our study suggests that renal function is effectively rescued after IRI through renal parenchymal injections of ADSC-CM prepared using a 3D spheroid culture system.
Notes
Supplementary Materials
Supplementary data including two figures can be found with this article online at https://doi.org/10.15283/ijsc22137.
Author Contributions
Conception and design: Yu Seon Kim, Dalsan You.
Acquisition of data: Yu Seon Kim, Joomin Aum, Myong Jin Jang, Dalsan You.
Analysis and interpretation of data: Yu Seon Kim, Bo Hyun Kim, Dalsan You.
Drafting of the manuscript: Yu Seon Kim.
Critical revision of the manuscript: Jungyo Suh, Nayoung Suh, Dalsan You.
Supervision: Dalsan You.
References
1. Webb S, Dobb G. 2007; ARF, ATN or AKI? It's now acute kidney injury. Anaesth Intensive Care. 35:843–844. DOI: 10.1177/0310057X0703500601. PMID: 18084974.


2. Bonventre JV, Weinberg JM. 2003; Recent advances in the pathophysiology of ischemic acute renal failure. J Am Soc Nephrol. 14:2199–2210. DOI: 10.1097/01.ASN.0000079785.13922.F6. PMID: 12874476.


3. Malek M, Nematbakhsh M. 2015; Renal ischemia/reperfusion injury; from pathophysiology to treatment. J Renal Inj Prev. 4:20–27.
4. Wang Y, He J, Pei X, Zhao W. 2013; Systematic review and meta-analysis of mesenchymal stem/stromal cells therapy for impaired renal function in small animal models. Neph-rology (Carlton). 18:201–208. DOI: 10.1111/nep.12018. PMID: 23217027.


5. Sávio-Silva C, Soinski-Sousa PE, Balby-Rocha MTA, Lira ÁO, Rangel ÉB. 2020; Mesenchymal stem cell therapy in acute kidney injury (AKI): review and perspectives. Rev Assoc Med Bras (1992). 66(Suppl 1):s45–s54. DOI: 10.1590/1806-9282.66.s1.45. PMID: 31939535.


6. Barkholt L, Flory E, Jekerle V, Lucas-Samuel S, Ahnert P, Bisset L, Büscher D, Fibbe W, Foussat A, Kwa M, Lantz O, Mačiulaitis R, Palomäki T, Schneider CK, Sensebé L, Tachdjian G, Tarte K, Tosca L, Salmikangas P. 2013; Risk of tumorigenicity in mesenchymal stromal cell-based therapies--bridging scientific observations and regulatory viewpoints. Cytotherapy. 15:753–759. DOI: 10.1016/j.jcyt.2013.03.005. PMID: 23602595.


7. Cobo F, Cortés JL, Cabrera C, Nieto A, Concha A. 2007; Micro-biological contamination in stem cell cultures. Cell Biol Int. 31:991–995. DOI: 10.1016/j.cellbi.2007.03.010. PMID: 17452110.


8. Russo FP, Alison MR, Bigger BW, Amofah E, Florou A, Amin F, Bou-Gharios G, Jeffery R, Iredale JP, Forbes SJ. 2006; The bone marrow functionally contributes to liver fibrosis. Gastroenterology. 130:1807–1821. DOI: 10.1053/j.gastro.2006.01.036. PMID: 16697743.


9. McLeod CM, Mauck RL. 2017; On the origin and impact of mesenchymal stem cell heterogeneity: new insights and emerging tools for single cell analysis. Eur Cell Mater. 34:217–231. DOI: 10.22203/eCM.v034a14. PMID: 29076514. PMCID: PMC7735381. PMID: db3ca2601ebe4950ab7604eb84cd57fd.
10. Aum J, Jang MJ, Kim YS, Kim BH, An DH, Han JH, Suh N, Kim CS, You D. 2022; Comparison of stromal vascular fraction and adipose-derived stem cells for protection of renal function in a rodent model of ischemic acute kidney injury. Stem Cells Int. 2022:1379680. DOI: 10.1155/2022/1379680. PMID: 35578662. PMCID: PMC9107055. PMID: 1454da64c0b9474e87ba641bf1d012cb.


11. Tatsumi K, Ohashi K, Matsubara Y, Kohori A, Ohno T, Kakidachi H, Horii A, Kanegae K, Utoh R, Iwata T, Okano T. 2013; Tissue factor triggers procoagulation in transplanted mesenchymal stem cells leading to thromboembolism. Biochem Biophys Res Commun. 431:203–209. DOI: 10.1016/j.bbrc.2012.12.134. PMID: 23313481.


12. Sun DZ, Abelson B, Babbar P, Damaser MS. 2019; Harnessing the mesenchymal stem cell secretome for regenerative urology. Nat Rev Urol. 16:363–375. DOI: 10.1038/s41585-019-0169-3. PMID: 30923338. PMCID: PMC7027199.


13. Bi B, Schmitt R, Israilova M, Nishio H, Cantley LG. 2007; Stromal cells protect against acute tubular injury via an endocrine effect. J Am Soc Nephrol. 18:2486–2496. DOI: 10.1681/ASN.2007020140. PMID: 17656474.


14. Tarng DC, Tseng WC, Lee PY, Chiou SH, Hsieh SL. 2016; Induced pluripotent stem cell-derived conditioned medium attenuates acute kidney injury by downregulating the oxidative stress-related pathway in ischemia-reperfusion rats. Cell Transplant. 25:517–530. DOI: 10.3727/096368915X688542. PMID: 26132529. PMID: 759e93323c9c47ce86c4efe259d8baae.


15. Abedi A, Azarnia M, Jamali Zahvarehy M, Foroutan T, Golestani S. 2016; Effect of different times of intraperitoneal injections of human bone marrow mesenchymal stem cell conditioned medium on gentamicin-induced acute kidney injury. Urol J. 13:2707–2716. PMID: 27351327.
16. Xing L, Cui R, Peng L, Ma J, Chen X, Xie RJ, Li B. 2014; Mesenchymal stem cells, not conditioned medium, contribute to kidney repair after ischemia-reperfusion injury. Stem Cell Res Ther. 5:101. DOI: 10.1186/scrt489. PMID: 25145540. PMCID: PMC4159523.


17. Abouelkheir M, ElTantawy DA, Saad MA, Abdelrahman KM, Sobh MA, Lotfy A, Sobh MA. 2016; Mesenchymal stem cells versus their conditioned medium in the treatment of cisplatin-induced acute kidney injury: evaluation of efficacy and cellular side effects. Int J Clin Exp Med. 9:23222–23234.
18. Abd El Zaher F, El Shawarby A, Hammouda G, Bahaa N. 2017; Role of mesenchymal stem cells versus their conditioned medium on cisplatin-induced acute kidney injury in albino rat. A histological and immunohistochemical study. Egypt J Histol. 40:37–51. DOI: 10.21608/EJH.2017.3695.


19. Bhang SH, Cho SW, La WG, Lee TJ, Yang HS, Sun AY, Baek SH, Rhie JW, Kim BS. 2011; Angiogenesis in ischemic tissue produced by spheroid grafting of human adipose-derived stromal cells. Biomaterials. 32:2734–2747. DOI: 10.1016/j.biomaterials.2010.12.035. PMID: 21262528.


20. You D, Jang MJ, Kim BH, Song G, Lee C, Suh N, Jeong IG, Ahn TY, Kim CS. 2015; Comparative study of autologous stromal vascular fraction and adipose-derived stem cells for erectile function recovery in a rat model of cavernous nerve injury. Stem Cells Transl Med. 4:351–358. DOI: 10.5966/sctm.2014-0161. PMID: 25792486. PMCID: PMC4367505.


21. Bourin P, Bunnell BA, Casteilla L, Dominici M, Katz AJ, March KL, Redl H, Rubin JP, Yoshimura K, Gimble JM. 2013; Stromal cells from the adipose tissue-derived stromal vascular fraction and culture expanded adipose tissue-derived stromal/stem cells: a joint statement of the International Federation for Adipose Therapeutics and Science (IFATS) and the International Society for Cellular Therapy (ISCT). Cytotherapy. 15:641–648. DOI: 10.1016/j.jcyt.2013.02.006. PMID: 23570660. PMCID: PMC3979435.


22. Kim SG, You D, Kim K, Aum J, Kim YS, Jang MJ, Moon KH, Kang HW. 2022; Therapeutic effect of human mesenchymal stem cell-conditioned medium on erectile dysfunction. World J Mens Health. 40:653–662. DOI: 10.5534/wjmh.210121. PMID: 35021313. PMCID: PMC9482857. PMID: e698c795991d4255a5d0dad00eeb2308.


23. Jang MJ, You D, Park JY, Kim K, Aum J, Lee C, Song G, Shin HC, Suh N, Kim YM, Kim CS. 2018; Hypoxic preconditioned mesenchymal stromal cell therapy in a rat model of renal ischemia-reperfusion injury: development of optimal protocol to potentiate therapeutic efficacy. Int J Stem Cells. 11:157–167. DOI: 10.15283/ijsc18073. PMID: 30497128. PMCID: PMC6285294.


24. Melnikov VY, Faubel S, Siegmund B, Lucia MS, Ljubanovic D, Edelstein CL. 2002; Neutrophil-independent mechanisms of caspase-1- and IL-18-mediated ischemic acute tubular necrosis in mice. J Clin Invest. 110:1083–1091. DOI: 10.1172/JCI0215623. PMID: 12393844. PMCID: PMC150794.


25. Lee C, Jang MJ, Kim BH, Park JY, You D, Jeong IG, Hong JH, Kim CS. 2017; Recovery of renal function after administration of adipose-tissue-derived stromal vascular fraction in rat model of acute kidney injury induced by ischemia/reperfusion injury. Cell Tissue Res. 368:603–613. DOI: 10.1007/s00441-017-2585-0. PMID: 28283911.


26. d'Angelo M, Cimini A, Castelli V. 2020; Insights into the effects of mesenchymal stem cell-derived secretome in Parkinson's disease. Int J Mol Sci. 21:5241. DOI: 10.3390/ijms21155241. PMID: 32718092. PMCID: PMC7432166. PMID: f08f081b48a646f3a76ce03d25a2ac72.
27. Gnecchi M, Melo LG. 2009; Bone marrow-derived mesenchymal stem cells: isolation, expansion, characterization, viral transduction, and production of conditioned medium. Methods Mol Biol. 482:281–294. DOI: 10.1007/978-1-59745-060-7_18. PMID: 19089363.
28. Sun B, Guo S, Xu F, Wang B, Liu X, Zhang Y, Xu Y. 2014; Concentrated hypoxia-preconditioned adipose mesenchymal stem cell-conditioned medium improves wounds healing in full-thickness skin defect model. Int Sch Res Notices. 2014:652713. DOI: 10.1155/2014/652713. PMID: 27433483. PMCID: PMC4897251.


29. Santos JM, Camões SP, Filipe E, Cipriano M, Barcia RN, Filipe M, Teixeira M, Simões S, Gaspar M, Mosqueira D, Nascimento DS, Pinto-do-Ó P, Cruz P, Cruz H, Castro M, Miranda JP. 2015; Three-dimensional spheroid cell culture of umbilical cord tissue-derived mesenchymal stromal cells leads to enhanced paracrine induction of wound healing. Stem Cell Res Ther. 6:90. DOI: 10.1186/s13287-015-0082-5. PMID: 25956381. PMCID: PMC4448539.


30. Zhou F, Hui Y, Xu Y, Lei H, Yang B, Guan R, Gao Z, Xin Z, Hou J. 2016; Effects of adipose-derived stem cells plus insulin on erectile function in streptozotocin-induced diabetic rats. Int Urol Nephrol. 48:657–669. DOI: 10.1007/s11255-016-1221-3. PMID: 26820518.


31. Hwang SJ, Cho TH, Lee B, Kim IS. 2018; Bone-healing capacity of conditioned medium derived from three-dimensionally cultivated human mesenchymal stem cells and electrical stimulation on collagen sponge. J Biomed Mater Res A. 106:311–320. DOI: 10.1002/jbm.a.36224. PMID: 28884512.


32. Liu L, Gao J, Yuan Y, Chang Q, Liao Y, Lu F. 2013; Hypoxia preconditioned human adipose derived mesenchymal stem cells enhance angiogenic potential via secretion of increased VEGF and bFGF. Cell Biol Int. 37:551–560. DOI: 10.1002/cbin.10097. PMID: 23505143.


33. Hu X, Yu SP, Fraser JL, Lu Z, Ogle ME, Wang JA, Wei L. 2008; Transplantation of hypoxia-preconditioned mesenchymal stem cells improves infarcted heart function via enhanced survival of implanted cells and angiogenesis. J Thorac Cardiovasc Surg. 135:799–808. DOI: 10.1016/j.jtcvs.2007.07.071. PMID: 18374759.


34. Hsiao ST, Lokmic Z, Peshavariya H, Abberton KM, Dusting GJ, Lim SY, Dilley RJ. 2013; Hypoxic conditioning enhances the angiogenic paracrine activity of human adipose-derived stem cells. Stem Cells Dev. 22:1614–1623. DOI: 10.1089/scd.2012.0602. PMID: 23282141. PMCID: PMC3653395.


35. Byrne MB, Leslie MT, Gaskins HR, Kenis PJA. 2014; Methods to study the tumor microenvironment under controlled oxygen conditions. Trends Biotechnol. 32:556–563. DOI: 10.1016/j.tibtech.2014.09.006. PMID: 25282035. PMCID: PMC4254115.


36. Korff T, Kimmina S, Martiny-Baron G, Augustin HG. 2001; Blood vessel maturation in a 3-dimensional spheroidal coculture model: direct contact with smooth muscle cells regulates endothelial cell quiescence and abrogates VEGF responsiveness. FASEB J. 15:447–457. DOI: 10.1096/fj.00-0139com. PMID: 11156960.


37. Wei Q, Wang MH, Dong Z. 2005; Differential gender differences in ischemic and nephrotoxic acute renal failure. Am J Nephrol. 25:491–49. DOI: 10.1159/000088171. PMID: 16155358.


Fig. 1
Characterization of ADSC. (A) Flow cytometry of ADSC from rats in the ADSC-CM group. Results are expressed as mean±standard error (n=9). (B) Appearance of ADSC from rats in the ADSC-CM group after three weeks of induction of adipogenic, osteogenic, or chondrogenic differentiation. Magnification, ×100 in adipogenesis and osteogenesis and ×400 in chondrogenesis (n=3). ADSC: adipose-derived stem cell, ADSC-CM: adipose-derived stem cell-conditioned medium.
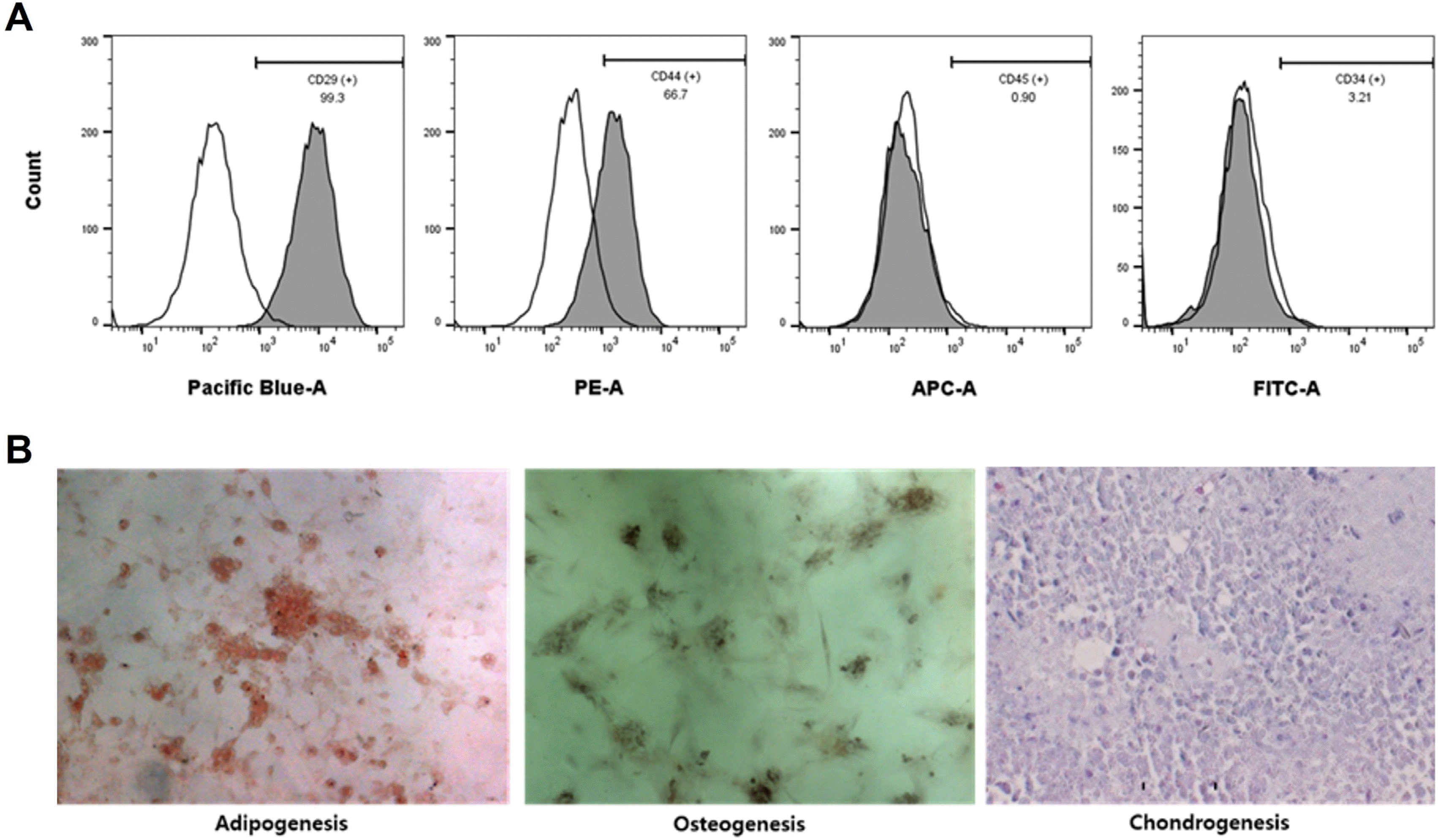
Fig. 2
Effects of ADSC-CM on renal function. (A) Changes in serum BUN, (B) serum creatinine, and (C) CrCl of each group during the experiment. Blue-colored stars indicate statistically significant differences in the ADSC-CM group compared with the IRI control group; **p<0.01; ***p<0.001. Three times per rat, ten rats per group, but nine rats in the ADSC-CM group. BUN: blood urea nitrogen, CrCl: creatinine clearance, IRI: ischemia-reperfusion injury, ADSC-CM: adipose-derived stem cell-conditioned medium.
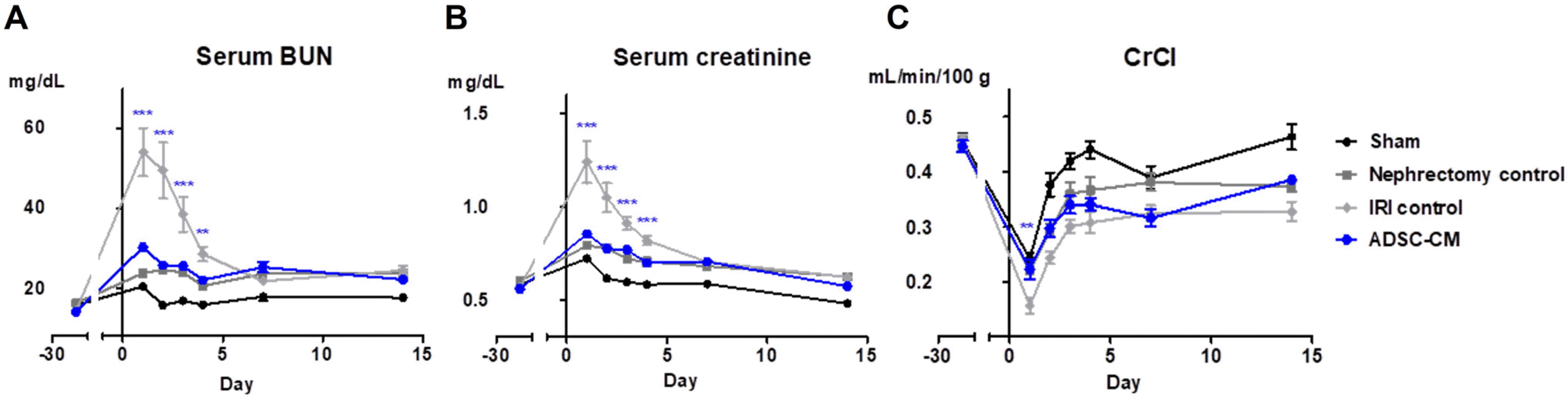
Fig. 3
Hematoxylin and eosin staining of kidney tissue. (A) Histopathological score in random non-overlapping fields. (B) Representative photomicrographs of cortex and medulla. Magnification ×40. Scale bar 50 μm. NS, p>0.05; ***p<0.001 compared with the IRI control group. Ten fields per rat, ten rats per group, but nine rats in the ADSC-CM group. NS: not significant, IRI: ischemia-reperfusion injury, ADSC-CM: adipose-derived stem cell-conditioned medium.
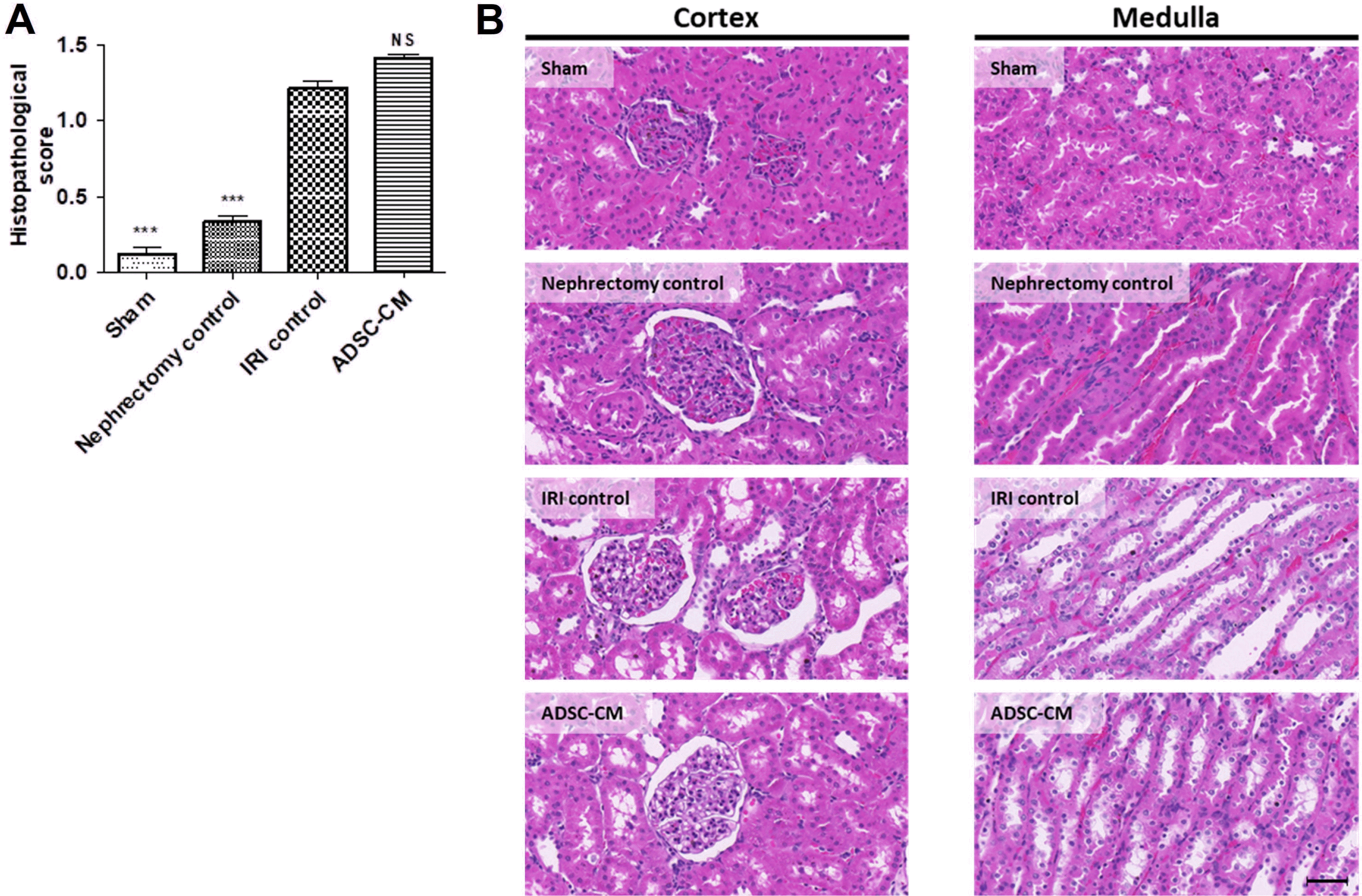
Fig. 4
Sirius red staining of kidney tissue. (A) The ratio of collagen-positive stained areas to random cortex and medulla areas. (B) Representative photomicrographs of cortex and medulla. Magnification ×40. Scale bar 50 μm. ***p<0.001 compared with the IRI control group. Four fields per rat, ten rats per group, but nine rats in the ADSC-CM group. IRI: ischemia-reperfusion injury, ADSC-CM: adipose-derived stem cell-conditioned medium.
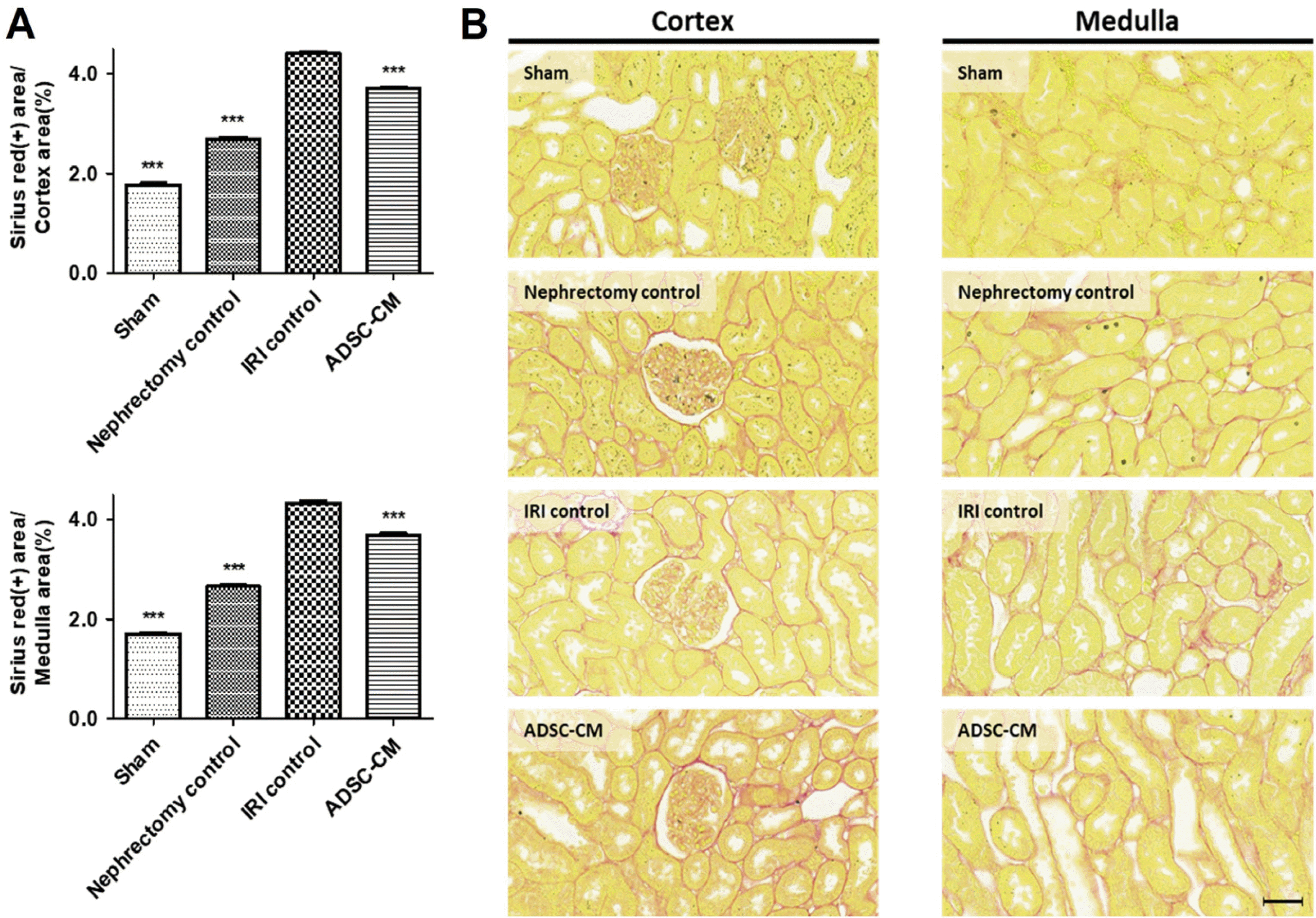
Fig. 5
TUNEL assay of kidney tissue. (A) The ratio of TUNEL-positive stained areas to random cortex and medulla areas. (B) Representative photomicrographs of cortex and medulla. Magnification ×40. Scale bar 50 μm. ***p<0.001 compared with the IRI control group. Four fields per rat, ten rats per group, but nine rats in the ADSC-CM group. TUNEL: terminal deoxynucleotidyl transferase dUTP nick end labeling, IRI: ischemia-reperfusion injury, ADSC-CM: adipose-derived stem cell-conditioned medium.
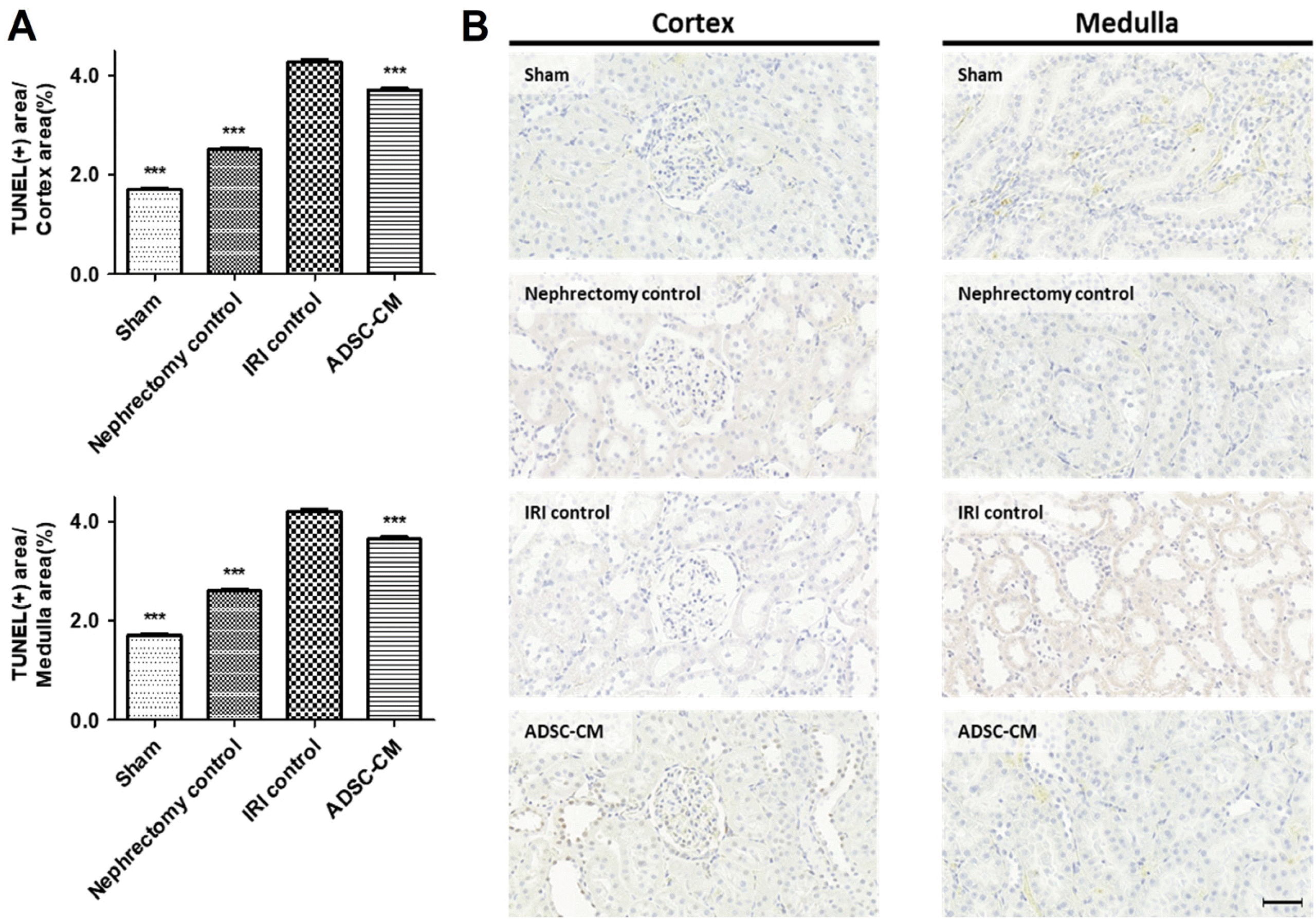
Fig. 6
Ki67 immunohistochemistry of kidney tissue. (A) The ratio of Ki67-positive stained areas to random cortex and medulla areas. (B) Representative photomicrographs of cortex and medulla. Magnification ×40. Scale bar 50 μm. ***p<0.001 compared with the IRI control group. Four fields per rat, ten rats per group, but nine rats in the ADSC-CM group. IRI: ischemia-reperfusion injury, ADSC-CM: adipose-derived stem cell-conditioned medium.
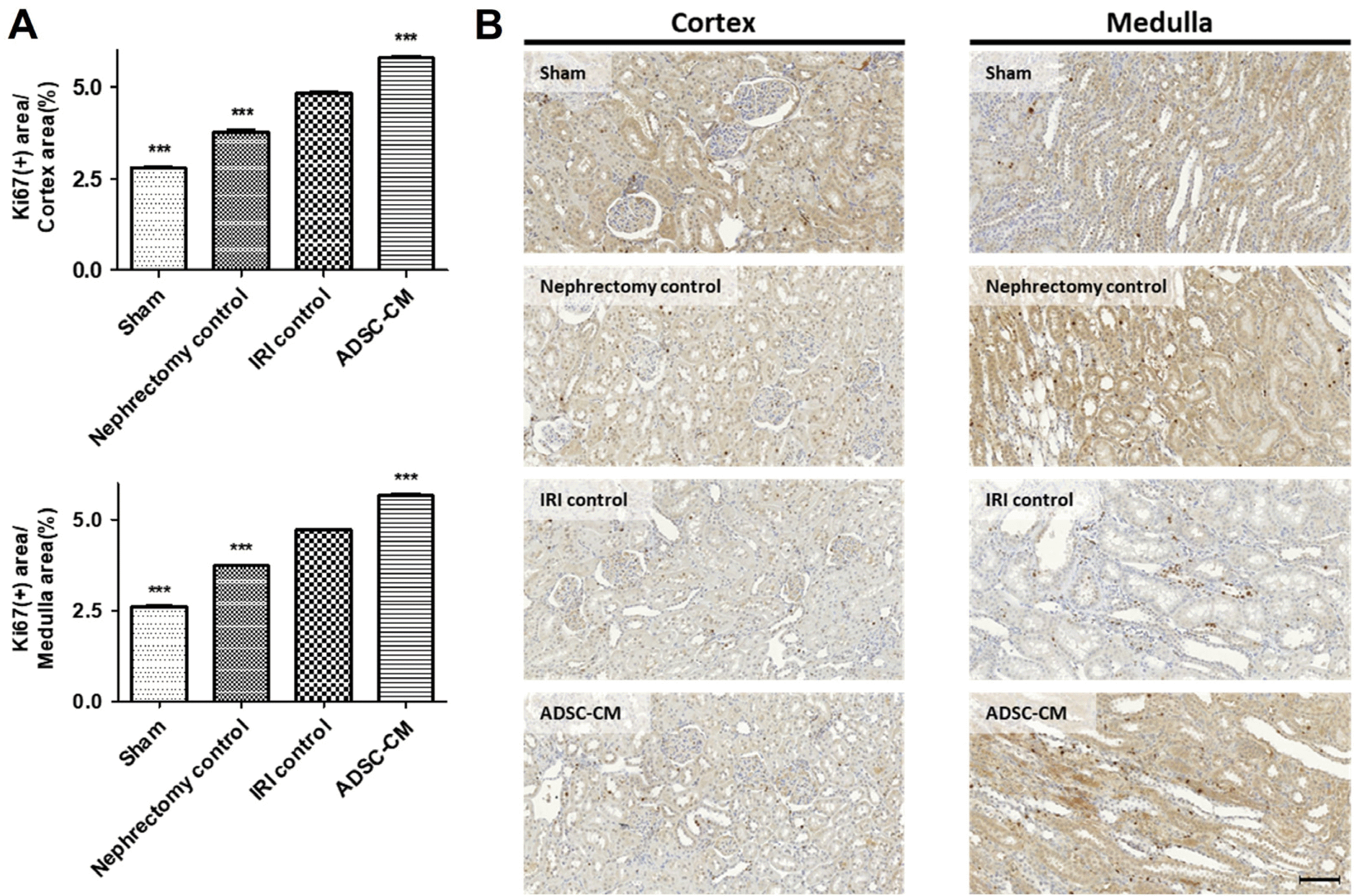
Fig. 7
GR immunohistochemistry of kidney tissue. (A) The ratio of GR-positive stained areas to the random cortex and medulla areas. (B) Representative photomicrographs of cortex and medulla. Magnification ×40. Scale bar 50 μm. ***p<0.001 compared with the IRI control group. Four fields per rat, ten rats per group, but nine rats in the ADSC-CM group. GR: glutathione reductase, IRI: ischemia-reperfusion injury, ADSC-CM: adipose-derived stem cell-conditioned medium.
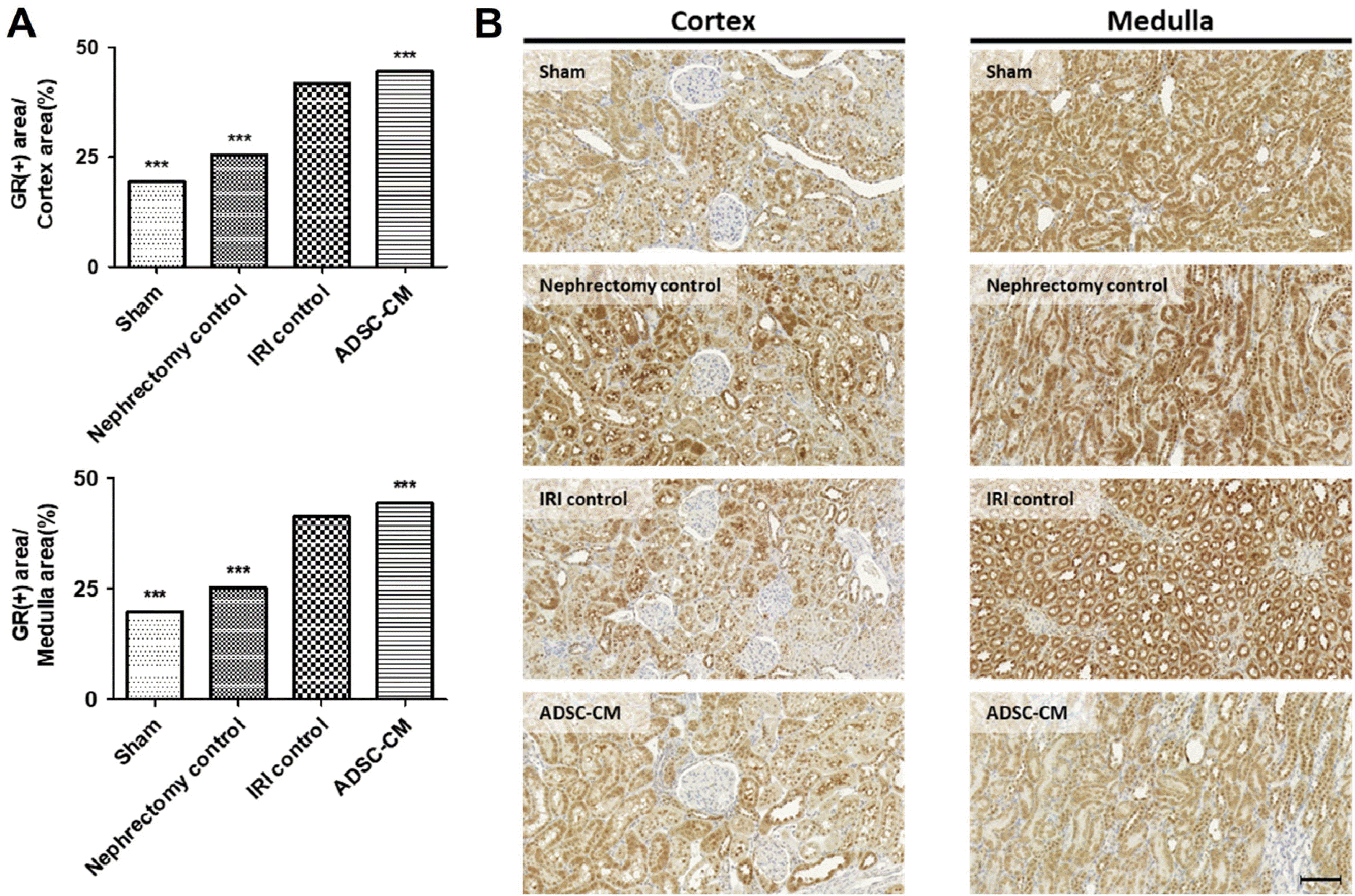
Fig. 8
GPx immunohistochemistry of kidney tissues. (A) The ratio of GPx-positive stained areas to random cortex and medulla areas. (B) Representative photomicrographs of cortex and medulla. Magnification ×40. Scale bar 50 μm. ***p<0.001 compared with the IRI control group. Four fields per rat, ten rats per group, but nine rats in the ADSC-CM group. GPx: glutathione peroxidase, IRI: ischemia-reperfusion injury, ADSC-CM: adipose-derived stem cell-conditioned medium.
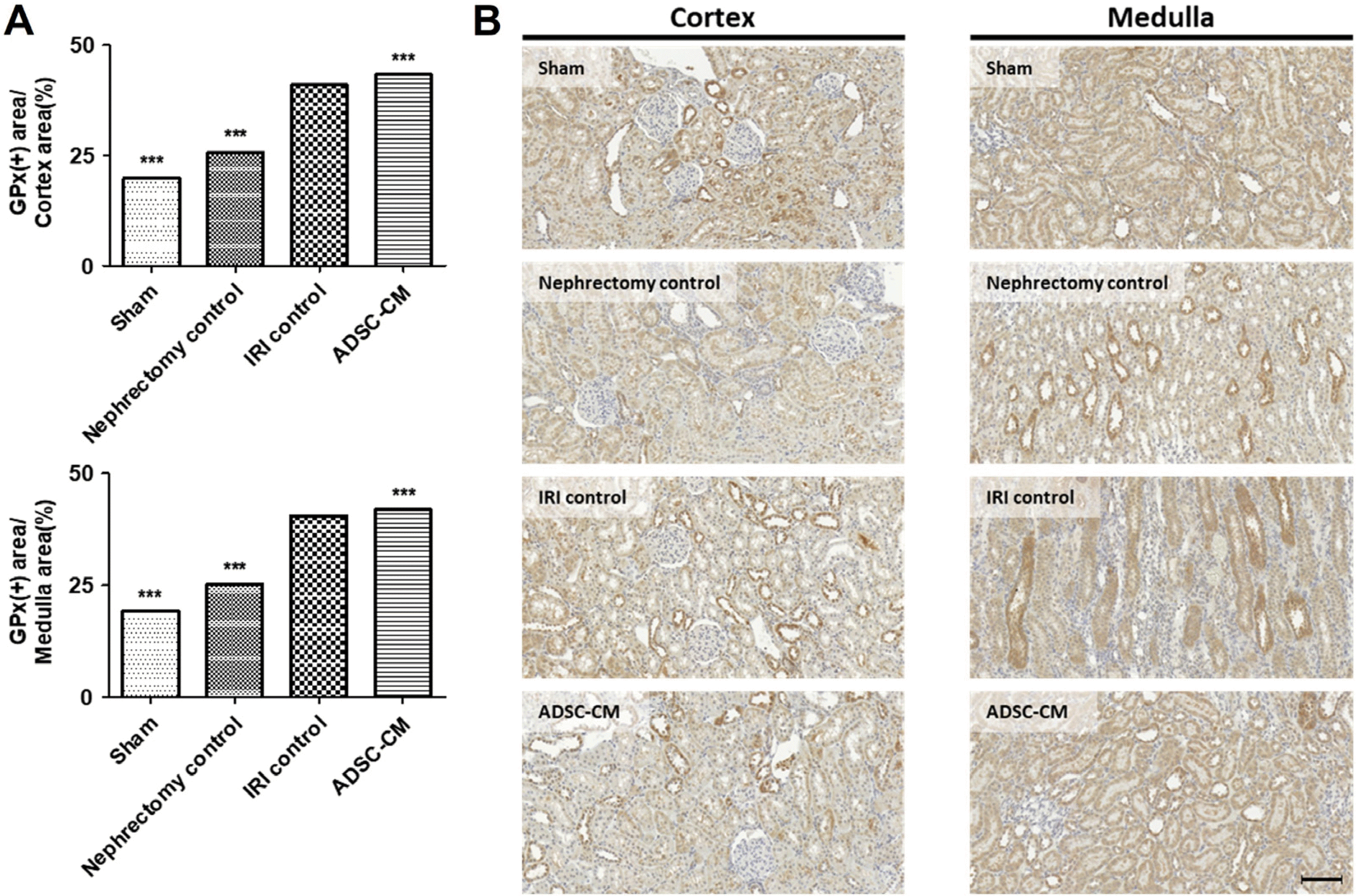