Abstract
Background and Objectives
DNA methyltransferases (Dnmts) play an important role in regulating DNA methylation during early developmental processes and cellular differentiation. In this study, we aimed to investigate the role of Dnmts in neural differentiation of embryonic stem cells (ESCs) and in maintenance of the resulting neural stem cells (NSCs).
Methods and Results
We used three types of Dnmt knockout (KO) ESCs, including Dnmt1 KO, Dnmt3a/3b double KO (Dnmt3 DKO), and Dnmt1/3a/3b triple KO (Dnmt TKO), to investigate the role of Dnmts in neural differentiation of ESCs. All three types of Dnmt KO ESCs could form neural rosette and differentiate into NSCs in vitro. Interestingly, however, after passage three, Dnmt KO ESC-derived NSCs could not maintain their self-renewal and differentiated into neurons and glial cells.
DNA methylation, a process of adding methyl groups to DNA bases (1), can regulate gene expression without changing the DNA sequence (2). DNA methyltransferases (Dnmts), which have enzymatic activity in DNA methylation, act as direct regulators of DNA methylation (3). In mammals, three active DNA methyltransferases, namely Dnmt1, Dnmt3A, and Dnmt3B, have been identified as direct regulators till date (4, 5). Among them, Dnmt1 is the most abundant and functions to maintain the methylated DNA state in both strands (6); it can bind to a hemimethylated double-stranded DNA and add methyl groups to an unmethylated strand during the DNA replication process (6). However, Dnmt3a and Dnmt3b, also called de-novo methyltransferases, can set up DNA methylation patterns without the footprints of methylation in DNA strands (7, 8). Due to such fundamental regulatory functions, Dnmts are considered to be associated with the development (9) and maintenance of stem cells (10), as well as with aging in mammals (11).
According to previous reports, Dnmts are essential proteins for cell proliferation and differentiation. Sen et al. (12) had reported that Dnmt1 maintains the function of self-renewal of progenitor cells in human skin cells. Georgia et al. (13). had reported that Dnmt1 maintains the survival of progenitor cells via repressing p53. Recently, Ramesh et al. (14) have reported that Dnmt1, in combination with ubiquitin-like with PHD and ring finger-1 (Uhrf1), maintains DNA methylation in neural stem cells (NSCs) and may be involved in the survival of NSCs. In hematopoietic stem cells (HSCs), Dnmt3a is suggested to be crucial (15), and loss of Dnmt1 could lead to biased differentiation into myeloerythroid and lymphoid-deficient cells (16). However, there have been very few reports on how both DNA methylation and DNA methyltransferase can affect the maintenance and differentiation of NSCs.
Several research groups have been studying the mechanisms underlying the maintenance and differentiation of NSCs. Although epidermal growth factor (EGF) and fibroblast growth factor 2 (FGF2) are known to mainly control the proliferation and self-renewal of neural stem/progenitor cells in vivo and in vitro (17), the specific mechanism underlying neural stem/progenitor cell regulation still remains unknown (18-20). As an epigenetic regulation, DNA methylation has been suggested as a major factor in brain development and neurogenesis (21, 22). However, studies on the direct link between Dnmts and the proliferation and differentiation of NSCs have not been reported yet.
Tsumura et al. (5) had shown, via a knockout (KO) experiment in mice, that mouse embryonic stem cells (ESCs) can be maintained and self-renewed in the absence of Dnmt1 and Dnmt3a/3b. Liao et al. (23) had shown targeted disruption of Dnmt1 to be critical for the viability of human ESCs, although not so for Dnmt3a/b. Thus, Dnmt KO ESC lines could be useful resources for studies on the differentiation of pluripotent stem cells. In this paper, we hypothesized that ES cells defective in DNA methylation, due to the KO of Dnmt1 or Dnmt3a/3b, or due to triple KO of all three Dnmts, could differentiate into NSCs. However, the derived NSCs lacked the ability to maintain stemness during a long-term culture. Hence, we suggested that regulation of DNA methylation is critical for the maintenance of NSCs.
The Dnmt1 KO, Dnmt3 DKO, and Dnmt TKO ES cell lines used in this study had been described in previous publications (5, 24). Olig2-GFP ES cells were used as control ES cells for monitoring the stemness of NSCs, since GFP fluorescence was controlled by Olig2, which is expressed in NSCs (25).
Dnmt KO ES cells and Olig2-GFP ESCs were allowed to differentiate into NSCs using the previously described protocol (25). Established NSCs were maintained in the NS medium, consisting of Dulbecco’s modified Eagle’s medium (Nutrient Mixture F-12; DMEM/F12) (Gibco, 1132-033) supplemented with 1×N2 supplement (Gibco, 17502-048), 1×penicillin/streptomycin/glutamine (P/S/G) (Gibco, 10378-016), 10 ng/ml recombinant human epidermal growth factor (EGF) (Gibco, PHG0311), 10 ng/ml basic fibroblast growth factor (bFGF) (R&D systems, 233-FB-500), 50 mg/ml bovine serum albumin fraction V (BSA) (Gibco, 15260-037), and 1 mM β-mercaptoethanol (Gibco, 21985-023). Several ESC lines were maintained in the mES medium, consisting of low-glucose DMEM (Hyclone, 11885-084) supplemented with 15% heat-inactivated fetal bovine serum (FBS) (Hyclone), 1×P/S/G (Gibco, 10378-016), 0.1 mM nonessential amino acids (NEAA) (Gibco, 1140-050), 1 mM β-mercaptoethanol (Gibco, 21985-023), and 103 U/ml leukemia inhibitory factor (ESGRO, Merck Millipore).
Several ESC lines were cultured for 2∼3 days in mEF medium, consisting of low-glucose DMEM (Hyclone, 11885-084) supplemented with 15% heat-inactivated FBS (Hyclone), 1×P/S/G (Gibco, 10378-016), 0.1 mM NEAA (Gibco, 1140-050), and 1 mM β-mercaptoethanol (Gibco, 21985-023) in suspension culture dishes. Thereafter, embryoid bodies (EBs) from ESCs were cultured in the N2B27 medium, consisting of 1:1 mixture of DMEM/12 medium (Gibco, 11320-033) and neurobasal medium (Gibco, 21103-49) supplemented with 1×N2 supplement (Gibco, 17502-48), 1×B27 supplement (Gibco, 17504-44), 1×P/S/G (Gibco, 10378-016), 20 ng/ml EGF (Gibco, PHG0311), and 20 ng/ml bFGF (R&D systems, 233-FB-00), for 3 days in suspension culture dishes. Next, the EBs were plated onto a 0.15% gelatin-coated dish for 3 days in NS medium. After a few days, NSC-like structure was observed. The established NSCs were maintained thereafter, and passaged in NS medium on a 0.15% gelatin-coated dish.
For immunocytochemistry, the cells were cultured in 4-well dishes until 70∼80% confluency. Thereafter, they were fixed with 4% paraformaldehyde for 30 min at 4℃, washed with phosphate-buffered saline (PBS) (Welgene, ML 008-01), and treated with 0.3% Triton X-100 in PBS for 10 min. Next, the cells were blocked with PBS containing 3% bovine serum albumin and 0.3% Triton X-100 for 30 min at 25℃. The blocked cells were then incubated overnight at 4℃ with the following primary antibodies: Oct4 (1:1,000; Santa Cruz Biotechnology), Nanog (1:1,000; Abcam), Tuj1 (1:1,000; Millipore), GFAP (1:1,000, Abcam), and O1 (1:1,000, eBioscience). The following day, incubated cells were washed thrice with PBS for 10 min and incubated with fluorescently labeled (Alexa Fluor 488 or 568; Abcam) secondary antibodies. The latter were used according to the manufacturer’s specifications. Cells were finally washed and stained with 10 μg/ml Hoechst (Hoechst 33342; Thermo Fisher Scie-ntific, H3570) and 0.3% Triton X-100 in PBS for 2 min at 25℃.
A 6-cm dish at 50% cell confluency was treated with 0.3 mg/ml Nocodazole for 16 hours. Cells were recovered by trypsinization and treated with hypotonic (0.56% wt/vol) KCl solution for 15 minutes at 37℃, and pelleted by centrifugation. Cells were then fixed by washing three times in fresh fixative (3:1 methanol: glacial acetic acid), and dropped onto clean glass slides. The slides were air dried, stained with 10% Giemsa (Sigma), and observed under a microscope.
A previous report had shown that deficiency of DNA methylation did not affect the self-renewal ability of mouse ESCs (5). However, there has been no report about the effect of DNA methylation on self-renewal and differentiation of NSCs. Thus, we aimed to investigate the correlation between NSC maintenance and impaired regulation of DNA methylation. As a preliminary approach, we treated Olig2-GFP NSCs with 5-AzaC, a Dnmt inhibitor directly incorporated into DNA and RNA (26), in order to perturb the regulation of DNA methylation in NSCs. Different concentrations of 5-AzaC, such as 25, 50, and 100 μM, were added to NSCs in the culture (Fig. 1A); on day 4∼5 after 5-AzaC treatment, Olig2-GFP NSCs treated with 50 and 100 μM 5-AzaC ceased to proliferate, and died (Fig. 1A). Although Olig2-GFP NSCs treated with 25 μM 5-AzaC still survived (Fig. 1A), they showed significantly lower cell numbers after passage 2 (approxi-mately day 6) (Fig. 1B). We concluded that demethylation of DNA impaired the self-renewal ability of NSCs. Next, to check the effect of 5-AzaC treatment on pluripotent stem cells, we treated ESCs with 25, 50, and 100 μM 5-AzaC. Interestingly, the treatment did not affect the self-renewal abilities of ESCs (Supplementary Fig. S1). Collectively, the results suggested that inhibition of Dnmts by 5-AzaC treatment could lead to a severe effect on the proliferation and survival of NSCs, but not on those of ESCs.
Since we had demonstrated DNA methylation as a possible factor in the maintenance of NSCs, we next investigated whether ESCs deficient in Dnmts could be differentiated into NSCs. We used three types of Dnmt knockout (KO) ESCs, including Dnmt1 KO, Dnmt3a/3b double KO (Dnmt3 DKO), and Dnmt1/3a/3b triple KO (Dnmt TKO), to investigate the effect of Dnmt KO on the differentiation potential of ESCs into NSCs. In addition, Olig2-GFP ESCs, expressing GFP under the control of Olig2 (25), the latter being expressed in NSCs, were used as control ESCs for checking the differentiation into NSCs. Immunocyto-chemical analysis revealed that all three KO cell lines (Dnmt1 KO at passage 17, Dnmt3 DKO at passage 11, and Dnmt TKO ESCs at passage 12) as well as the control ESCs (Olig2-GFP ESCs at passage 8) expressed the pluripotency markers Oct4 and Nanog (Fig. 2A), as was consistent with previous studies (5, 7).
To identify the differentiation potential of Dnmt KO ESCs into NSCs, we allowed the differentiation of all three Dnmt KO ESC lines and control ESCs into NSCs via EB and neurosphere formation in EGF- and bFGF-containing medium, according to a previously described protocol (25, 27). All Dnmt KO and control ESC lines successfully formed EBs on day 3 of neural lineage differentiation protocol (Fig. 2B). We also observed the neural rosette structures (Fig. 2C), which expressed NSC markers Sox2 and Nestin in Dnmt KO ESCs and Olig2-GFP in control ESCs, on day 9 after differentiation (Fig. 2C). After passaging the rosette structures onto the gelatin-coated dish in NSC medium, we could establish NSC lines from all Dnmt KO and control ESCs (Fig. 2D). The established NSCs from Dnmt KO ESC lines (Dnmt1 KO, Dnmt3 DKO, and Dnmt TKO NSCs) showed similar morphologies as in those from control Olig2-GFP ESCs (Fig. 2D). The results indicated the differentiation potential of ESCs into NSCs to not be affected by Dnmt deficiency.
During a subsequent culture, Dnmt KO ESC-derived NSC lines (Dnmt1 KO, Dnmt3 DKO, and Dnmt TKO NSCs) lost NSC marker expression and slender bipolar morphology after passage 3, whereas control NSCs (Olig2-GFP NSCs), derived from control ESCs, retained both NSC marker expression and typical NSC morphology (Fig. 3). We conducted NSC-derivation experiment thrice using Dnmt KO ESCs; all failed to maintain NSCs beyond passage 3. Thus, we speculated that if the Dnmt KO ESC-derived NSCs could not maintain self-renewal ability, they spontaneously differentiated into neural subtypes, such as neurons and glial cells. To identify whether NSCs were differentiated into neurons, astrocytes, and oligodendrocytes during the passage, we conducted immunocyto-chemical analysis using Tuj1 (neuronal marker), GFAP (astrocyte marker), and O1 (oligodendrocyte marker) (Fig. 4A). All samples from Dnmt KO NSCs stained positive for Tuj1, GFAP, and O1 (Fig. 4A); however, NSCs differentiated from control ESCs maintained self-renewal and did not get stained by the antibodies for Tuj1, GFAP, and O1 (Fig. 4A). Control and Dnmt KO cell lines also maintained normal diploid karyotypes (Fig. 4B). Taken together, we found that Dnmt KO ESCs differentiated into NSCs, which subsequently lost self-renewal ability and spontaneously differentiated into neural subtypes.
In this study, we investigated the mechanism by which Dnmt deficiency affects the maintenance and self-renewal ability of ESCs and NSCs. Three Dnmt KO ESC lines (Dnmt1 KO, Dnmt3 DKO, and Dnmt TKO ESCs) showed normal self-renewal ability, as described previously by other researchers (7, 24). Although we cannot explain the mechanism by which ESCs tolerate the Dnmt deficiency and defective regulation of DNA methylation, we proposed that ESCs must be special cell types that maintain stemness even without the essential genes. They are known to be able to maintain self-renewal capacities without the critical genes associated with DNA demethylases (Tet1 and Tet3) (28, 29), mitochondria, energy metabolism (Dnm1l, Mff, and Fis1) (30), pluripotency marker Nanog (31), and so on (32, 33).
Three Dnmt KO ESC lines showed efficient differentiation into NSCs, indicating that defective regulation of DNA methylation had no effect on their directed differentiation into NSCs. However, Dnmt KO ESC-derived NSC lines could not maintain stem-cell property and eventually differentiated into neural subtypes, such as neurons and glial cells, indicating that regulation of DNA methylation is critical for the maintenance of NSCs, though not for their differentiation ability. Although the differentiated neurons and glial cells expressed the specific markers, we did not further analyze the functionality of these cells; deficiency of Dnmts might affect the survival or biological functions of neurons and glial cells. The molecular mechanisms underlying the effect of Dnmts on the maintenance and proliferation of NSCs have not yet been elucidated. Okano et al. (7) had demonstrated that Dnmt KO mice died shortly after birth, which could be partly explained by the observation of Dnmt1 and Dnmt3a expression in adult brain (34); Dnmt1 or Dnmt3a KO in neural stem/progenitor cells could affect the survival of newly generated neurons in vivo (35, 36). Noguchi et al. (35) had also shown that the deficiency of Dnmt in NSCs affects the normal development of dentate gyrus, resulting in a reduced volume of granule cell layer.
Till date, numerous research groups have explored the specific mechanisms involved in the maintenance and differentiation of NSCs, such as signaling pathways and epigenetic regulation. Although the most well-known factors for the self-renewal of NSCs, both in vivo and in vitro, are epidermal growth factor (EGF) and fibroblast growth factor 2 (FGF2) (17), the specific mechanism for maintenance of neural stem/progenitor cells still remains unknown (18-20). Sato et al. (17) had reported that the PI3K and mTOR pathways constitute the underlying mechanism for the survival of NSCs. The crosstalk of notch and growth factor/cytokine signaling pathways could also be related to the self-renewal and cell fate specification of NSCs (37, 38). Recently, Engler et al. (39) have shown that Notch1 and Notch2 signaling is essential for the transition from quiescence state to neural differentiation in mouse brain. The Wnt (40) and BMP signaling (41) pathways are also involved in adult neurogenesis and neuronal function. As an epigenetic regulation, DNA methylation has been suggested as the major factor underlying brain development and neurogenesis (21, 22). In relation to DNA methylation, ten-eleven translocation (TET) 1, 2, and 3 proteins are expressed in brain and are associated with both NSC formation and differentiation (22, 29). TET1 protein could regulate hippocampal neurogenesis (42) while TET3 was shown to play a critical role in the maintenance and terminal differentiation of neural progenitor cells (29). In addition, methyl-CpG-biding domain 1 (MBD1), which is expressed in NSCs and adult neurons, could orchestrate NSC maintenance and neuronal commitment (43), and the balance between stem cell state and commitment could be controlled via MBD1-regulated miR-184 (44). In this study, we showed that deletion of Dnmt family could disrupt the maintenance of NSCs and induce their differentiation. However, further studies would be required for a better understanding of the mechanism of Dnmt in the regulation of maintenance and differentiation of NSCs.
Supplementary data including one figure can be found with this article online at https://doi.org/10.15283/ijsc22138.
Notes
Author Contributions
Bong Jong Seo, Tae Kyung Hong and Jeong Tae Do wrote the manuscript and designed the experiments. Bong Jong Seo, Tae Kyung Hong and Jae Hoon Song performed experiments and analyzed the data. Bong Jong Seo, Tae Kyung Hong, Sang Hoon Yoon, Sang Jun Uhm, Hyuk Song, Kwonho Hong, Hans R. Schöler and Jeong Tae Do performed data analysis.
References
1. Zemach A, McDaniel IE, Silva P, Zilberman D. 2010; Genome-wide evolutionary analysis of eukaryotic DNA methylation. Science. 328:916–919. DOI: 10.1126/science.1186366. PMID: 20395474.
2. Weber M, Hellmann I, Stadler MB, Ramos L, Pääbo S, Rebhan M, Schübeler D. 2007; Distribution, silencing potential and evolutionary impact of promoter DNA methylation in the human genome. Nat Genet. 39:457–466. DOI: 10.1038/ng1990. PMID: 17334365.
3. Leonhardt H, Page AW, Weier HU, Bestor TH. 1992; A targeting sequence directs DNA methyltransferase to sites of DNA replication in mammalian nuclei. Cell. 71:865–873. DOI: 10.1016/0092-8674(92)90561-P. PMID: 1423634.
4. Palii SS, Van Emburgh BO, Sankpal UT, Brown KD, Robertson KD. 2008; DNA methylation inhibitor 5-Aza-2'-deoxy-cytidine induces reversible genome-wide DNA damage that is distinctly influenced by DNA methyltransferases 1 and 3B. Mol Cell Biol. 28:752–771. DOI: 10.1128/MCB.01799-07. PMID: 17991895. PMCID: PMC2223421.
5. Tsumura A, Hayakawa T, Kumaki Y, Takebayashi S, Sakaue M, Matsuoka C, Shimotohno K, Ishikawa F, Li E, Ueda HR, Nakayama J, Okano M. 2006; Maintenance of self-renewal ability of mouse embryonic stem cells in the absence of DNA methyltransferases Dnmt1, Dnmt3a and Dnmt3b. Genes Cells. 11:805–814. DOI: 10.1111/j.1365-2443.2006.00984.x. PMID: 16824199.
6. Bronner C. 2011; Control of DNMT1 abundance in epigenetic inheritance by acetylation, ubiquitylation, and the histone code. Sci Signal. 4:pe3. DOI: 10.1126/scisignal.2001764. PMID: 21266713.
7. Okano M, Bell DW, Haber DA, Li E. 1999; DNA methyltransferases Dnmt3a and Dnmt3b are essential for de novo methylation and mammalian development. Cell. 99:247–257. DOI: 10.1016/S0092-8674(00)81656-6. PMID: 10555141.
8. Hu YG, Hirasawa R, Hu JL, Hata K, Li CL, Jin Y, Chen T, Li E, Rigolet M, Viegas-Péquignot E, Sasaki H, Xu GL. 2008; Regulation of DNA methylation activity through Dnmt3L promoter methylation by Dnmt3 enzymes in embryonic development. Hum Mol Genet. 17:2654–2664. DOI: 10.1093/hmg/ddn165. PMID: 18544626.
9. Kato Y, Kaneda M, Hata K, Kumaki K, Hisano M, Kohara Y, Okano M, Li E, Nozaki M, Sasaki H. 2007; Role of the Dnmt3 family in de novo methylation of imprinted and repetitive sequences during male germ cell development in the mouse. Hum Mol Genet. 16:2272–2280. DOI: 10.1093/hmg/ddm179. PMID: 17616512.
10. Chédin F. 2011; The DNMT3 family of mammalian de novo DNA methyltransferases. Prog Mol Biol Transl Sci. 101:255–285. DOI: 10.1016/B978-0-12-387685-0.00007-X. PMID: 21507354.
11. Xiao Y, Word B, Starlard-Davenport A, Haefele A, Lyn-Cook BD, Hammons G. 2008; Age and gender affect DNMT3a and DNMT3b expression in human liver. Cell Biol Toxicol. 24:265–272. DOI: 10.1007/s10565-007-9035-9. PMID: 17929180.
12. Sen GL, Reuter JA, Webster DE, Zhu L, Khavari PA. 2010; DNMT1 maintains progenitor function in self-renewing somatic tissue. Nature. 463:563–567. DOI: 10.1038/nature08683. PMID: 20081831. PMCID: PMC3050546.
13. Georgia S, Kanji M, Bhushan A. 2013; DNMT1 represses p53 to maintain progenitor cell survival during pancreatic organo-genesis. Genes Dev. 27:372–377. DOI: 10.1101/gad.207001.112. PMID: 23431054. PMCID: PMC3589554.
14. Ramesh V, Bayam E, Cernilogar FM, Bonapace IM, Schulze M, Riemenschneider MJ, Schotta G, Götz M. 2016; Loss of Uhrf1 in neural stem cells leads to activation of retrovi-ral elements and delayed neurodegeneration. Genes Dev. 30:2199–2212. DOI: 10.1101/gad.284992.116. PMID: 27798843. PMCID: PMC5088568.
15. Challen GA, Sun D, Jeong M, Luo M, Jelinek J, Berg JS, Bock C, Vasanthakumar A, Gu H, Xi Y, Liang S, Lu Y, Darlington GJ, Meissner A, Issa JP, Godley LA, Li W, Goodell MA. 2011; Dnmt3a is essential for hematopoietic stem cell differentiation. Nat Genet. 44:23–31. DOI: 10.1038/ng.1009. PMID: 22138693. PMCID: PMC3637952.
16. Bröske AM, Vockentanz L, Kharazi S, Huska MR, Mancini E, Scheller M, Kuhl C, Enns A, Prinz M, Jaenisch R, Nerlov C, Leutz A, Andrade-Navarro MA, Jacobsen SE, Rosenbauer F. 2009; DNA methylation protects hematopoietic stem cell multipotency from myeloerythroid restriction. Nat Genet. 41:1207–1215. DOI: 10.1038/ng.463. PMID: 19801979.
17. Sato A, Sunayama J, Matsuda K, Tachibana K, Sakurada K, Tomiyama A, Kayama T, Kitanaka C. 2010; Regulation of neural stem/progenitor cell maintenance by PI3K and mTOR. Neurosci Lett. 470:115–120. DOI: 10.1016/j.neulet.2009.12.067. PMID: 20045038.
18. Campos LS, Leone DP, Relvas JB, Brakebusch C, Fässler R, Suter U, ffrench-Constant C. 2004; Beta1 integrins activate a MAPK signalling pathway in neural stem cells that contributes to their maintenance. Development. 131:3433–3444. DOI: 10.1242/dev.01199. PMID: 15226259.
19. Braunschweig L, Meyer AK, Wagenführ L, Storch A. 2015; Oxygen regulates proliferation of neural stem cells through Wnt/β-catenin signalling. Mol Cell Neurosci. 67:84–92. DOI: 10.1016/j.mcn.2015.06.006. PMID: 26079803.
20. Ehm O, Göritz C, Covic M, Schäffner I, Schwarz TJ, Karaca E, Kempkes B, Kremmer E, Pfrieger FW, Espinosa L, Bigas A, Giachino C, Taylor V, Frisén J, Lie DC. 2010; RBPJkappa-dependent signaling is essential for long-term maintenance of neural stem cells in the adult hippocampus. J Neurosci. 30:13794–13807. DOI: 10.1523/JNEUROSCI.1567-10.2010. PMID: 20943920. PMCID: PMC6633732.
21. Ladd-Acosta C, Pevsner J, Sabunciyan S, Yolken RH, Webster MJ, Dinkins T, Callinan PA, Fan JB, Potash JB, Feinberg AP. 2007; DNA methylation signatures within the human brain. Am J Hum Genet. 81:1304–1315. DOI: 10.1086/524110. PMID: 17999367. PMCID: PMC2276356.
22. Jang HS, Shin WJ, Lee JE, Do JT. 2017; CpG and Non-CpG methylation in epigenetic gene regulation and brain function. Genes (Basel). 8:148. DOI: 10.3390/genes8060148. PMID: 28545252. PMCID: PMC5485512.
23. Liao J, Karnik R, Gu H, Ziller MJ, Clement K, Tsankov AM, Akopian V, Gifford CA, Donaghey J, Galonska C, Pop R, Reyon D, Tsai SQ, Mallard W, Joung JK, Rinn JL, Gnirke A, Meissner A. 2015; Targeted disruption of DNMT1, DNMT3A and DNMT3B in human embryonic stem cells. Nat Genet. 47:469–478. DOI: 10.1038/ng.3258. PMID: 25822089. PMCID: PMC4414868.
24. Biniszkiewicz D, Gribnau J, Ramsahoye B, Gaudet F, Eggan K, Humpherys D, Mastrangelo MA, Jun Z, Walter J, Jaenisch R. 2002; Dnmt1 overexpression causes genomic hypermethylation, loss of imprinting, and embryonic lethality. Mol Cell Biol. 22:2124–2135. DOI: 10.1128/MCB.22.7.2124-2135.2002. PMID: 11884600. PMCID: PMC133685.
25. Choi HW, Kim JS, Choi S, Hong YJ, Kim MJ, Seo HG, Do JT. 2014; Neural stem cells differentiated from iPS cells spontaneously regain pluripotency. Stem Cells. 32:2596–2604. DOI: 10.1002/stem.1757. PMID: 24898298.
26. Müller AM, Florek M. 2014; 5-Azacytidine/5-Azacitidine. Recent Results Cancer Res. 201:299–324. DOI: 10.1007/978-3-642-54490-3_19. PMID: 24756801.
27. Conti L, Pollard SM, Gorba T, Reitano E, Toselli M, Biella G, Sun Y, Sanzone S, Ying QL, Cattaneo E, Smith A. 2005; Niche-independent symmetrical self-renewal of a mammalian tissue stem cell. PLoS Biol. 3:e283. DOI: 10.1371/journal.pbio.0030283. PMID: 16086633. PMCID: PMC1184591. PMID: 12caa13fe1cc4e2781031e1d2981ad9c.
28. Dawlaty MM, Ganz K, Powell BE, Hu YC, Markoulaki S, Cheng AW, Gao Q, Kim J, Choi SW, Page DC, Jaenisch R. 2011; Tet1 is dispensable for maintaining pluripotency and its loss is compatible with embryonic and postnatal development. Cell Stem Cell. 9:166–175. DOI: 10.1016/j.stem.2011.07.010. PMID: 21816367. PMCID: PMC3154739.
29. Li T, Yang D, Li J, Tang Y, Yang J, Le W. 2015; Critical role of Tet3 in neural progenitor cell maintenance and terminal differentiation. Mol Neurobiol. 51:142–154. DOI: 10.1007/s12035-014-8734-5. PMID: 24838624.
30. Seo BJ, Choi J, La H, Habib O, Choi Y, Hong K, Do JT. 2020; Role of mitochondrial fission-related genes in mitochondrial morphology and energy metabolism in mouse embryonic stem cells. Redox Biol. 36:101599. DOI: 10.1016/j.redox.2020.101599. PMID: 32521505. PMCID: PMC7286981.
31. Chambers I, Silva J, Colby D, Nichols J, Nijmeijer B, Robertson M, Vrana J, Jones K, Grotewold L, Smith A. 2007; Nanog safeguards pluripotency and mediates germline development. Nature. 450:1230–1234. DOI: 10.1038/nature06403. PMID: 18097409.
32. Tateishi S, Niwa H, Miyazaki J, Fujimoto S, Inoue H, Yamaizumi M. 2003; Enhanced genomic instability and defective postreplication repair in RAD18 knockout mouse embryonic stem cells. Mol Cell Biol. 23:474–481. DOI: 10.1128/MCB.23.2.474-481.2003. PMID: 12509447. PMCID: PMC151530.
33. Langton S, Gudas LJ. 2008; CYP26A1 knockout embryonic stem cells exhibit reduced differentiation and growth arrest in response to retinoic acid. Dev Biol. 315:331–354. DOI: 10.1016/j.ydbio.2007.12.021. PMID: 18241852.
34. Murao N, Noguchi H, Nakashima K. 2016; Epigenetic regulation of neural stem cell property from embryo to adult. Neuroe-pigenetics. 5:1–10. DOI: 10.1016/j.nepig.2016.01.001.
35. Noguchi H, Kimura A, Murao N, Matsuda T, Namihira M, Nakashima K. 2015; Expression of DNMT1 in neural stem/precursor cells is critical for survival of newly generated neurons in the adult hippocampus. Neurosci Res. 95:1–11. DOI: 10.1016/j.neures.2015.01.014. PMID: 25659757.
36. Wu H, Coskun V, Tao J, Xie W, Ge W, Yoshikawa K, Li E, Zhang Y, Sun YE. 2010; Dnmt3a-dependent nonpromoter DNA methylation facilitates transcription of neurogenic genes. Science. 329:444–448. DOI: 10.1126/science.1190485. PMID: 20651149. PMCID: PMC3539760.
37. Gaiano N, Fishell G. 2002; The role of notch in promoting glial and neural stem cell fates. Annu Rev Neurosci. 25:471–490. DOI: 10.1146/annurev.neuro.25.030702.130823. PMID: 12052917.
38. Aguirre A, Rubio ME, Gallo V. 2010; Notch and EGFR pathway interaction regulates neural stem cell number and self-renewal. Nature. 467:323–327. DOI: 10.1038/nature09347. PMID: 20844536. PMCID: PMC2941915.
39. Engler A, Rolando C, Giachino C, Saotome I, Erni A, Brien C, Zhang R, Zimber-Strobl U, Radtke F, Artavanis-Tsakonas S, Louvi A, Taylor V. 2018; Notch2 signaling maintains NSC quiescence in the murine ventricular-subventricular zone. Cell Rep. 22:992–1002. DOI: 10.1016/j.celrep.2017.12.094. PMID: 29386140.
40. Valvezan AJ, Klein PS. 2012; GSK-3 and Wnt signaling in neurogenesis and bipolar disorder. Front Mol Neurosci. 5:1. DOI: 10.3389/fnmol.2012.00001. PMID: 22319467. PMCID: PMC3268224.
41. Lim DA, Tramontin AD, Trevejo JM, Herrera DG, García-Verdugo JM, Alvarez-Buylla A. 2000; Noggin antagonizes BMP signaling to create a niche for adult neurogenesis. Neuron. 28:713–726. DOI: 10.1016/S0896-6273(00)00148-3. PMID: 11163261.
42. Zhang RR, Cui QY, Murai K, Lim YC, Smith ZD, Jin S, Ye P, Rosa L, Lee YK, Wu HP, Liu W, Xu ZM, Yang L, Ding YQ, Tang F, Meissner A, Ding C, Shi Y, Xu GL. 2013; Tet1 regulates adult hippocampal neurogenesis and cognition. Cell Stem Cell. 13:237–245. DOI: 10.1016/j.stem.2013.05.006. PMID: 23770080. PMCID: PMC4474382.
43. Jobe EM, Gao Y, Eisinger BE, Mladucky JK, Giuliani CC, Kelnhofer LE, Zhao X. 2017; Methyl-CpG-binding protein MBD1 regulates neuronal lineage commitment through maintaining adult neural stem cell identity. J Neurosci. 37:523–536. DOI: 10.1523/JNEUROSCI.1075-16.2016. PMID: 28100736. PMCID: PMC5242405.
44. Liu C, Teng ZQ, Santistevan NJ, Szulwach KE, Guo W, Jin P, Zhao X. 2010; Epigenetic regulation of miR-184 by MBD1 governs neural stem cell proliferation and differentiation. Cell Stem Cell. 6:433–444. DOI: 10.1016/j.stem.2010.02.017. PMID: 20452318. PMCID: PMC2867837.
Fig. 1
Effect of Dnmt inhibitor 5-AzaC on the proliferation of neural stem cells (NSCs). (A) Representative images of neural stem cells cultured in various concentrations of 5-AzaC; scale bars: 200 μm. (B) Cell proliferation assay to compare the rate of cell proliferation in control (DMSO) and 5-AzaC-treated NSCs; Student’s t-test; ***p-value<0.001.
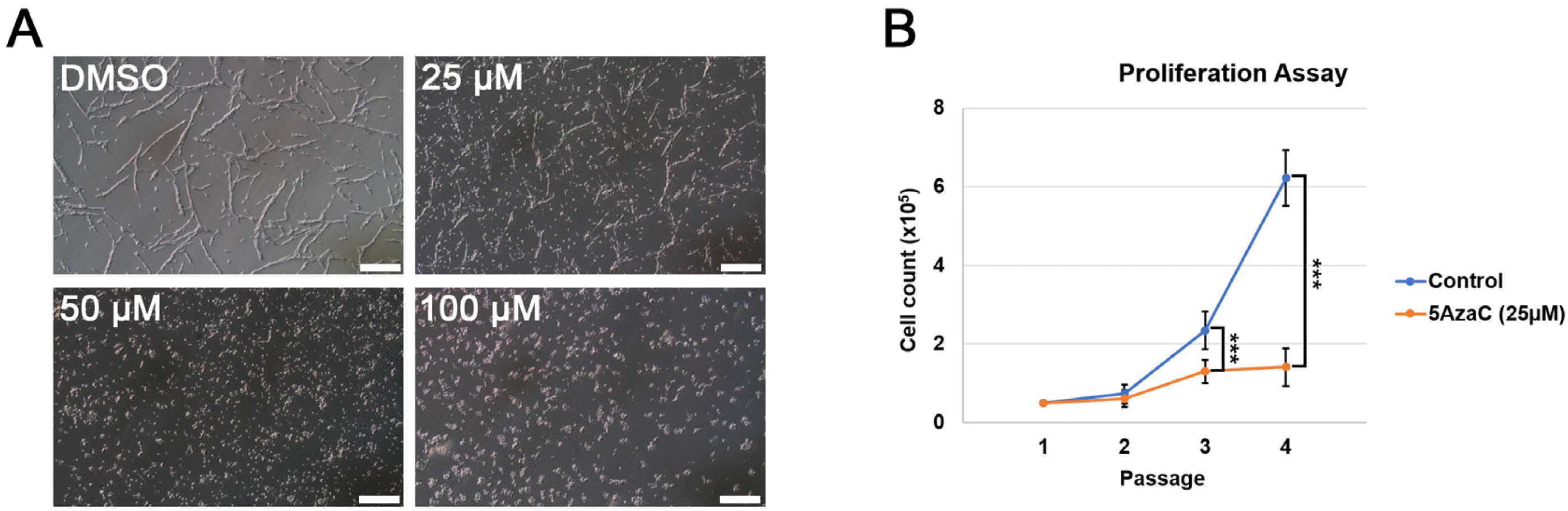
Fig. 2
Pluripotency of three types of Dnmt knockout (KO) ESCs and their differentiation potential into neural rosette and neural stem cells (NSCs). (A) Immunocytochemical analysis of control (Olig2-GFP) and three types of Dnmt KO ESCs, including Dnmt1 KO, Dnmt3a/3b double KO (Dnmt3 DKO), and Dnmt1/3a/3b triple KO (Dnmt TKO). All ESC lines expressed the pluripotency markers Oct4 and Nanog. Nuclei were counter-stained with Hoechst (blue); scale bars: 200 μm. (B) Embryoid body formation of control (Olig2-GFP) and three types of Dnmt KO ESCs; scale bars: 200 μm. (C) Neural rosette formation in control and three types of Dnmt KO ESCs. White arrows indicate neural rosettes, which stained positive for the NSC markers Sox2 and Nestin. Nuclei were counter-stained with Hoechst (blue). Control (Olig2-GFP) ESCs expressed Olig2-GFP, which is expressed in NSCs after commitment from pluripotent stem cells; scale bars: 100 μm. (D) Established NSC lines from control and three types of Dnmt KO ESCs; scale bars: 100 μm.
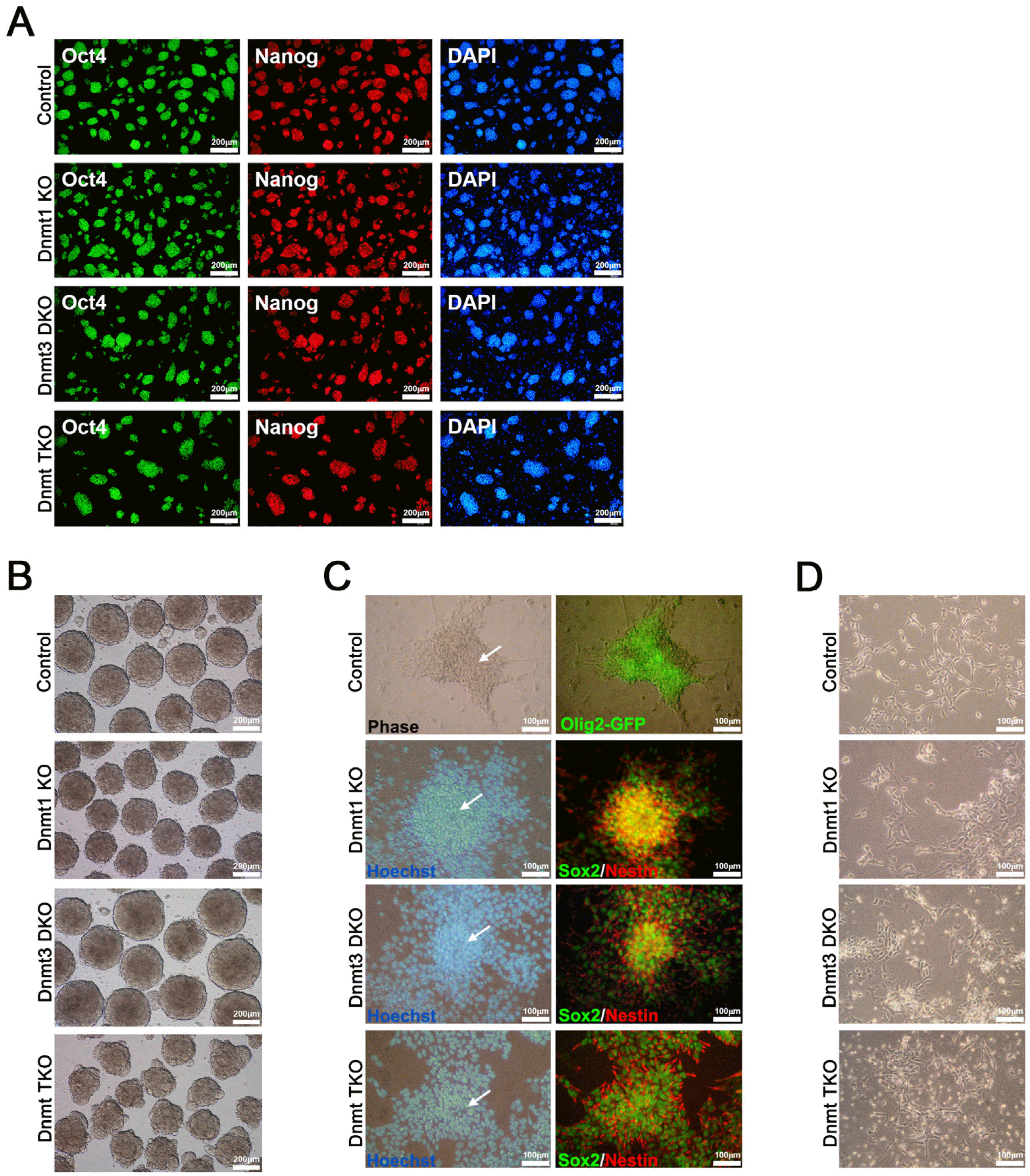
Fig. 3
Immunocytochemical analysis to determine the maintenance of neural stem cells derived from Dnmt knockout (KO) ESCs. After passage three, NSCs from three types of Dnmt KO ESCs, including Dnmt1 KO, Dnmt3a/3b double KO (Dnmt3 DKO), and Dnmt1/3a/3b triple KO (Dnmt TKO), were no longer immunoreactive to the neural stem cell markers Sox2 and Nestin. Control NSCs derived from Olig2-GFP ESCs maintained NSC marker ex-pression. Nuclei were counter-stained with Hoechst (blue); scale bars: 50 μm.
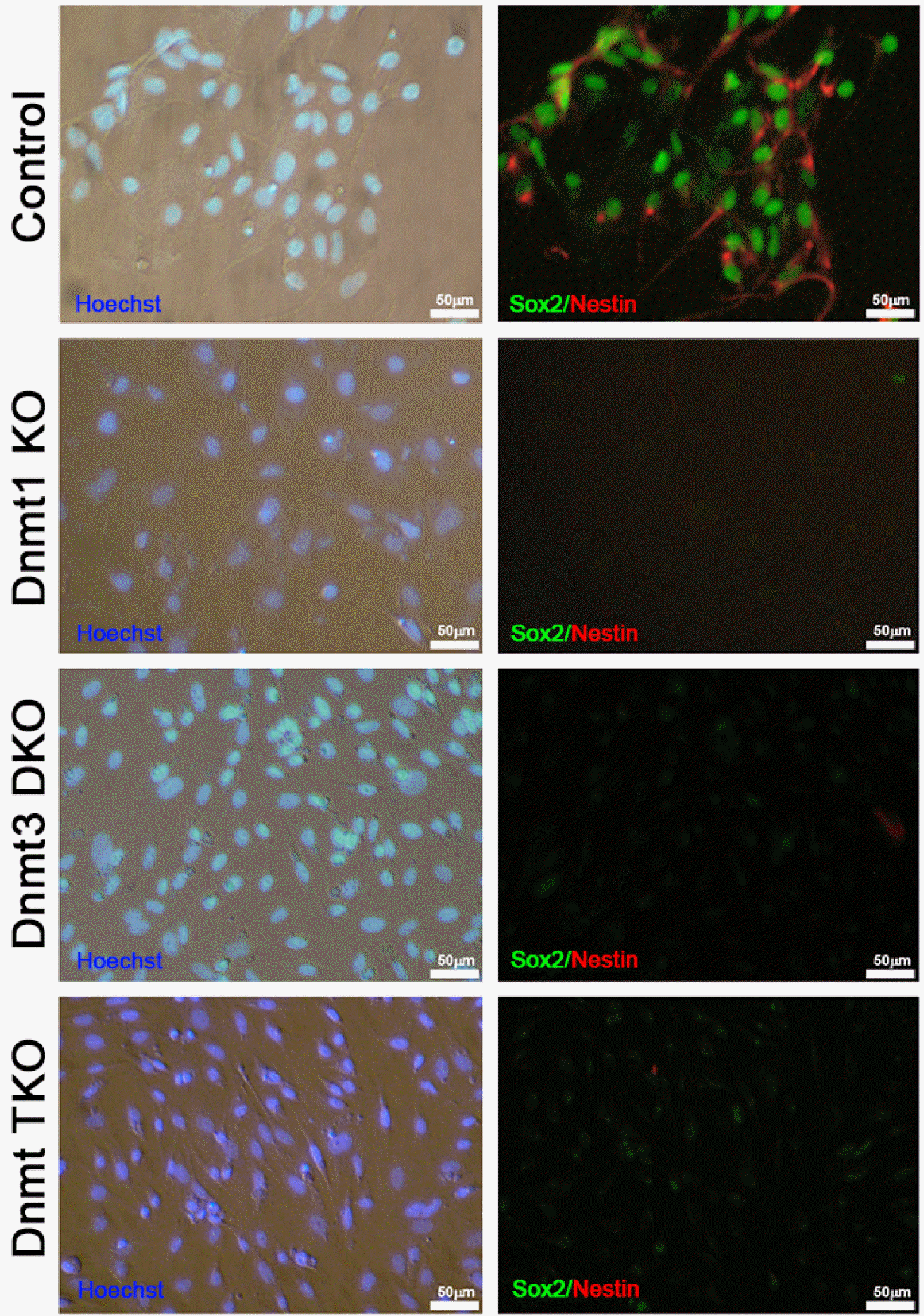
Fig. 4
Differentiation of Dnmt knockout (KO) NSCs into neural subtypes. (A) Immunocytochemical analysis of control (Olig2-GFP) and three types of Dnmt KO NSCs, including Dnmt1 KO, Dnmt3a/3b double KO (Dnmt3 DKO), and Dnmt1/3a/3b triple KO (Dnmt TKO). During the culture, Dnmt KO NSCs spontaneously differentiated into neurons (Tuj1+), astrocytes (GFAP+), and oligodendrocytes (O1+). Nuclei were counter-stained with Hoechst (blue); scale bars: 200 μm for Tuj1 and GFAP, and 100 μm for O1. Control NSCs derived from Olig2-GFP ESCs maintained self-renewal and were not stained by antibodies for Tuj1, GFAP, and O1; scale bar: 200 μm. (B) Representative karyotype of control and three types of Dnmt KO cell lines. Karyotyping revealed no chromosome abnormalities in all groups; scale bar: 5 μm.
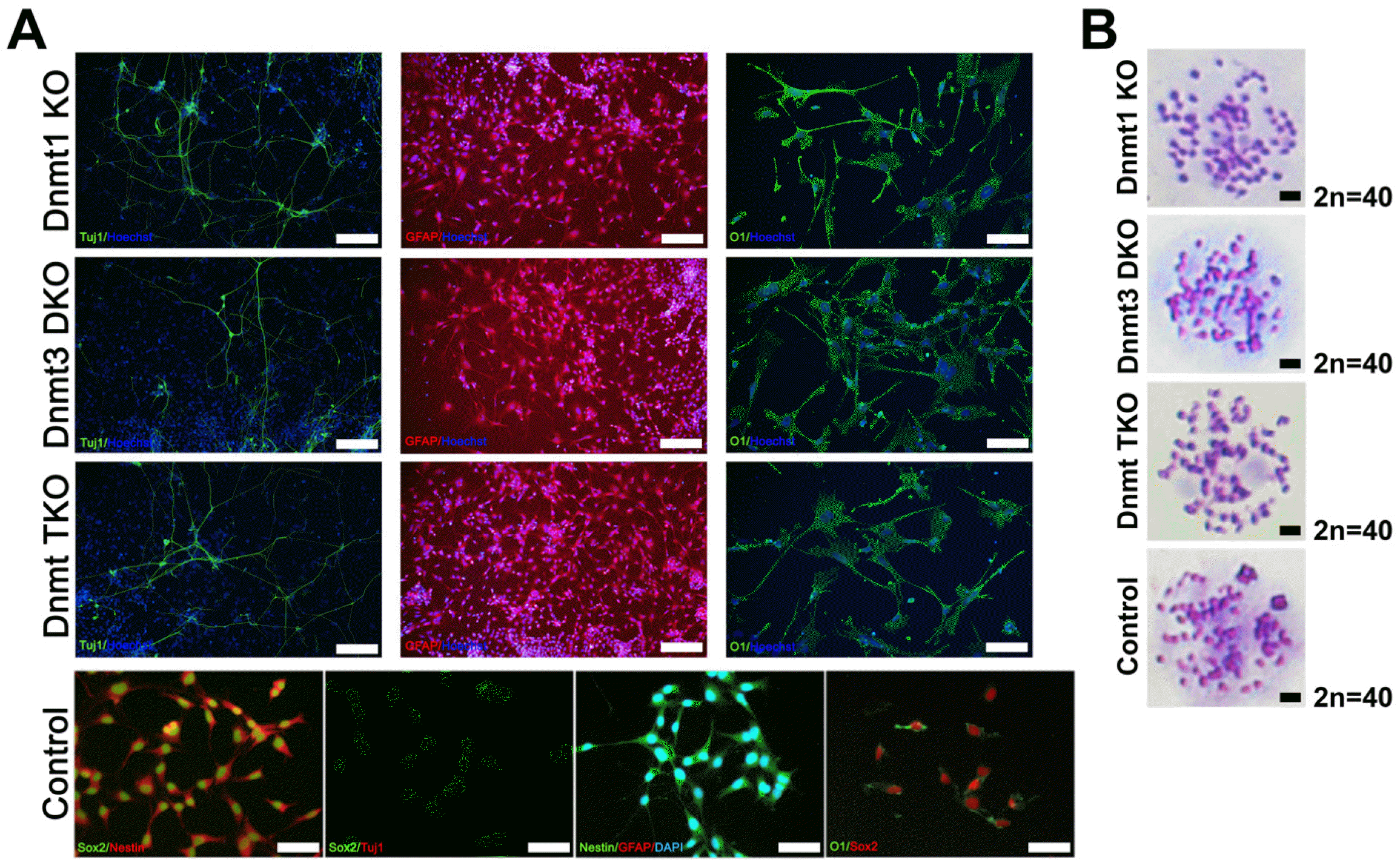