INTRODUCTION

MATERIALS AND METHODS
Chemicals
Mice and diets
![]() | Fig. 1Experimental design. The mice were divided into 3 groups: Ct group, BD group, Re group. The BD group mice were fed a BD diet. The recovery group mice were fed a BD diet until day 21 and a biotin-containing diet from day 22 to day 64. Some mice from each group were euthanized on days 22, 43, or 64.Ct group, control group; BD group, biotin deficient group; Re group, recovery group.
|
Tissue and plasma collection
Oocyte collection
Nile red staining of lipid droplets in oocytes
Mitochondrial membrane-potential assay
Immunofluorescence
Expression of genes in ovaries
Table 1
List of primers
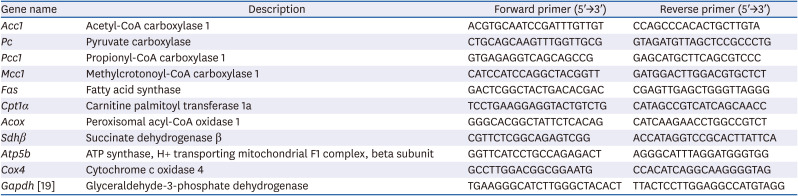
Gene name | Description | Forward primer (5′→3′) | Reverse primer (5′→3′) |
---|---|---|---|
Acc1 | Acetyl-CoA carboxylase 1 | ACGTGCAATCCGATTTGTTGT | CCAGCCCACACTGCTTGTA |
Pc | Pyruvate carboxylase | CTGCAGCAAGTTTGGTTGCG | GTAGATGTTAGCTCCGCCCTG |
Pcc1 | Propionyl-CoA carboxylase 1 | GTGAGAGGTCAGCAGCCG | GAGCATGCTTCAGCGTCCC |
Mcc1 | Methylcrotonoyl-CoA carboxylase 1 | CATCCATCCAGGCTACGGTT | GATGGACTTGGACGTGCTCT |
Fas | Fatty acid synthase | GACTCGGCTACTGACACGAC | CGAGTTGAGCTGGGTTAGGG |
Cpt1α | Carnitine palmitoyl transferase 1a | TCCTGAAGGAGGTACTGTCTG | CATAGCCGTCATCAGCAACC |
Acox | Peroxisomal acyl-CoA oxidase 1 | GGGCACGGCTATTCTCACAG | CATCAAGAACCTGGCCGTCT |
Sdhβ | Succinate dehydrogenase β | CGTTCTCGGCAGAGTCGG | ACCATAGGTCCGCACTTATTCA |
Atp5b | ATP synthase, H+ transporting mitochondrial F1 complex, beta subunit | GGTTCATCCTGCCAGAGACT | AGGGCATTTAGGATGGGTGG |
Cox4 | Cytochrome c oxidase 4 | GCCTTGGACGGCGGAATG | CCACATCAGGCAAGGGGTAG |
Gapdh [19] | Glyceraldehyde-3-phosphate dehydrogenase | TGAAGGGCATCTTGGGCTACACT | TTACTCCTTGGAGGCCATGTAGG |
Western blotting
Measurement of biotin in urine and liver samples
Serum TG analyses
Statistical analyses

RESULTS
Bodyweight, food intake, tissue weight, and biotin nutritional status
Table 2
Effect of biotin-deficiency and biotin re-feeding on the food intake, tissue weight, oocyte ovulation, and biotin nutritional status
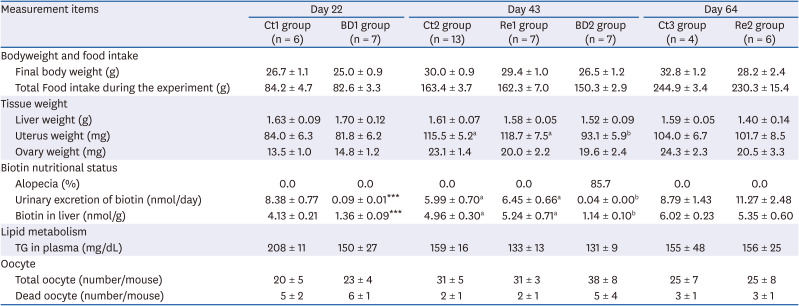
Effect of biotin deficiency and re-feeding on the TG level of plasma
Effect of biotin deficiency and re-feeding on the number of ovulated oocytes
Effect of biotin deficiency and re-feeding on the lipid droplets in oocytes
![]() | Fig. 2Lipid droplet levels in oocytes on days 22, 43, and 64. The oocytes were stained with Nile Red, and the lipid droplets in oocytes were detected using confocal fluorescence microscopy on days 22 (A), 43 (B), and 64 (C). Dots of orange are lipid droplets in the oocyte (right panels). The bar graphs show the fluorescence intensity of oocytes in the Ct group (blue bar), the BD group (white bar), and the Re group (light blue bar). Ct1, 2 and 3 group, n = 32, 82, and 16, on days 22, 43, and 64, respectively; BD1 and 2 group, n = 39 and 57 on days 22 and 43, respectively; Re1 and 2 group, n = 62 and 27 on days 43 and 64, respectively. Values are means ± SE. The data on days 22 and 64 were analyzed using the Student's t-test. The data on day 43 were analyzed by 1-way analysis of variance. A different letter means a significant difference at P < 0.05.Ct group, control group; BD group, biotin deficient group; Re group, recovery group.
*P < 0.05, ***P < 0.001.
|
Effect of a biotin deficiency and re-feeding on the mitochondrial function in oocytes
![]() | Fig. 3Mitochondrial membrane potential in oocytes on days 22, 43, and 64. The oocytes from each group were stained with JC-1 (A). The mitochondrial membrane potential was calculated as the ratio of red fluorescence (corresponding to active mitochondria) to green fluorescence (corresponding to mitochondria with lower activity), on days 22 (B), 43 (C), and 64 (D). The bar graphs show the fluorescence intensity of oocytes in the Ct group (blue bar), the BD group (white bar), and the Re group (light blue bar). C1,2 and 3group, n = 19, 67, and 13 on days 22, 43, 64, respectively; BD1 and 2 group, n = 28 and 36 on days 22 and 43, respectively; Re 11 and 2 group, n = 33 and 25 on days 43 and 64, respectively. The values are the means ± SE. The data on days 22 and 64 were analyzed using the Student's t-test. The data on day 43 were analyzed by 1-way analysis of variance. A different letter means a significant difference at P < 0.05.Ct group, control group; BD group, biotin deficient group; Re group, recovery group.
*P < 0.05, **P < 0.01.
|
Effect of a biotin deficiency and re-feeding on the phosphorylation of ACC in ovaries
![]() | Fig. 4p-ACC levels in ovaries. ACC, p-ACC, and GAPDH protein expression in the ovaries were analyzed by Western blotting (A). The data intensity of each band was quantified using ImageJ software, and the level of expression of p-ACC was normalized to the ACC level (B). The bar graphs show the p-ACC/ACC rate in the ovaries of mice in the Ct group (blue bar), the BD group (white bar), and the Re group (light blue bar). The values are the means ± SE, n = 3 to 4/group. The data on days 22 and 64 were analyzed using the Student's t-test. The data on day 43 were analyzed using 1-way analysis of variance. A different letter means a significant difference at P < 0.05.p-ACC, phosphorylated ACC; ACC, acetyl CoA carboxylase; GAPDH, glyceraldehyde-3-phosphate dehydrogenase; Ct group, control group; BD group, biotin deficient group; Re group, recovery group.
|
Effect on biotin coenzyme gene expression in ovaries
![]() | Fig. 5Gene expression related to biotin-dependent enzymes, lipogenesis metabolism, and energy metabolism in the ovaries on days 22, 43, and 64. The mRNA levels of Acc1, Pc, Pcc1, and Mcc1 (A-D) were analyzed as the biotin-dependent enzymes, mRNAs of Fas (E) as a marker for lipogenesis, of Cpt1α (F) and Acox1 (G) for fatty acid β-oxidation, and Shdβ, Cox4, and Atp5b (H-J) for the energy metabolism. The bar graphs show the gene expression levels in the ovaries of the mice in the Ct group (blue bar), the BD group (white bar), and the Re group (light blue bar). The values are the means ± SE, n = 3 to 4/group. The data on days 22 and 64 were analyzed using the Student's t-test. The data on day 43 were analyzed by 1-way analysis of variance. A different letter means a significant difference at P < 0.05.mRNA, messenger RNA; Acc1, acetyl-CoA carboxylase 1; Pc, pyruvate carboxylase; Pcc1, propionyl-CoA carboxylase 1; Mcc1, β-methylcrotonyl-CoA carboxylase 1; Fas, fatty acid synthase; Cpt1α, carnitine palmitoyl transferase 1α; Acox1, peroxisomal acyl-CoA oxidase 1; Sdhβ, succinate dehydrogenase β; Cox4, cytochrome c oxidase 4; Ct group, control group; BD group, biotin deficient group; Re group, recovery group.
**P < 0.01.
|
Effect on lipid metabolism gene expression in the ovaries
Effect on energy metabolism gene expression in ovaries
Effect of long-term re-feeding of biotin on oocyte quality
![]() | Fig. 6Frequency of abnormal oocytes on day 64. Tubulin and chromosomes were stained using an immunofluorescence stain and propidium iodide. The oocytes were classified as normal oocytes or abnormal oocytes (the latter includes those oocytes with spindle defects, chromosomal misalignments, or were immature) (A). The percentage of abnormal oocytes was calculated by division (number of abnormal oocytes/number of total oocytes × 100) (B). The bar graphs show the percentage of abnormal oocytes in mice of the Ct group (blue bar) and the Re group (light blue bar). Ct3 group, n = 42; Re2 group, n = 51. The oocytes were collected from 3 to 4 mice in each group. Data were analyzed using the χ2 test.Ct group, control group; Re group, recovery group.
**P < 0.01.
|

DISCUSSION
