Abstract
Background
In the osseointegration of dental implants, the implant surface properties have been reported to be some of the most important critical factors. The effect of implant’s surfaces created by resorbable blast media (RBM) followed by laser ablation on bone tissue reactions was examined using the removal torque test and histomorphometric analysis.
Methods
Two types of dental implants, RBM-laser implants (experimental group) and RBM implants (control group) (CSM implant system, Daegu, Korea; L=6 mm, diameter=3.75 mm) were placed into the right and left distal femoral metaphysis of 17 adult rabbits. Six weeks after placement, removal torque was measured and histomorphometric analysis was performed.
Results
The mean removal torque was 24.0±10.2 Ncm and 46.6±16.4 Ncm for the control and test specimens, respectively. The experimental RBM-laser implants had significantly higher removal torque values than the control RBM implants (p=0.013). The mean values of total and cortical bone to implant contact (BIC) were respectively 46.3±10.8% and 65.3±12.5% for the experimental group, and 41.9±18.5% and 57.6±10.6% for the control group. The experimental RBM-laser implants showed a higher degree of total and cortical BIC compared with RBM implants, but there was no statistical significance (p=0.482, 0.225).
Osseointegrated dental implants have been widely accepted as the method for the functional and esthetical restoration of missing teeth. Their successful long-term stability has been reported in a large number of clinical studies [1-4]. The following factors are critical to the success of dental implant treatments: the mechanical properties of the implant material, the implant design, the implant surface configuration, the quality of the host tissue, the surgical technique, and the loading pattern [5]. Implant surfaces treated with resorbable blast media (RBM) have a higher removal torque and superior interfacial bone contact than machined implants, providing more benefits on early bone formation and initial stability [6-9]. Laser processing has been reported to be a new method for treating implant surfaces to produce a high degree of purity with sufficient roughness for good osseointegration [10].
The purpose of this study was to evaluate the synergistic potential of implant surfaces created by RBM treatment followed by laser ablation (RBM-laser implants) on bone tissue reactions compared to routine RBM surface-treated implants (RBM implants).
Thirty-four commercially pure titanium (Ti) dental implants (CSM implant system, Daegu, Korea) were used. The implants were 6 mm long with an outer diameter of 3.75 mm.
First, the 34 implants, which included the control group (17 implants), were blasted with 10 µm hydroxyapatite (HA) powder using an Index Type Auto Blast M/C (Korea Shot Blast Co., Ltd, Siheung, Korea). Thereafter, only 17 implants, the experimental group, were modified by laser ablation. A Nd: YAG laser (JENOPTIK Laser Optik Systeme GmbH, Jena, Germany), 15 kHz laser beam (10 W, 2 μsec in pulse width) was used.
Thirty-four implants (L=6 mm; d=3.75 mm) were placed in the femurs of the rabbits (17 animals) and evaluated after 6 weeks of healing. As the experimental and the control groups, each implants were placed respectively in the right and the left femurs of the same rabbits.
Permission was obtained from the Bioethics Committee for Animal Experimentation of the Yeungnam University College of Medicine (permission no. YUMC-2009-021) and the animal experiments were carried out in accordance with the guidelines. (1) Seventeen adult white rabbits, weighing 2.8-3.3 kg, were used. (2) Prior to surgery, the operation site was cleaned with a mixture of iodine and 75% ethanol. The femoral metaphysis was exposed by a full thickness flap and the bone was denuded. The sites were prepared with progressive drilling under saline cooling. The test and control implants were placed in each of the distal femoral metaphysics on the right and left sides, respectively. (3) Immediately after surgery, the rabbits were kept in separate cages. (4) They were allowed post-surgical full-weight bearing and movement. Six weeks after surgery, the animals were sacrificed.
A topographic evaluation was performed by field emission scanning electron microscopy (FE-SEM, Hitachi S-4300 and 2DX-350, Tokyo, Japan) to compare surface structure on both implant groups. The peaks, valleys and flanks were measured in two implant samples. A ×500 magnification was used. Energy dispersive spectrometry (EDS) was used to evaluate the surface’s chemical composition.
Surface roughness was measured on thread-tops, flanks and valleys-selected at random on the implant surface-using an Optical Profiler (Wyko NT8000, Veeco, Tucson, AZ, USA). It was calculated as arithmetic average height (Ra), root mean square height (Rq), maximum height (Rt), and developed surface ratio (Sdr).
The removal torque of the implants was measured using a manual torque gauge (MGT12, Mark-10 Corporation, NY, USA). The result was recorded measuring the maximum removal torque at which fracture occurred between the implant and the bone. The anticlockwise movement to remove the implant was performed trying to avoid forces in directions different from the vertical.
Histologic specimens including the implant and its surrounding tissue were obtained and observed under natural and fluorescent light using an optical microscope (BX-51T, Olympus, Tokyo, Japan). A fluorescent marker (tetracycline) was injected intramuscularly to the animals at 4 and 5 weeks after placement.
Digital images with magnifications of ×20 and ×100 were captured using a digital camera. Measurements, such as the entire length of the implant, length of bone to implant contact (BIC) and degree of BIC were calculated using software (iMTechnology, Daejeon, Korea) analyses of digital optical microscopy images at ×20 magnification.
The surface morphology of the specimens was observed by FE-SEM. Images of both original samples (fixture) and removed, torqued samples (fixture) were taken at magnifications ×500 (Figs. 1, 2).
The RBM-laser implants (experimental group) showed distinct porous structures dispersed uniformly over the implant’s surface. These porous structures were approximately 20-40 μm in size (Fig. 1). The RBM implants (control group) also showed pronounced porous structures but these were irregularly dispersed and their size was only 1-5 μm (Fig. 2).
The surface roughness was measured using an Optical Profiler (Wyko NT8000, Veeco). In the experimental group, the Ra at the top, valley and incline was 9.96 μm, 6.78 μm, and 6.97 μm, respectively. The Rq at the top, valley and incline was 12.07 μm, 9.00 μm, and 8.68 μm, respectively. The Rt at the top, valley and incline was 72.83 μm, 72.80 μm, and 63.29 μm, respectively. In the control group, the Ra at the top, valley and incline was 1.08 μm, 0.87 μm, and 1.19 μm, respectively. The Rq at the top, valley and incline was 1.41 μm, 1.14 μm, and 1.53 μm, respectively. The Rt at the top, valley and incline was 18.74 μm, 16.26 μm, and 22.45 μm, respectively. The Sdr was 230.76% and 55.00% for the experimental and control groups, respectively (Table 1).
Table 2 shows the removal torque values obtained 6 weeks after implant placement. The mean removal torque in the experimental and control group was 46.6±16.4 Ncm, 24.0±10.2 Ncm, respectively.
Statistical analysis of the removal torque revealed that the experimental RBM-laser implant values were significantly higher than those of the control RBM implants (p=0.013).
In the experimental group, newly formed bone on the rough surface of the implant was observed indicating a favorable BIC.
Fluorescence microscopy observations revealed a faint fluorescent signal along part of the subperiosteum. This means that the newly formed, peri-implant bone consists of mature bone gently remodeled (Fig. 5) with ongoing bone formation, as expected at 4 or 5 weeks after surgery. There are no cementing lines or lamellar bone present. In the control group, newly formed bone into the peri-smooth implant surface was also observed, which means a relatively favorable BIC. On the other hand, vessel development occurred and a bony union was partially lost. On fluorescence microscopy, a fluorescent line was observed near the peri-implant surface, suggesting that a new bone apposition had progressed (Fig. 6).
Six weeks after surgery, the mean values of total BIC were 46.3±10.8% for the experimental group and 41.9±18.5% for the control group (Table 3). And the mean values of cortical BIC were 65.3±12.5% for the experimental group and 57.6±10.6% for the control group (Table 4). Implants with RBM-laser surfaces showed a higher degree of total and cortical BIC compared to RBM implants; however, this difference did not reach statistical significance (p=0.482, 0.225).
Our results indicated that part of the research hypothesis could be accepted. This study showed that the experimental RBM followed by laser treatment achieved a higher removal torque than that of the control RBM implants. Removal torque measurements are used as biomechanical indicators of osseointegration of implants. A positive correlation was observed between the removal torque of the implant and the amount of BIC [11], a higher removal torque might be interpreted as improved osseointegration [12].
RBM surface blasting with coarsely ground calcium phosphate gives the implant’s surface a coarse appearance without leaving residues. RBM implants have shown significantly higher removal torque values and BIC percentages than machined implants [7,8,13]. Also, laser-treated implants achieve higher removal torque values than machined implants [12].
The RBM implants showed 0.5-3.0 μm porous structures that were dispersed irregularly throughout the implant surface, whereas the RBM-laser implants had many 20-40 μm porous structures dispersed uniformly over the implant surface. These coarse porous structures contained hundreds of fine porous structures within them, which play an important role in the growth of bone tissue around the implant surface area. This indicates that active bone appositions with strong bone-to-implant attachment were achieved on the RBM-laser implant surfaces, which might be the reason for their higher removal torque.
The degree of surface titanium contamination determines the mechanical stability and the osseoconductive quality of the implant [14]. Laser processing had been reported to produce implant’s surfaces with a high degree of purity [10].
EDS analyses of the RBM implants showed considerable surface contamination with several foreign elements. However, RBM-laser implants showed less contamination with Ti and O peaks. This showed that RBM-laser treatment of the implant’s surface led to a surface roughness with a higher degree of purity than that of RBM implants.
Faeda et al. [15] reported that the surface roughness of laser-treated implants was 1.38±0.23 μm, which was ten times higher than that of machined implants. Wennerberg et al. [16] reported that screw-shaped implants with an average surface roughness of about 1.5 μm were found to be optimal for bone growth, based on removal torque test experiments. In contrast, Rønold et al. [17] found that an optimal surface roughness for bone attachment was in the range of 3.62 to 3.90 μm. Other studies state that the optimal surface roughness of laser-treated implants remains unknown [18] and that optimal roughness values may differ depending on the surface modification employed on the implant [19].
Surface roughness was measured at the top, valley and incline using an Optical Profiler (Wyko NT8000, Veeco). The experimental group revealed a considerably higher surface roughness than the control group. This suggests that a higher surface roughness increased the implant’s surface area and affected the bone-implant bonding. Moreover, considering the correlation between surface roughness and Sdr, the surface roughness of the experimental group increased significantly compared to the control group. This was attributed to the porous structures resulting from the surface treatment process of the experimental implants. In addition, the increased surface roughness of the RBM-laser implants leads to greater mechanical shear strength, resulting in the need of a higher torque for the implant to be removed.
Surface modifications through a range of processes have resulted in increased BIC and biomechanical bone bonding compared to the smooth surface of machined implants [5,20,21]. Interfacial bone formation may also be promoted by a roughened surface, as a significantly greater percentage of BIC has been observed adjacent to micro rough titanium surfaces, compared to the percentage of BIC observed on machined or polished titanium surfaces [22].
In this study, the RBM-laser-treated surfaces had a higher BIC than the control RBM surfaces. However, these differences were no statistically significant. Nevertheless, the experimental group showed higher total BIC than the control group. This might indicate that RBM-laser-treated surfaces acquire a more convenient surface topography.
Laser treatment of implants has been demonstrated to be a fast, clean, and easy method for implant’s surface modification [23]. Rong et al. [18] reported that laser-treated and acid-etched surfaces had better osteoconductivity than laser-treated surface. Recently, it was demonstrated that associating laser-treated implants with HA coatings could reduce the implant’s healing period [15]. Also, implant’s surfaces produced by dual treatment with laser etching and microarc oxidation resulted in enhanced bone responses compared to those obtained using pure titanium machined implant surfaces [24].
Within the limitation of this study, the experimental group showed a higher percentage BIC and removal torque (p=0.013), favorable surface roughness. Therefore, RBM followed by laser surface treatment can promote a favorable osseointegration. Nevertheless, further studies of RBM and laser-treated surfaces are still needed.
References
1. Levine RA, Clem DS 3rd, Wilson TG Jr, Higginbottom F, Solnit G. Multicenter retrospective analysis of the ITI implant system used for single-tooth replacements: results of loading for 2 or more years. Int J Oral Maxillofac Implants. 1999; 14:516–20.
2. Park YJ, Cho SA. Retrospective chart analysis on survival rate of fixtures installed at the tuberosity bone for cases with missing unilateral upper molars: a study of 7 cases. J Oral Maxillofac Surg. 2010; 68:1338–44.


3. Lekholm U, van Steenberghe D, Herrmann I, Bolender C, Folmer T, Gunne J, et al. Osseointegrated implants in the treatment of partially edentulous jaws: a prospective 5-year multicenter study. Int J Oral Maxillofac Implants. 1994; 9:627–35.
4. Adell R, Eriksson B, Lekholm U, Brånemark PI, Jemt T. Long-term follow-up study of osseointegrated implants in the treatment of totally edentulous jaws. Int J Oral Maxillofac Implants. 1990; 5:347–59.
5. Albrektsson T, Brånemark PI, Hansson HA, Lindström J. Osseointegrated titanium implants. Requirements for ensuring a long-lasting, direct bone-to-implant anchorage in man. Acta Orthop Scand. 1981; 52:155–70.
6. Wennerberg A, Albrektsson T, Lausmaa J. Torque and histomorphometric evaluation of c.p. titanium screws blasted with 25- and 75-microns-sized particles of Al2O3. J Biomed Mater Res. 1996; 30:251–60.
7. Gonshor A, Goveia G, Sotirakis E. A prospective, multicenter, 4-year study of the ACE Surgical resorbable blast media implant. J Oral Implantol. 2003; 29:174–80.


8. Piattelli M, Scarano A, Paolantonio M, Iezzi G, Petrone G, Piattelli A. Bone response to machined and resorbable blast material titanium implants: an experimental study in rabbits. J Oral Implantol. 2002; 28:2–8.


9. Yoo IS, Yim SB, Chung CH, Hong KS. A compare research of machined implant and RBM surface implant. J Korean Acad Periodontol. 2008; 38:467–74.


10. Gaggl A, Schultes G, Müller WD, Kärcher H. Scanning electron microscopical analysis of laser-treated titanium implant surfaces--a comparative study. Biomaterials. 2000; 21:1067–73.


11. Johansson C, Albrektsson T. Integration of screw implants in the rabbit: a 1-year follow-up of removal torque of titanium implants. Int J Oral Maxillofac Implants. 1987; 2:69–75.
12. Cho SA, Park KT. The removal torque of titanium screw inserted in rabbit tibia treated by dual acid etching. Biomaterials. 2003; 24:3611–7.


13. Kim KS, Suh KW, Leesungbok R, Ryu JJ. Removal torque of bicortically stabilized RBM (resorbable blast media) pin implants in rabbit tibia. J Korean Acad Prosthodont. 2006; 44:722–33.
14. Han CH, Johansson CB, Wennerberg A, Albrektsson T. Quantitative and qualitative investigations of surface enlarged titanium and titanium alloy implants. Clin Oral Implants Res. 1998; 9:1–10.


15. Faeda RS, Tavares HS, Sartori R, Guastaldi AC, Marcantonio E Jr. Biological performance of chemical hydroxyapatite coating associated with implant surface modification by laser beam: biomechanical study in rabbit tibias. J Oral Maxillofac Surg. 2009; 67:1706–15.


16. Wennerberg A, Albrektsson T, Andersson B, Krol JJ. A histomorphometric and removal torque study of screw-shaped titanium implants with three different surface topographies. Clin Oral Implants Res. 1995; 6:24–30.


17. Rønold HJ, Lyngstadaas SP, Ellingsen JE. Analysing the optimal value for titanium implant roughness in bone attachment using a tensile test. Biomaterials. 2003; 24:4559–64.


18. Rong M, Zhou L, Gou Z, Zhu A, Zhou D. The early osseointegration of the laser-treated and acid-etched dental implants surface: an experimental study in rabbits. J Mater Sci Mater Med. 2009; 20:1721–8.


19. Sul YT, Johansson C, Wennerberg A, Cho LR, Chang BS, Albrektsson T. Optimum surface properties of oxidized implants for reinforcement of osseointegration: surface chemistry, oxide thickness, porosity, roughness, and crystal structure. Int J Oral Maxillofac Implants. 2005; 20:349–59.
20. Albrektsson T, Wennerberg A. Oral implant surfaces: part 1--review focusing on topographic and chemical properties of different surfaces and in vivo responses to them. Int J Prosthodont. 2004; 17:536–43.
21. Albrektsson T, Wennerberg A. Oral implant surfaces: part 2--review focusing on clinical knowledge of different surfaces. Int J Prosthodont. 2004; 17:544–64.
22. Wennerberg A, Hallgren C, Johansson C, Danelli S. A histomorphometric evaluation of screw-shaped implants each prepared with two surface roughnesses. Clin Oral Implants Res. 1998; 9:11–9.


23. Jartoft P, Krantz M. Kinoform guided laser microfabrication of titanium implant surfaces [dissertation]. Göteborg: Chalmers University of Technology;1997.
24. Guo Z, Zhou L, Rong M, Zhu A, Geng H. Bone response to a pure titanium implant surface modified by laser etching and microarc oxidation. Int J Oral Maxillofac Implants. 2010; 25:130–6.
Fig. 1.
FE-SEM image of the RBM-laser sample (original magnification, ×500). RBM-laser implant (experimental group) reveals precise porous structures dispersed uniformly throughout the implant surfaces. These porous structures are about 20-40 μm in size. FE-SEM, field emission-scanning electron microscopy; RBM, resorbable blast media.

Fig. 2.
FE-SEM image of the RBM sample (original magnification, ×500). RBM implant (control group) also reveals precise porous structures, however, these dispersed irregularly and its size is only 1-5 μm. FE-SEM, field emission-scanning electron microscopy; RBM, resorbable blast media.
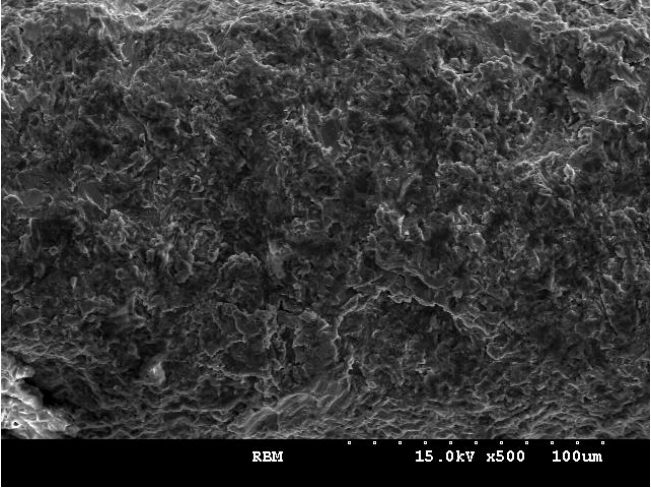
Fig. 3.
EDS spectrum of the control RBM treated implant. The EDS analysis of RBM implant showed considerable surface contamination with several foreign elements. EDS, energy dispersive spectrometer; RBM, resorbable blast media; Ti, titanium; O, oxygen.
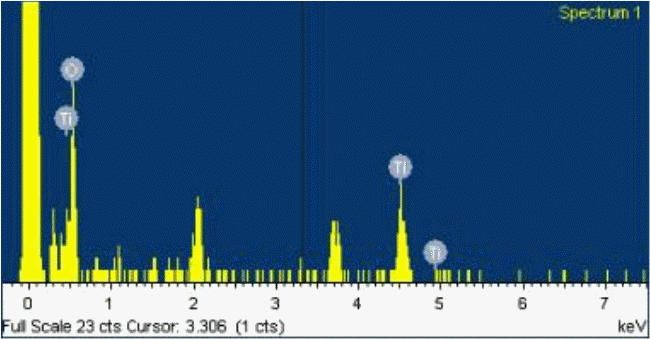
Fig. 4.
EDS spectrum of the experimental RBM-laser treated implant RBM-laser implant showed a clean surface with Ti and O peaks. EDS, energy dispersive spectrometer; RBM, resorbable blast media; Ti, titanium; O, oxygen.
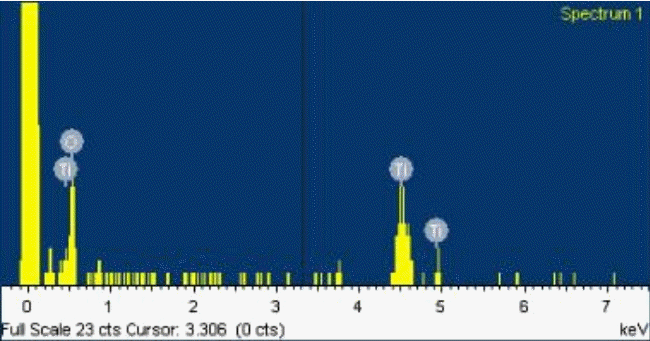
Fig. 5.
Histological image of the experimental group after 6 weeks of healing (left image, original magnification, ×20; A-D, original magnification, ×100). On the optical microscopic observation, favorable bone to implant contact by newly formed bone into the peri-implant rough surface was observed (A, B). On the fluorescence microscopic observation, the fluorescent line was seen faintly in some of subperiosteum (C, D).
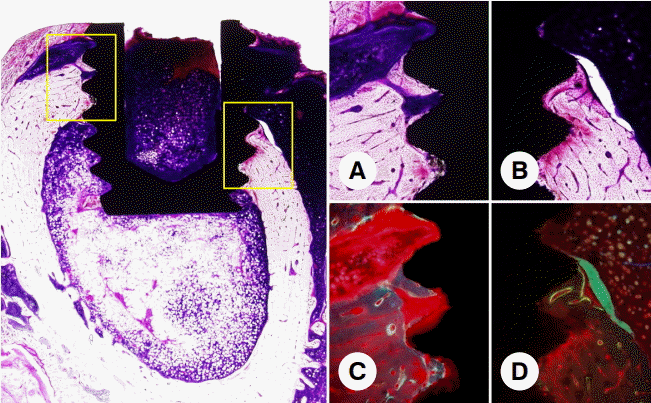
Fig. 6.
Histological image of the control group after 6 weeks of healing (left image, original magnification, ×20; A-D, original magnification, ×100). On the optical microscopic observation, newly formed bone into the peri-smooth implant surface was also observed but was observed that fibrous tissue development went, so a bony union was lost partially (A, B). On the fluorescence microscopic observation, the fluorescent line was seen near the peri-implant surface (C, D).
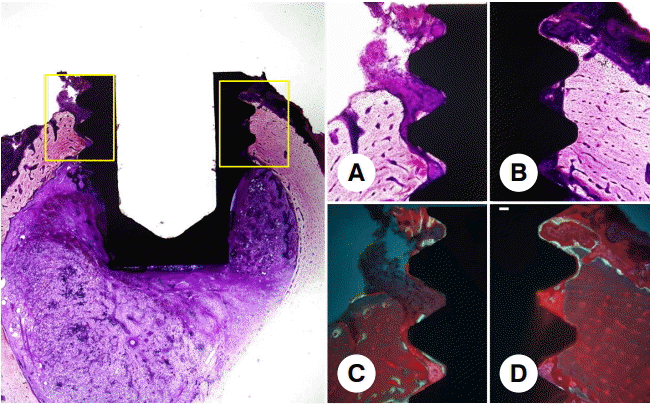
Table 1.
Surface roughness and developed surface ratio (Sdr) of the control and experimental group samples
Surface roughness value (Ra, Rq, Rt) and developed surface ratio (Sdr) of the control and experimental samples.
RBM, resorbable blast media; Ra, arithmetic average height of surface roughness; Rq, root mean square height of surface roughness; Rt, maximum height of surface roughness; Sdr, developed surface ratio of surface roughness.
Table 2.
Results of removal torque between the experimental and control groups after 6 weeks of healing
Sample | Right (experimental group) | Left (control group) | p-valuea) |
---|---|---|---|
No.1 | 35.7 | 29.4 | |
No.2 | 24.7 | 9.8 | |
No.3 | 58.9 | 37.2 | |
No.4 | 73.4 | 16.9 | |
No.5 | 48.9 | 12.8 | |
No.6 | 28.7 | 18.1 | |
No.7 | 53.1 | 28.4 | |
No.8 | 20.7 | 21.5 | |
No.9 | 40.8 | 41.2 | |
No.10 | 41.3 | 25.1 | |
Mean±SD (Ncm) | 46.6±16.4 | 24.0±10.2 | 0.013 |
Table 3.
Total BIC of the experimental and control groups after 6 weeks of healing
RBM-laser (experimental group) | RBM (control group) | p-valuea) | |
---|---|---|---|
Total BIC (%) | 38.3 | 37.4 | |
41.9 | 22.5 | ||
31.4 | 20.2 | ||
64.1 | 36.9 | ||
44.2 | 60.0 | ||
53.5 | 70.7 | ||
50.5 | 45.3 | ||
Mean±SD (%) | 46.3±10.8 | 41.9±18.5 | 0.482 |
Table 4.
Cortical BIC of the experimental and control groups after 6 weeks of healing
RBM-laser (experimental group) | RBM (control group) | p-valuea) | |
---|---|---|---|
Cortical BIC (%) | 76.1 | 66.2 | |
73.7 | 65.6 | ||
39.5 | 44.5 | ||
67.9 | 42.0 | ||
63.4 | 65.5 | ||
73.6 | 64.6 | ||
62.8 | 54.7 | ||
Mean±SD (%) | 65.3±12.5 | 57.6±10.6 | 0.225 |