Abstract
Background
In addition to the metabolic effects in diabetes, glucagon-like peptide 1 receptor (GLP-1R) agonists lead to a small but substantial increase in heart rate (HR). However, the GLP-1R actions on the autonomic nervous system (ANS) in diabetes remain debated. Therefore, this meta-analysis evaluates the effect of GLP-1R agonist on measures of ANS function in diabetes.
Methods
According to the Cochrane Collaboration and Preferred Reporting Items for Systematic reviews and Meta-Analyses (PRISMA) statement, we conducted a meta-analysis considering clinical trials in which the autonomic function was evaluated in diabetic subjects chronically treated with GLP-1R agonists. The outcomes were the change of ANS function measured by heart rate variability (HRV) and cardiac autonomic reflex tests (CARTs).
Results
In the studies enrolled, HR significantly increased after treatment (P<0.001), whereas low frequency/high frequency ratio did not differ (P=0.410); no changes in other measures of HRV were detected. Considering CARTs, only the 30:15 value derived from lying-to-standing test was significantly lower after treatment (P=0.002), but only two studies reported this measurement. No differences in other CARTs outcome were observed.
The diabetic autonomic neuropathy is defined as a heterogeneous category of disorders of the autonomic nervous system (ANS) in individuals with either diabetes mellitus or metabolic derangements of pre-diabetes, when other potential causes have been excluded [1]. In particular, the cardiac autonomic neuropathy (CAN) is the manifestation of an ANS imbalance, due to the impairment of autonomic control of the cardiovascular system [1,2]. CAN affects at least 20% of unselected patients, and this incidence raises up to 65% of subjects with either increasing age or long diabetes duration [2-4]. However, the actual CAN prevalence varies, depending upon diagnostic criteria, patient cohort, and testing modality [2,5]. According to standard cardiac autonomic reflex tests (CARTs), the CAN prevalence is reported about 7% in type 2 diabetes mellitus (T2DM) and increases with diabetes duration by 4.6% to 6% per year [2,5]. It is well known that CAN incidence is influenced by diabetic disease duration, patient age, glycaemic control, and concomitant metabolic syndrome features [6,7]. The CAN diagnosis in people with diabetes is extremely relevant, influencing the prognosis for cardiovascular morbidity and predicting the overall cardiovascular risk [8]. Indeed, CAN is related to silent myocardial ischemia, stroke, postural hypotension, exercise intolerance and enhanced intraoperative instability [9]. Therefore, from a clinical perspective, an individual with diabetes and CAN is at higher risk of mortality and of cardiovascular complications with heavy impact on morbidity and prognosis.
Glucagon-like peptide 1 receptor (GLP-1R) agonists represent a relatively new class of anti-hyperglycemic agents, addressing most of the pathophysiological mechanisms involved in the development of T2DM. The main GLP-1R agonist actions are the stimulation of insulin secretion, the inhibition of glucagon secretion, the delay of the gastric emptying time and the stimulation of neogenesis of insulin-secreting cells [10]. Moreover, GLP-1R agonists show favourable effects on body weight and metabolic profile, with a lower risk of hypoglycaemia. Moreover, the favourable metabolic effects, together with a reduction in blood pressure (BP), contribute to reduce the cardiovascular risk. For all these reasons, the GLP1-R agonist use is constantly increasing to treat T2DM.
Among beneficial GLP-1R agonist effects, heart rate (HR) increase has been observed. Despite the resting HR increase could be considered a safety concern [11], GLP-1R agonist administration is associated to major adverse cardiovascular events reduction, including stroke, cardiovascular and allcause mortality, as suggested by the cardiovascular outcome trials (Supplementary Table 1) [12-18]. This GLP-1R agonist favourable effect on cardio-metabolic health is becoming increasingly evident, although the underlying mechanisms remain largely unknown. Improvement of hypertension [19], endothelial function [20] and a natriuretic GLP-1R agonist-related effect [21] have been proposed. However, while a small but substantial increase in HR by about 3 bpm has been reported by the majority of the available trials [22], the action of GLP-1R agonists on autonomic function in diabetes remains still debated. With this in mind, it is clear that the GLP-1R agonist effects on HR and on ANS need to be reconciled with the favourable cardiovascular outcomes in clinical trials. Thus, we performed this meta-analysis with the main aim to highlight the GLP-1R agonist actions on available measures of autonomic function in diabetic people.
This meta-analysis was performed according to the Cochrane Collaboration and Preferred Reporting Items for Systematic reviews and Meta-Analyses (PRISMA) statement. To ensure originality and transparency of the review process, the meta-analysis was a priori registered in the International Prospective Register of Systematic Reviews (PROSPERO; registration number CRD42020218063). The literature search was performed until October 10th, 2021 considering the following string: (((autonomic function)) OR ((autonomic dysfunction)) OR (cardiac autonomic neuropathy) AND ((((((((GLP-1 receptor agonist)) OR (GLP-1R agonist)) OR (semaglutide)) OR (liraglutide)) OR (exenatide)) OR (lixisenatide) OR (dulaglutide)) OR (albiglutide). Medline, Embase, and Cochrane databases were considered.
All available GLP-1R agonists were considered potentially eligible, whether applied in clinical trial aiming at evaluating autonomic function before and after chronic administration in participants with diabetes. Thus, each parameter of autonomic function was considered before and after chronic treatment in the study groups. Moreover, when available, mean parameters were compared between study and control groups after treatment.
CARTs represent the gold standard in autonomic testing [2,23]. CARTs involve measuring autonomic responses through changes in HR and BP to provocative physiological manoeuvres. The standard CARTs recommended for diagnosis of CAN include: the deep breathing (DB) test (expiration/inspiration [E/I] ratio), the lying-to-standing (LS) test (30:15), the Valsalva manoeuvre (VM) and the BP response to standing [2]. E/I index from DB test represents the ratio between the 3 maximum and the 3 minimum RR intervals (the intervals between two consecutive eletrocardiogram R waves) in a cycle of expiration and inspiration. In LS test the maximum/minimum 30:15 value is the ratio of the longest RR interval measured between the 25th and 35th beat after the change of posture and the shortest RR interval measured between the 10th and 20th beat. The VM test indicates the ratio between the longest RR interval after exhalation and the shortest RR interval during exhalation. Other approaches in clinical research are currently available to evaluate CAN such as heart rate variability (HRV), baroreflex sensitivity, muscle sympathetic nerve activity, heart sympathetic imaging [24]. Non-invasive and widely used in clinical research, HRV provides key information about autonomic—parasympathetic and sympathetic—modulation of the cardio-vascular system. The measurement of HRV includes two domains. In the frequency domain, the components of the HRV obtained by spectral analysis consist of low frequency (LF) and high frequency (HF) indices. These indices provide information about both the sympathetic and parasympathetic influences on heart. Thus, the LF/HF ratio is the index of sympathetic-parasympathetic balance. Time domain measures of the normal RR intervals mainly include differences between the longest and the shortest RR intervals, the standard deviations of RR interval (SDNN), and the square root of the mean squared difference of successive RR intervals (RMSSD) [24].
The literature search evaluated all clinical trials with the following inclusion criteria: (1) either interventional or observational; (2) in which the autonomic function was evaluated; (3) in people with diabetes; (4) treated with GLP-1R agonists. In particular, change of ANS function before and after chronic GLP-1R agonist treatment using measures of HRV was considered the main outcome. Treatment was considered chronic when GLP-1R agonist was administered for more than 4 weeks. Both participants with type 1 diabetes mellitus (T1DM) and T2DM were considered eligible. Randomization and presence of controls were not considered as inclusion criteria.
Two authors (C.G. and D.S.) separately performed the literature search, collecting abstracts of each study. Each abstract was evaluated for inclusion criteria and data were extracted from each study considered eligible. C.G. and D.S. performed quality control checks on extracted data. For the literature search, the primary endpoint was the LF/HF ratio after chronic GLP-1R agonist administration. Secondary endpoints extracted were: HR, SDNN, and RSMSSD as measures of HRV and E/I ratio, 30:15, VM as parameters of CARTs.
The risk of bias was assessed independently by two investigators (C.G. and D.S.), using Cochrane risk-of-bias algorithm. In particular, the following criteria were considered for each included trial, (1) randomization method, (2) concealment of allocation, (3) presence or absence of blinding to treatment allocation, (4) presence or absence of blinding of outcome assessment, (5) potential incomplete data biases, and (6) reporting biases.
Using the Review Manager (RevMan) 5.4 Software (version 5.4.1, The Nordic Cochrane Centre, The Cochrane Collaboration, Copenhagen, Denmark; 2014), continuous variables were comprehensively evaluated as inverse variance of mean variables. When data were reported in the original manuscript as median or logarithm, they were transformed in mean±standard deviation. Indeed, mean±standard deviation is required for the meta-analytic approach. However, since these parameters could be obtained using different approaches, the meta-analyses were performed using standard mean difference. Considering that studies included in the meta-analysis reported different treatment durations and different time-points in which endpoints were evaluated, we considered the last available time point for each trial (median 24 weeks; range, 12 to 72). The degree of heterogeneity among the studies included in each analysis was examined by inspecting both the scatter in the data points and the overlap in their confidence intervals (CIs), and by performing I2 statistics. The inverse variance with the fixed model was initially chosen, whereas the random effect model was preferred in case of I2 higher than 60%. Sensitivity analyses were performed, considering the type of diabetes and the GLP-1R agonist used, when possible. Values of P<0.05 were considered statistically significant.
Manuscripts (n=596) were identified by the literature search (Fig. 1). After abstract evaluations, 16 studies were considered for the full text analysis (Fig. 1). Among these, ten studies were excluded (reasons reported in Fig. 1) and six studies were enrolled [25-30] in the final analysis (Fig. 1). Table 1 summarizes the characteristics of the studies included in the meta-analysis. Moreover, Table 2 shows the characteristics of the patient cohorts included in the analysis: a total of 182 individuals with diabetes were enrolled (110 men and 72 women), with a mean age of 54.68±10.7 years. Only two studies enrolled patients with type 1 diabetes mellitus.
The LF/HF ratio did not differ after treatment in the entire sample evaluated (P=0.410), as well as considering exenatide (P=0.290) or liraglutide (P=0.930) treatment, separately (Fig. 2). This lack of LF/HF ratio difference after treatment was maintained also dividing studies according to the type of diabetes considered (standard mean difference, –0.03; 95% CI, –0.26 to 0.20; I2=0%; P=0.080) (Supplementary Fig. 1). Thus, chronic treatment with GLP-1R agonists seems not to affect LF/HF ratio, also after sensitivity analyses. This was further confirmed comparing study and control groups, showing no differences in the LF/HF ratio after treatment (mean difference, 0.18; 95% CI, –0.66 to 1.01; I2=0%; P=0.680) (Supplementary Fig. 2).
HR was reported in all trials, for a total of 182 patients, evaluated before and after chronic treatment. The HR significantly increased after treatment (P<0.001), with a low heterogeneity rate (6%) (Fig. 3). This significant improvement remained considering the use of liraglutide or exenatide separately (Fig. 3). Since Cacciatori et al. [26] did not report a control group, this study was excluded when study and control groups were compared post-treatment. In this analysis, HR was not significantly different between study and control groups (mean difference, –0.86; 95% CI, –3.26 to 1.55; I2=16%; P=0.480) (Supplementary Fig. 3).
After chronic treatment, SDNN did not significantly change (standard mean difference, 0.08; 95% CI, –0.65 to 0.81; P=0.830) (Supplementary Fig. 4). Considering the study-control comparison, significantly lower SDNN values were detected in the study-compared to control groups (P=0.040) (Fig. 4).
RSMSSD was evaluated in six studies, showing no difference after treatment (standard mean difference, 0.57; 95% CI, –0.41 to 1.55; I2=94%; P=0.250) (Supplementary Fig. 5). Similarly, no difference between study and control groups were detected (standard mean difference, –0.38; 95% CI, –1.20 to 0.44; P=0.360) (Supplementary Fig. 6).
The E/I ratio did not change after chronic GLP-1R agonist administration (mean difference, –1.13; 95% CI, –3.64 to 1.38; I2=95%; P=0.380) (Supplementary Fig. 7). In the study vs. control group comparison, the E/I ratio was not significantly different (mean difference, 0.0; 95% CI, –0.004 to 0.004; P=0.999) (Supplementary Fig. 8).
The 30:15 value was significantly lower after treatment (standard mean difference, 0.50; 95% CI, 0.18 to 0.83; P=0.002), although this result was obtained considering only two studies (Fig. 5). Only Hansen et al. [27] reported LS mean difference in the comparison between study and control groups; thus, this analysis was not feasible.
The VM did not significantly change after treatment (mean difference, 0.04; 95% CI, –0.08 to 0.15; P=0.520) (Supplementary Fig. 9).
Finally, the risk of bias was evaluated (Supplementary Fig. 10), showing a good quality of the included studies, in terms of reporting and incomplete biases. On the contrary, a variable quality in terms of blinding was detected, reflecting the difficulty to perform a completely randomized, double-blind clinical trial in this setting.
Here, we confirm that chronic GLP-1R agonist administration increases HR, according to a previously available meta-analysis [22]. Systematically, the HR increase after chronic GLP-1R agonist administration is evident in five of six included trials. Indeed, only one study found no significant changes in HR after GLP-1R agonist administration, but, differently from the others, authors used a short-acting GLP-1R agonist, such as exenatide, for much longer time [28]. Thus, we could speculate that the GLP-1R agonist effect on HR could depend on the molecule used and the duration of the administration. Moreover, together with the HR increase, no significant change in other ANS-related parameters is evident in our meta-analysis. This result suggests that the chronic GLP-1R agonist administration may not influence the sympathetic and parasympathetic functions. Thus, we could speculate that the HR modifications induced by GLP-1R agonist is not consequence of sympathetic or parasympathetic stimulation, but other mechanisms should be involved.
GLP-1R agonists are increasingly used in clinical practice in diabetes, considering the wide range of positive effects on glucose homeostasis, body weight, BP, and the low risk of hypoglycaemia. However, an overall GLP-1R agonist effect on ANS is far from being elucidated, even with the meta-analytic approach. Considering each study separately, interesting results could be extracted. Jaiswal et al. [28] did not detect any change in autonomic function after 18 months of treatment with the short-acting GLP-1R agonist exenatide in patients with T2DM, evaluating either the gold-standard CARTs, such as DB and VM or measures of HRV, such as HR, LF/HF, SDNN, RMSSD. In particular, that study was characterized by different follow-up length (18 months) than other studies included in the analysis (range, 12 to 26 weeks). Therefore, we could not exclude that this difference represents a confounding factor, or a determinant of a kind of mitigation of HR increase over time. On the contrary, Kumarathurai et al. [31] reported detrimental reductions in several HRV indices in T2DM patients treated with liraglutide for 12 weeks. In particular, liraglutide decreased SDNN, RMSSD, and HF without changes in LF/HF ratio compared to placebo [31]. This SDNN decrease persisted despite body weight loss and metabolic parameter improvement, suggesting an impairment in vagal activity after treatment [31]. Moreover, in order to elucidate the role of the cardiac sympatho-vagal balance as a possible mediator of the reported HR increase in GLP-1R agonist treatment, Cacciatori et al. [26] performed CARTs and power spectral analysis of HRV in 28 T2DM patients after exenatide extended-release administration. The main result of this study is an expected HR increase together with an unexpected LF/HF decrease, suggesting the existence of a compensatory mechanism [26]. This phenomenon is characterized by a “shift” of the sympatho-vagal balance with reduction of the LF component and no change in parasympathetic tone (HF) [26]. In contrast, Nystrom et al. [30] demonstrated the absence of changes in sympathetic or parasympathetic activity evaluated by HRV in 62 T2DM individuals receiving 1.8 mg liraglutide once daily in comparison with 4 mg glimepiride once daily. Similarly, in the context of T1DM, changes neither in the HRV domains, nor in cardiac vagal tone and cardiac sensitivity to the baroreflex were observed in 19 patients treated with liraglutide for 26 weeks [25]. This result was confirmed by the post-hoc analysis of Hansen et al. [27], showing no changes in CAN measures after liraglutide 1.8 mg once-daily for 24 weeks. In this study, the E/I ratio declined significantly in both liraglutide and placebo groups.
Accordingly, our findings show no difference in the LF/HF ratio after treatment, considering both different molecules (exenatide and/or liraglutide) and both type of diabetes (T1DM and/or T2DM). Moreover, others HRV measures, such as SDNN and RSMSSD, do not change after chronic GLP-1R agonist administration. These results are confirmed considering liraglutide and exenatide separately, suggesting no differences between molecules. LF/HF ratio represents an index widely used in clinical practice for CAN evaluation in diabetic patients, providing information about autonomic—parasympathetic and sympathetic—modulation of the cardiovascular system. Even if considered among the methods of investigation for cardiac autonomic dysfunction in human research studies [24], LF/HF ratio from HRV study confirms to be a measure not accurate and not directly related to sympatho-vagal balance, according to previous studies [32,33]. Among CARTs measurements, representing the gold standard for the diagnosis of CAN, the only parameter that seems to be influenced by GLP-1R agonist administration is the 30:15 ratio, which decreases after treatment. However, the strength of this result is limited by the small number of trials reporting this parameter. Thus, our meta-analysis suggests that chronic GLP-1R agonist treatment does not influence the sympatho-vagal balance in people with diabetes. Hence, the HR increase could depend on different mechanisms.
In animal models, GLP-1 engages GLP-1R in central, peripheral, and ANSs, enhancing the sympathetic nervous system activity, and reducing the parasympathetic nervous system activity [34]. In this regard, Baggio et al. [34] suggested that the GLP-1R agonist-related HR increase is the final effect of direct chronotropic action, which is attenuated by propanolol but not by atropine. Moreover, the in vivo GLP-1R agonist administration induces c-fos expression—a marker of neuronal activity—in the adrenal medulla, activates neurons involved in autonomic control in the brain, and activates tyrosine hydroxylase transcription in brainstem catecholamine neurons [35]. These findings suggest that the central GLP-1 action could be involved in the regulation of the sympathetic pathway [35]. However, the identification of GLP-1R expression in mouse atria [36] and in monkey sinoatrial node [37] raised the additional possibility that GLP-1 may directly modulate HR through the cardiac GLP-1R. Moreover, Berkelaar et al. [38] evaluated 130 healthy participants undergoing hyperglycaemic clamps and acute exposure to GLP-1 during hyperglycaemia, showing a small acute HR increase without an acute decrease in cardiac vagal control, measured by HRV. In this setting, serum insulin was positively associated with HR [38], suggesting that GLP-1 effect could be mediated by GLP1-driven increase in endogenous insulin [39]. Again, other experimental trials suggested the sympathetic nervous system activation after GLP-1R agonist infusion in healthy individuals [40,41]. All these examples demonstrate that the action of GLP-1R agonist on the sympathetic and parasympathetic systems must be both direct and indirect but this should be further studied with properly designed clinical and experimental trials. Indeed, the present meta-analytic approach is not able to reach strong conclusive results, due to the limited number of studies available. In particular, the scarcity of studies on the topic does not allow to perform further subgroup analyses, representing an important limit intrinsic to the meta-analysis itself. Thus, the lack of significant effects of GLP-1R agonist chronic administration on ANS should be considered in light with the limited amount of data available.
In conclusion, the present meta-analysis confirms the HR increase but seems to exclude an alteration of the sympatho-vagal balance due to chronic treatment with GLP-1R agonists in people with diabetes. Indeed, despite the accumulating data linking GLP-1R signalling to autonomic and neuroendocrine responses, the neural pathways underlying these actions are not fully understood. Furthermore, considering some discrepancies in the available preclinical and clinical findings, it is conceivable to suggest possible species-specific patterns of GLP-1R, as well as differences among GLP-1R agonists. More information is needed on the mechanisms through which the GLP-1R agonists administration may affect autonomic activity in individuals with diabetes.
SUPPLEMENTARY MATERIALS
Supplementary materials related to this article can be found online at https://doi.org/10.4093/dmj.2021.0314.
Supplementary Table 1.
Hazard ratio (95% confidence interval) of the primary composite outcome and secondary outcomes reported in the cardiovascular outcome trials
Supplementary Fig. 1.
Low frequency/high frequency (LF/HF) ratio standard mean difference after the glucagon-like peptide 1 receptor chronic administration, dividing studies on the basis of the type of diabetes considered (standard mean difference, –0.03; 95% confidence interval [CI], –0.26 to 0.20; I2=0%; P=0.080). SD, standard deviation; IV, inverse variance; T1DM, type 1 diabetes mellitus; T2DM, type 2 diabetes mellitus.
Supplementary Fig. 2.
Low frequency/high frequency (LF/HF) ratio ratio difference after the glucagon-like peptide 1 receptor chronic administration comparing study and control groups. Comparing study and control groups, no differences in the LF/HF ratio after treatment were observed (mean difference, 0.18; 95% confidence interval [CI], –0.66 to 1.01; I2=0%; P=0.680). SD, standard deviation; IV, inverse variance.
Supplementary Fig. 3.
Heart rate (HR) mean difference after the glucagon-like peptide 1 receptor chronic administration comparing study and control groups. HR was not significantly different between study and control groups (mean difference, –0.86; 95% confidence interval [CI], –3.26 to 1.55; I2=16%; P=0.480) in five of the six studies. SD, standard deviation; IV, inverse variance.
Supplementary Fig. 4.
Standard deviations of RR interval (SDNN) mean difference considering before and after the glucagonlike peptide 1 receptor cchronic administration. After chronic treatment, SDNN did not significantly change (standard mean difference, 0.08; 95% confidence interval [CI], –0.65 to 0.81; P=0.830). SD, standard deviation; IV, inverse variance.
Supplementary Fig. 5.
Square root of the mean squared difference of successive RR interval (RSMSSD) standard mean difference considering before and after the glucagon-like peptide 1 receptor chronic administration. RSMSSD value documented no difference after treatment (standard mean difference, 0.57; 95% confidence interval [CI], –0.41 to 1.55; I2=94%; P=0.250). SD, standard deviation; IV, inverse variance.
Supplementary Fig. 6.
Square root of the mean squared difference of successive RR interval (RSMSSD) standard mean difference after the glucagon-like peptide 1 receptor chronic administration comparing study and control groups. RSMSSD value documented no difference between study and control groups (standard mean difference, –0.38; 95% confidence interval [CI], –1.20 to 0.44; P=0.360). SD, standard deviation; IV, inverse variance.
Supplementary Fig. 7.
Expiration/inspiration (E/I) ratio mean difference before and after treatment. No changes in E/I ratio after glucagon-like peptide 1 receptor chronic agonist administration (mean difference, –1.13; 95% confidence interval [CI], –3.64 to 1.38; I2=95%; P=0.380). SD, standard deviation; IV, inverse variance.
Supplementary Fig. 8.
Expiration/inspiration (E/I) ratio difference after the glucagon-like peptide 1 receptor chronic administration comparing study and control groups. In the study vs. control group comparison, the E/I ratio was not significantly different (mean difference, 0.0; 95% confidence interval [CI], –0.004 to 0.004; P=0.999). SD, standard deviation; IV, inverse variance.
Supplementary Fig. 9.
Valsalva manoeuvre (VM) mean difference considering before and after the glucagon-like peptide 1 receptor chronic administration. No significantly changes in VM value after treatment (mean difference, 0.04; 95% confidence interval [CI], –0.08 to 0.15; P=0.520). SD, standard deviation; IV, inverse variance.
Supplementary Fig. 10.
Risk of bias graph. Evaluation of the risk of bias showed a good quality of the studies, in terms of reporting and incomplete biases. On the contrary, a variable quality in terms of blinding was detected.
Notes
REFERENCES
1. Tesfaye S, Boulton AJ, Dyck PJ, Freeman R, Horowitz M, Kempler P, et al. Diabetic neuropathies: update on definitions, diagnostic criteria, estimation of severity, and treatments. Diabetes Care. 2010; 33:2285–93.


2. Spallone V, Ziegler D, Freeman R, Bernardi L, Frontoni S, Pop-Busui R, et al. Cardiovascular autonomic neuropathy in diabetes: clinical impact, assessment, diagnosis, and management. Diabetes Metab Res Rev. 2011; 27:639–53.


3. Neil HA, Thompson AV, John S, McCarthy ST, Mann JI. Diabetic autonomic neuropathy: the prevalence of impaired heart rate variability in a geographically defined population. Diabet Med. 1989; 6:20–4.


4. Ziegler D, Gries FA, Spuler M, Lessmann F. The epidemiology of diabetic neuropathy. DiaCAN Multicenter Study Group. Diabet Med. 1993; 10 Suppl 2:82S–86S.


5. Zoppini G, Cacciatori V, Raimondo D, Gemma M, Trombetta M, Dauriz M, et al. Prevalence of cardiovascular autonomic neuropathy in a cohort of patients with newly diagnosed type 2 diabetes: the Verona Newly Diagnosed Type 2 Diabetes Study (VNDS). Diabetes Care. 2015; 38:1487–93.


6. Williams SM, Eleftheriadou A, Alam U, Cuthbertson DJ, Wilding JP. Correction to: Cardiac autonomic neuropathy in obesity, metabolic syndrome and prediabetes: a narrative review. Diabetes Ther. 2019; 10:2023.


7. Spallone V. Update on the impact, diagnosis and management of cardiovascular autonomic neuropathy in diabetes: what is defined, what is new, and what is unmet. Diabetes Metab J. 2019; 43:3–30.


8. Pop-Busui R, Boulton AJ, Feldman EL, Bril V, Freeman R, Malik RA, et al. Diabetic neuropathy: a position statement by the American Diabetes Association. Diabetes Care. 2017; 40:136–54.


9. Vinik AI, Ziegler D. Diabetic cardiovascular autonomic neuropathy. Circulation. 2007; 115:387–97.


10. Drucker DJ. Mechanisms of action and therapeutic application of glucagon-like peptide-1. Cell Metab. 2018; 27:740–56.


11. Cooney MT, Vartiainen E, Laatikainen T, Juolevi A, Dudina A, Graham IM. Elevated resting heart rate is an independent risk factor for cardiovascular disease in healthy men and women. Am Heart J. 2010; 159:612–9.


12. Marso SP, Daniels GH, Brown-Frandsen K, Kristensen P, Mann JF, Nauck MA, et al. Liraglutide and cardiovascular outcomes in type 2 diabetes. N Engl J Med. 2016; 375:311–22.
13. Pfeffer MA, Claggett B, Diaz R, Dickstein K, Gerstein HC, Kober LV, et al. Lixisenatide in patients with type 2 diabetes and acute coronary syndrome. N Engl J Med. 2015; 373:2247–57.


14. Marso SP, Bain SC, Consoli A, Eliaschewitz FG, Jodar E, Leiter LA, et al. Semaglutide and cardiovascular outcomes in patients with type 2 diabetes. N Engl J Med. 2016; 375:1834–44.


15. Holman RR, Bethel MA, Mentz RJ, Thompson VP, Lokhnygina Y, Buse JB, et al. Effects of once-weekly exenatide on cardiovascular outcomes in type 2 diabetes. N Engl J Med. 2017; 377:1228–39.


16. Hernandez AF, Green JB, Janmohamed S, D’Agostino RB Sr, Granger CB, Jones NP, et al. Albiglutide and cardiovascular outcomes in patients with type 2 diabetes and cardiovascular disease (Harmony Outcomes): a double-blind, randomised placebo-controlled trial. Lancet. 2018; 392:1519–29.
17. Gerstein HC, Colhoun HM, Dagenais GR, Diaz R, Lakshmanan M, Pais P, et al. Dulaglutide and cardiovascular outcomes in type 2 diabetes (REWIND): a double-blind, randomised placebo-controlled trial. Lancet. 2019; 394:121–30.
18. Husain M, Birkenfeld AL, Donsmark M, Dungan K, Eliaschewitz FG, Franco DR, et al. Oral semaglutide and cardiovascular outcomes in patients with type 2 diabetes. N Engl J Med. 2019; 381:841–51.


19. Katout M, Zhu H, Rutsky J, Shah P, Brook RD, Zhong J, et al. Effect of GLP-1 mimetics on blood pressure and relationship to weight loss and glycemia lowering: results of a systematic meta-analysis and meta-regression. Am J Hypertens. 2014; 27:130–9.


20. Nystrom T, Gutniak MK, Zhang Q, Zhang F, Holst JJ, Ahren B, et al. Effects of glucagon-like peptide-1 on endothelial function in type 2 diabetes patients with stable coronary artery disease. Am J Physiol Endocrinol Metab. 2004; 287:E1209–15.
21. Gutzwiller JP, Tschopp S, Bock A, Zehnder CE, Huber AR, Kreyenbuehl M, et al. Glucagon-like peptide 1 induces natriuresis in healthy subjects and in insulin-resistant obese men. J Clin Endocrinol Metab. 2004; 89:3055–61.


22. Sun F, Wu S, Guo S, Yu K, Yang Z, Li L, et al. Impact of GLP-1 receptor agonists on blood pressure, heart rate and hypertension among patients with type 2 diabetes: a systematic review and network meta-analysis. Diabetes Res Clin Pract. 2015; 110:26–37.


23. England JD, Gronseth GS, Franklin G, Carter GT, Kinsella LJ, Cohen JA, et al. Practice parameter: evaluation of distal symmetric polyneuropathy: role of autonomic testing, nerve biopsy, and skin biopsy (an evidence-based review): report of the American Academy of Neurology, American Association of Neuromuscular and Electrodiagnostic Medicine, and American Academy of Physical Medicine and Rehabilitation. Neurology. 2009; 72:177–84.


24. Bernardi L, Spallone V, Stevens M, Hilsted J, Frontoni S, PopBusui R, et al. Methods of investigation for cardiac autonomic dysfunction in human research studies. Diabetes Metab Res Rev. 2011; 27:654–64.
25. Brock C, Hansen CS, Karmisholt J, Moller HJ, Juhl A, Farmer AD, et al. Liraglutide treatment reduced interleukin-6 in adults with type 1 diabetes but did not improve established autonomic or polyneuropathy. Br J Clin Pharmacol. 2019; 85:2512–23.


26. Cacciatori V, Zoppini G, Bellavere F, Rigolon R, Thomaseth K, Pichiri I, et al. Long-acting GLP-1 receptor agonist exenatide influence on the autonomic cardiac sympatho-vagal balance. J Endocr Soc. 2017; 2:53–62.


27. Hansen CS, Frandsen CS, Fleischer J, Vistisen D, Holst JJ, Tarnow L, et al. Liraglutide-induced weight loss may be affected by autonomic regulation in type 1 diabetes. Front Endocrinol (Lausanne). 2019; 10:242.


28. Jaiswal M, Martin CL, Brown MB, Callaghan B, Albers JW, Feldman EL, et al. Effects of exenatide on measures of diabetic neuropathy in subjects with type 2 diabetes: results from an 18-month proof-of-concept open-label randomized study. J Diabetes Complications. 2015; 29:1287–94.


29. Kumarathurai P, Anholm C, Fabricius-Bjerre A, Nielsen OW, Kristiansen O, Madsbad S, et al. Effects of the glucagon-like peptide-1 receptor agonist liraglutide on 24-h ambulatory blood pressure in patients with type 2 diabetes and stable coronary artery disease: a randomized, double-blind, placebo-controlled, crossover study. J Hypertens. 2017; 35:1070–8.
30. Nystrom T, Santos-Pardo I, Fang X, Cao Y, Hedberg F, Jendle J. Heart rate variability in type 2 diabetic subjects randomized to liraglutide or glimepiride treatment, both in combination with metformin: a randomized, open, parallel-group study. Endocrinol Diabetes Metab. 2019; 2:e00058.
31. Kumarathurai P, Anholm C, Larsen BS, Olsen RH, Madsbad S, Kristiansen O, et al. Effects of liraglutide on heart rate and heart rate variability: a randomized, double-blind, placebocontrolled crossover study. Diabetes Care. 2017; 40:117–24.
32. Goldstein DS, Bentho O, Park MY, Sharabi Y. Low-frequency power of heart rate variability is not a measure of cardiac sympathetic tone but may be a measure of modulation of cardiac autonomic outflows by baroreflexes. Exp Physiol. 2011; 96:1255–61.


33. Billman GE. The LF/HF ratio does not accurately measure cardiac sympatho-vagal balance. Front Physiol. 2013; 4:26.


34. Baggio LL, Ussher JR, McLean BA, Cao X, Kabir MG, Mulvihill EE, et al. The autonomic nervous system and cardiac GLP1 receptors control heart rate in mice. Mol Metab. 2017; 6:1339–49.


35. Yamamoto H, Lee CE, Marcus JN, Williams TD, Overton JM, Lopez ME, et al. Glucagon-like peptide-1 receptor stimulation increases blood pressure and heart rate and activates autonomic regulatory neurons. J Clin Invest. 2002; 110:43–52.


36. Richards P, Parker HE, Adriaenssens AE, Hodgson JM, Cork SC, Trapp S, et al. Identification and characterization of GLP-1 receptor-expressing cells using a new transgenic mouse model. Diabetes. 2014; 63:1224–33.


37. Pyke C, Heller RS, Kirk RK, Orskov C, Reedtz-Runge S, Kaastrup P, et al. GLP-1 receptor localization in monkey and human tissue: novel distribution revealed with extensively validated monoclonal antibody. Endocrinology. 2014; 155:1280–90.
38. Berkelaar M, Eekhoff EM, Simonis-Bik AM, Boomsma DI, Diamant M, Ijzerman RG, et al. Effects of induced hyperinsulinaemia with and without hyperglycaemia on measures of cardiac vagal control. Diabetologia. 2013; 56:1436–43.


39. Valensi P, Chiheb S, Fysekidis M. Insulin- and glucagon-like peptide-1-induced changes in heart rate and vagosympathetic activity: why they matter. Diabetologia. 2013; 56:1196–200.


Fig. 2.
Low frequency/high frequency (LF/HF) ratio mean difference after the chronic administration of glucagon-like peptide 1 receptor agonists. LF/HF ratio mean difference after treatment in the entire sample (P=0.410) and considering only exenatide (P=0.290) or only liraglutide (P=0.930) treatment. SD, standard deviation; IV, inverse variance; CI, confidence interval.
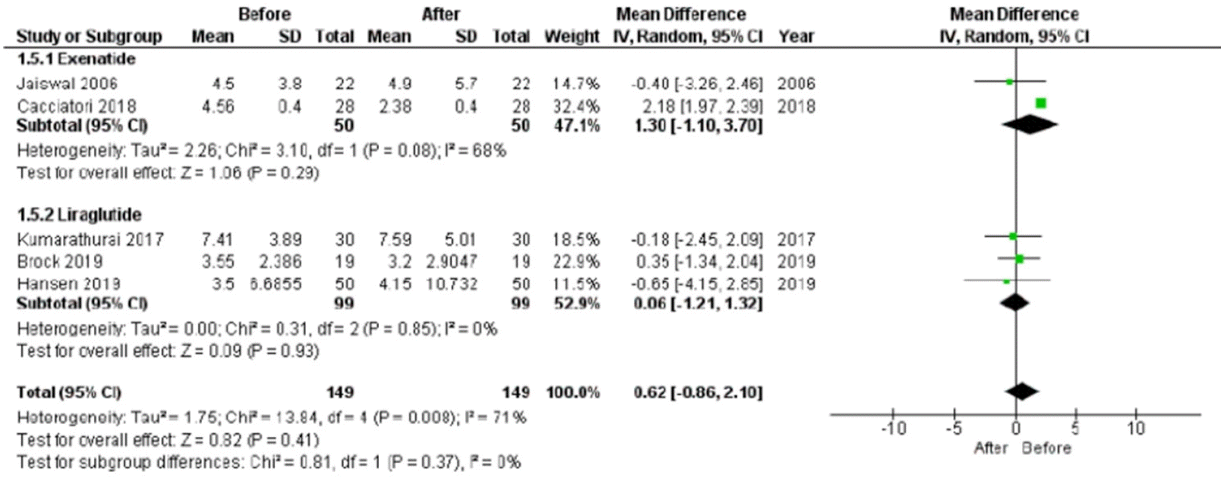
Fig. 3.
Heart rate (HR) mean difference before and after treatment. HR significantly increases after treatment (P<0.001), with a low heterogeneity rate (6%). SD, standard deviation; IV, inverse variance; CI, confidence interval.
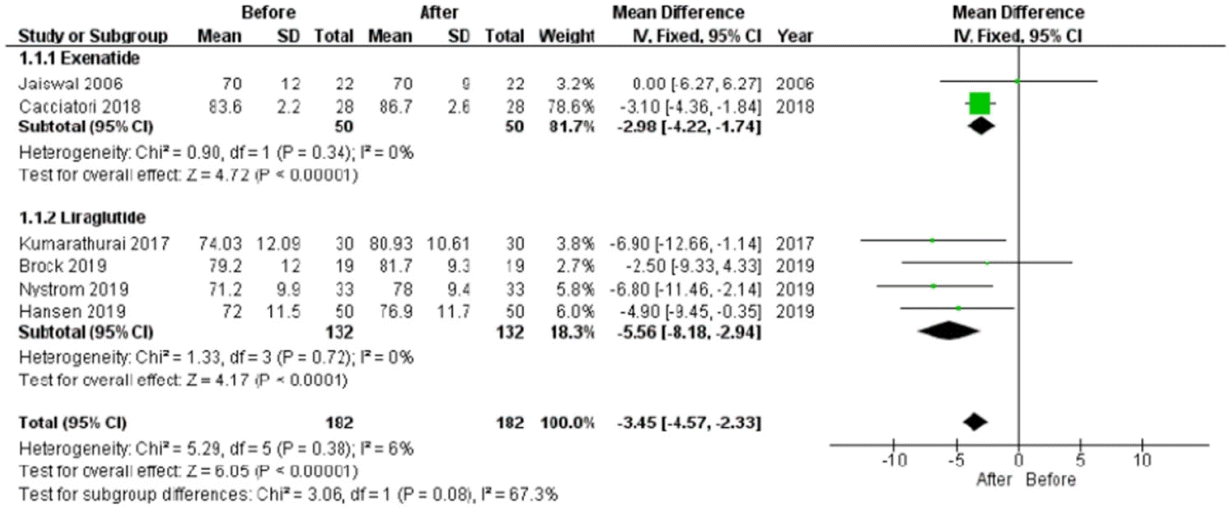
Fig. 4.
Standard deviations of RR interval (SDNN) mean difference after the glucagon-like peptide 1 receptor chronic administration comparing study and control groups. Significantly lower SDNN values were detected in the study-compared to control groups (P=0.040). SD, standard deviation; IV, inverse variance; CI, confidence interval.
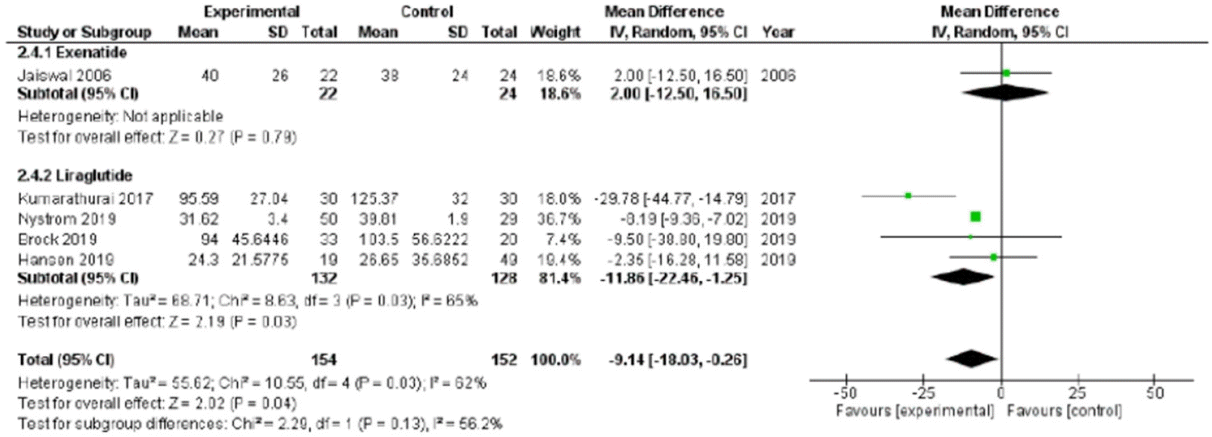
Fig. 5.
A 30:15 value standard mean difference after chronic glucagon-like peptide 1 receptor agonists treatment. SD, standard deviation; IV, inverse variance; CI, confidence interval.
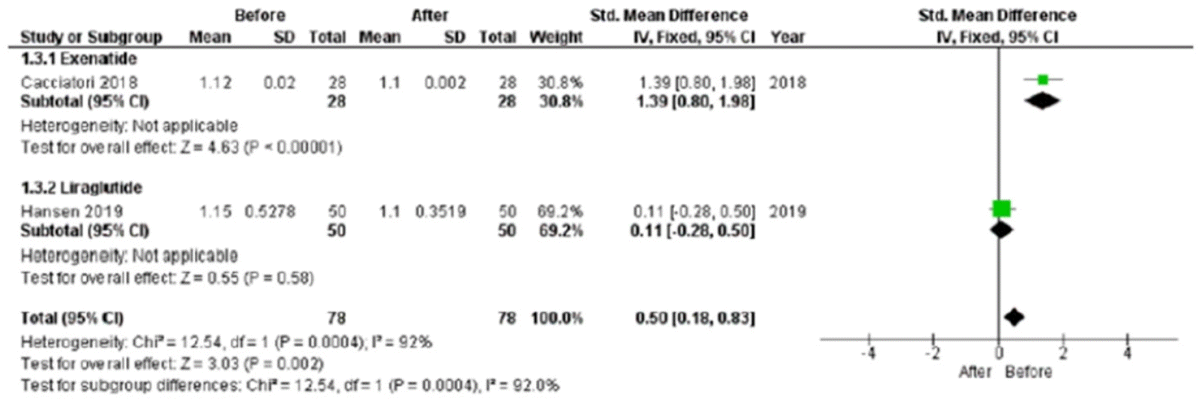
Table 1.
General characteristics of the six included studies
Study | Study design | Subjects | Treatment | Control | Follow-up | Outcome | Results |
---|---|---|---|---|---|---|---|
Jaiswal et al. (2015) [28] | Open-label randomized, controlled | 46 T2DM | Daily exenatide | Glargine | 12 and 18 months | CARTs: DB and VM test | CARTs: no changes E/I, VM |
HRV: HR, LF/HF, SDNN, RMSSD | HRV: no changes HR, LH/HF, SDNN, and RMSSD | ||||||
Kumarathurai et al. (2017) [31] | Double-blind placebo controlled | 30 T2DM | Liraglutide | Placebo | 12 weeks | HRV: HR, LF/HF, SDNN, RMSSD | ↓SDNN, ↓RMSSD |
↑HR, ↓HF, = LF/HF | |||||||
Cacciatori et al. (2017) [26] | Observational study | 28 T2DM | Exenatide ER | No control | 3 and 6 months | HRV (lying and standing positions): HR, LF/HF | HRV: ↑HR; ↓LF; = HF; ↓LF/HF |
CARTs: LS, DB, and VM | CARTs: no change LS, DB, and VM | ||||||
Nystrom et al. (2019) [30] | Open-label randomized, controlled | 62 T2DM | Liraglutide | Glimepiride | 18 weeks | HRV: HR, LF/HF, SDNN, RMSSD | ↑HR; no changes LF, HF, SDNN, and RMSSD |
Brock et al. (2019) [25] | Double-blind placebo controlled | 39 T1DM | Liraglutide | Placebo | 26 weeks | HRV: HR, LF/HF, SDNN, RMSSD | No changes HR, LF, HF, SDNN, and RMSSD |
Hansen et al. (2019) [27] | Double-blind placebo controlled | 99 T1DM | Liraglutide | Placebo | 24 weeks | HRV: LF/HF, SDNN, RMSSD | ↑HR; no changes SDNN, RMSSD, HF, LF, HF/LF ratio |
CARTs: LS, DB, and VM test | No changes E/I, 30:15 and VM |
T2DM, type 2 diabetes mellitus; CART, cardiovascular reflex test; DB, deep breathing; VM, Valsalva manoeuvre; HRV, heart rate variability; HR, heart rate; LF, low frequency; HF, high frequency; SDNN, standard deviation of beat-to-beat (NN) interval; RMSSD, root mean square of successive difference; E/I, expiration/inspiration; ER, extended release; LS, lying-to-standing; T1DM, type 1 diabetes mellitus.
Table 2.
General characteristics of the cohorts included in the analysis
Study | Type of diabetes | No. of subjects | Sex | Age (mean±SD), yr | Molecule | Dosage | Treatment duration |
---|---|---|---|---|---|---|---|
Jaiswal et al. (2015) [28] | T2DM | 22 | 13M, 9F | 51±13 | Exenatide | 10 μg twice daily | 18 months |
Kumarathurai et al. (2017) [31] | T2DM | 30 | ND | ND | Liraglutide | 1.8 mg daily | 12 weeks |
Cacciatori et al. (2017) [26] | T2DM | 28 | 13M, 15F | 62.9±9.6 | Exenatide ER | 2 mg once weekly | 24 weeks |
Nystrom et al. (2019) [30] | T2DM | 33 | 24M, 9F | 60.8±7.6 | Liraglutide | 1.8 mg daily | 18 weeks |
Brock et al. (2019) [25] | T1DM | 19 | 17M, 2F | 51±10 | Liraglutide | 1.2–1.8 mg daily | 26 weeks |
Hansen et al. (2019) [27] | T1DM | 50 | 30M, 20F | 47.7±13.3 | Liraglutide | 1.8 mg daily | 24 weeks |