Abstract
Objective
Methods
Results
Notes
Conceptualization: Kim Y, Han N, Jung SH. Methodology: Kim Y, Han N. Formal analysis: Kim Y, Kim J. Project administration: Kim Y, Kim J. Visualization: Kim Y, Kim J, Nam H. Writing - original draft: Kim Y. Writing - review and editing: Han N, Kim HD, Eom MJ. Approval of the final manuscript: all authors.
REFERENCES

































Fig. 1.
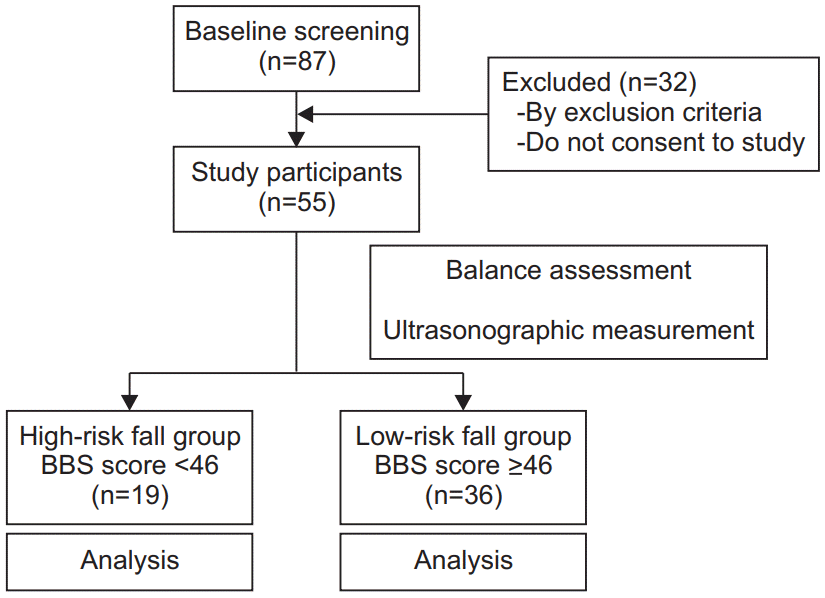
Fig. 2.
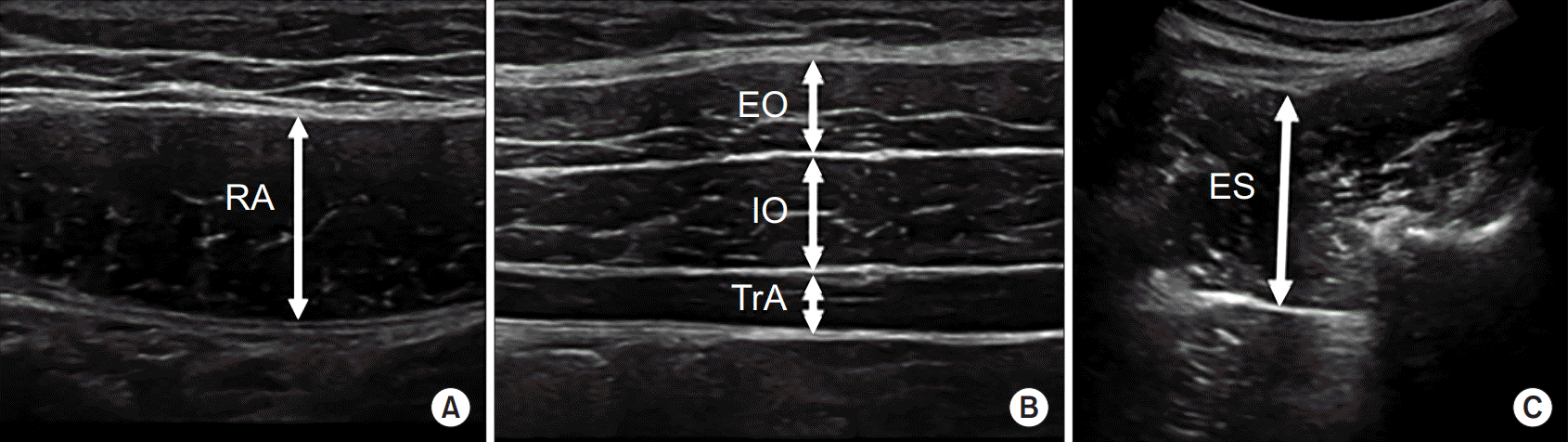
Table 1.
Values are presented as mean±standard deviation or as number (%).
BMI, body mass index; BG, basal ganglia; NIHSS, National Institutes of Health Stroke Scale; mRS, modified Rankin Scale; SARA, Scale for the Assessment and Rating of Ataxia; BBS, Berg Balance Scale; TUG, Timed Up and Go Test; TCT, Trunk Control Test.
Table 2.
Non-paretic | Paretic | p-value | |
---|---|---|---|
Rectus abdominis | |||
Resting thickness (mm) | 7.60±2.26 | 7.49±2.42 | 0.337 |
Contraction thickness (mm) | 8.95±2.54 | 8.22±2.64 | <0.001* |
Contractility | 1.19±0.12 | 1.10±0.10 | <0.001* |
External oblique | |||
Resting thickness (mm) | 3.60±1.22 | 3.56±1.04 | 0.675 |
Contraction thickness (mm) | 4.54±1.43 | 4.14±1.25 | <0.001* |
Contractility | 1.28±0.19 | 1.16±0.14 | <0.001* |
Internal oblique | |||
Resting thickness (mm) | 6.70±2.21 | 6.48±2.09 | 0.042* |
Contraction thickness (mm) | 8.68±2.71 | 7.74±2.49 | <0.001* |
Contractility | 1.30±0.15 | 1.20±1.32 | <0.001* |
Transversus abdominis | |||
Resting thickness (mm) | 3.16±1.35 | 3.00±1.29 | 0.047* |
Contraction thickness (mm) | 4.99±2.09 | 4.26±1.95 | <0.001* |
Contractility | 1.62±0.49 | 1.43±0.43 | <0.001* |
Erector spinae | |||
Resting thickness (mm) | 37.51±6.19 | 36.71±6.12 | 0.045* |
Contraction thickness (mm) | 44.47±6.56 | 41.40±7.00 | <0.001* |
Contractility | 1.19±0.11 | 1.13±0.09 | <0.001* |
Table 3.
Pearson correlation coefficient |
||||
---|---|---|---|---|
SARA | BBS | TUG | TCT | |
Rectus abdominis | ||||
NPS thickness | -0.184 | 0.191 | -0.221 | 0.101 |
PS thickness | -0.168 | 0.184 | -0.240 | -0.221 |
NPS contractility | 0.431** | -0.434** | 0.340* | -0.371** |
PS contractility | 0.067 | -0.065 | 0.068 | 0.058 |
Contractility ratio | 0.529** | -0.534** | 0.393** | -0.605** |
External oblique | ||||
NPS thickness | -0.263 | 0.309* | -0.385** | 0.118 |
PS thickness | -0.342 | 0.355 | -0.420 | -0.213 |
NPS contractility | 0.212 | -0.212 | 0.089 | -0.232 |
PS contractility | -0.222 | 0.194 | -0.241 | 0.175 |
Contractility ratio | 0.562** | -0.533** | 0.414** | -0.542** |
Internal oblique | ||||
NPS thickness | -0.115 | 0.161 | -0.220 | 0.060 |
PS thickness | -0.130 | 0.192 | -0.274 | 0.077 |
NPS contractility | 0.497** | -0.491** | 0.439** | -0.395** |
PS contractility | -0.083 | 0.100 | -0.066 | 0.063 |
Contractility ratio | 0.659** | -0.673** | 0.568** | -0.541** |
Transversus abdominis | ||||
NPS thickness | -0.118 | 0.091 | -0.119 | 0.200 |
PS thickness | -0.131 | 0.102 | -0.149 | 0.192 |
NPS contractility | -0.029 | 0.063 | -0.031 | -0.110 |
PS contractility | -0.237 | 0.247 | -0.215 | 0.070 |
Contractility ratio | 0.374** | -0.337* | 0.311* | -0.320* |
Erector spinae | ||||
NPS thickness | -0.313* | 0.392** | -0.298* | 0.134 |
PS thickness | -0.236 | 0.306* | -0.243 | 0.035 |
NPS contractility | 0.170 | -0.092 | 0.014 | 0.024 |
PS contractility | -0.237 | 0.301* | -0.354** | 0.191 |
Contractility ratio | 0.562** | -0.533** | 0.414** | -0.542** |
Table 4.
High-risk fall group (n=19) | Low-risk fall group (n=36) | p-value | |
---|---|---|---|
Rectus abdominis | |||
NPS thickness (mm) | 6.94±2.20 | 7.95±2.23 | 0.117 |
PS thickness (mm) | 6.86±2.45 | 7.81±2.36 | 0.103 |
NPS contractility | 1.23±0.13 | 1.16±1.10 | 0.061 |
PS contractility | 1.10±0.09 | 1.10±0.10 | 0.852 |
Contractility ratio | 1.11±0.09 | 1.06±0.06 | 0.001** |
External oblique | |||
NPS thickness (mm) | 3.09±1.27 | 3.86±1.12 | 0.011* |
PS thickness (mm) | 3.12±1.08 | 3.79±0.95 | 0.008* |
NPS contractility | 1.32±0.19 | 1.26±0.19 | 0.193 |
PS contractility | 1.15±0.13 | 1.17±0.15 | 0.710 |
Contractility ratio | 1.15±0.16 | 1.07±0.08 | 0.048* |
Internal oblique | |||
NPS thickness (mm) | 6.06±1.79 | 7.05±2.36 | 0.159 |
PS thickness (mm) | 5.73±1.83 | 6.88±2.14 | 0.037* |
NPS contractility | 1.37±0.14 | 1.27±0.14 | 0.044* |
PS contractility | 1.17±0.13 | 1.20±0.13 | 0.381 |
Contractility ratio | 1.16±0.14 | 1.05±0.07 | <0.001** |
Transversus abdominis | |||
NPS thickness (mm) | 2.91±1.36 | 3.30±1.34 | 0.125 |
PS thickness (mm) | 2.76±1.34 | 3.13±1.26 | 0.172 |
NPS contractility | 1.57±3.55 | 1.65±0.51 | 0.979 |
PS contractility | 1.28±0.27 | 1.51±0.47 | 0.026* |
Contractility ratio | 1.25±0.33 | 1.09±0.14 | <0.001** |
Erector spinae | |||
NPS thickness (mm) | 33.7±4.27 | 39.5±6.15 | 0.001** |
PS thickness (mm) | 33.9±4.02 | 38.1±6.55 | 0.019* |
NPS contractility | 1.20±0.13 | 1.18±0.09 | 0.818 |
PS contractility | 1.10±0.07 | 1.14±0.09 | 0.028* |
Contractility ratio | 1.10±0.07 | 1.04±0.04 | 0.002** |