Abstract
Purpose: During the treatments of cancer patients with a linear accelerator (LINAC) using photon beams with energies ≥8 MV, the components inside the LINAC head get activated through the interaction of photonuclear reaction (γ, n) and neutron capture (n, γ). We used spectroscopy and measured the dose rate for the LINAC in operation after the treatment ended.
Methods: We performed spectroscopy and dose rate measurements for three units of LINACs with a portable high-purity Germanium (HPGe) detector and a survey meter. The spectra were obtained after the beams were turned off. Spectroscopy was conducted for 3,600 seconds, and the dose rate was measured three times. We identified the radionuclides for each LINAC.
Results: According to gamma spectroscopy results, most of the nuclides were short-lived radionuclides with half-lives of 100 days, except for 60Co, 65Zn, and 181W nuclides. The dose rate for three LINACs obtained immediately in front of the crosshair was in the range of 0.113 to 0.129 µSv/h. The maximum and minimum dose rates measured on weekends were 0.097 µSv/h and 0.092 µSv/h, respectively. Compared with the differences in weekday data, there was no significant difference between the data measured on Saturday and Sunday.
Conclusions: Most of the detected radionuclides had half-lives <100 days, and the dose rate decreased rapidly. For equipment that primarily used energies ≤10 MV, when the equipment was transferred after at least 10 minutes after shutting it down, it is expected that there will be little effect on the workers’ exposure.
Radiation therapy devices and techniques have continued to develop since the 2000s, and linear accelerators (LINACs) have been used extensively and constitute the most ubiquitous treatment equipment in Korea. As of 2020, 64% (182 units) of the 285 therapeutic radiation generators installed in Korea were LINACs [1,2]. The structure and components’ materials of the LINAC differ depending on the manufacturer. The equipment and parts gradually become radioactive owing to activation phenomena during operation.
In the case of cancer treatments using a photon beam with an energy ≥8 MV, radiation occurs because of a chain reaction of the following two reactions [3,4]. With continuous irradiation, neutrons are generated through a photonuclear reaction (γ, n) inside the LINAC’s head, and these then interact with the components inside the LINAC head, such as the target, primary collimator, flattening filter, and ion chamber, causing a neutron capture (n, γ) reaction that generates gamma rays [5-7]. The LINAC gradually becomes radioactive, exposing radiologists and patients receiving treatment to unintentional radiation [8]. Furthermore, the radiation exposure to workers in the process of dismantling a LINAC which is intended to be disposed after the end of its operation should be considered. According to a disposal company in the Republic of Korea, when the LINAC is transferred for disposal or installed in another institution without dismantling the equipment before its move, the effect of radiation leakage through the crosshair for the beam exit (radiation exposure window) cannot be ignored despite the fact that the LINAC’s head is surrounded by lead or iron shields to minimize leakage radiation [9,10].
The level of activation and the detected radionuclides may vary depending on the period of use, energy, workload, and manufacturer of the equipment, and it is important to obtain and evaluate this type of information. In Korea, guidelines and regulations related to the demolition, disposal, and release of equipment are not clear. Therefore, it is believed that measuring the degree of activation by LINAC-operated periods or manufacturer can be a good standard when transferring/disposing LINACs at a similar level in the future.
In this study, to provide recommendations for the minimization of the level of exposure to workers who are responsible for the relocation and moving of LINACs intended for dismantling, we measured the dose rate and analyzed nuclides using a survey meter and gamma spectroscopy at the beam exit of the LINAC during operation.
We performed radio activation measurements and evaluations on selected LINAC types, including the Clinac iX, Infinity, and Oncor Impression Plus, which are representative LINACs from manufacturers, such as Varian (Palo Alto, CA, USA), Elekta (Stockholm, Sweden), and Siemens (Erlangen, Germany), installed in the Department of Radiation Oncology at the Hospital in Korea. We investigated the models according to the manufacturer, period of use, energy, number of patients treated per day, workload, and intensity modulated radiation therapy (IMRT) ratio (Table 1). The ratio of energy used was also confirmed. All three LINACs had a source-to-surface distance of 100 cm. The installation year and operation period were based on the measurement date. Varian’s Clinac iX LINAC was installed in 2014 and operated for eight years. Elekta’s Infinity LINAC was installed in 2021 and operated for approximately six months, and the Oncor Impression Plus LINAC (Siemens) had been used for approximately 15 years. All three LINACs used 4 MV and 6 MV energy beams at relatively high ratios equal to 70% to 80% compared with the 10 MV energy beam. With the exception of the Elekta Infinity equipment (20% IMRT ratio), the IMRT ratio was confirmed to be >80%. The average number of patients treated with the use of the Varian LINAC was 40 to 50 per day, and the average number of patients treated using the Elekta and Siemens LINACs was 20 to 30 per day.
Fig. 1 shows the gamma spectroscopy measurement and evaluation procedure diagram for the activation of the medical LINAC. Our study aims to evaluate the activation of the entire LINAC equipment without pre-processing the measurement sample/object, and a qualitative methodology for the analysis of radionuclides was presented. After linking the portable high-purity Germanium (HPGe) detector and software, the high voltage was set to 3,000 V and the measurement time was set to 3,600 seconds. Measurements were then conducted. The channel information of the spectrum was corrected with energy using a 152Eu standard source, and a peak matching the library nuclide was identified based on the calibrated energy information. The spectrum was acquired after placing the detector on the couch and in front of the beam’s exit path, and the background spectrum was measured outside the treatment room and then subtracted during the analysis process.
Following the execution of in-situ spectroscopy procedure established in this study, nuclide spectral measurements for nuclide identification were performed on the LINACs used in the medical institutions. We used a portable HPGe detector (Falcon 5000; Canberra Inc., Meriden, CT, USA), and the relative efficiency of the instrument was 18% (based on a 3˝×3˝ NaI[Tl] detector). The full-width-at-half-maximum of the gamma spectroscopy system was 0.15% (2.0 keV) for gamma rays with energy equal to 1,332 keV and 0.82% (1.0 keV) for 122 keV gamma rays. GENIE-2000 (Canberra Inc.) software was used for analysis. Channel-energy calibration was performed with 152Eu certificated reference material.
As shown in Fig. 2, after rotating the gantry of the LINAC for treatment by 90°, the portable HPGe detector was positioned in front of the beam exit, and measurements were recorded for 3,600 seconds. The height and position of the couch were adjusted such that the portable HPGe detector was located at the center of the crosshair. The background spectrum was measured outside the treatment room based on considerations that the activation had already progressed inside the treatment room. Spectral measurements were performed immediately after the treatment was finished, and it was prepared to take less than 10 minutes from the detector setup for measurement. Measurements were recorded thrice for each LINAC: the 1st measurement was obtained on weekdays after the treatment, and the 2nd and 3rd measurements were obtained on weekends (Saturday and Sunday) to identify long-lived nuclides. Spectral analyses were performed, and nuclides detected owing to activation for each manufacturer were compared.
Immediately after treatment, a survey meter (FH-40 G-L10; Thermo Scientific, Waltham, MA, USA) was used to measure the dose rate in front of the beam exit. Measurements were performed on weekdays after patient treatment and on weekends (Saturday and Sunday), and the average value and standard deviation were calculated after three measurements. Additionally, after irradiating with a 600 MU dose using a 10 MV Varian LINAC, the dose rate variation with time was confirmed.
Table 2 shows the nuclides detected after gamma spectroscopy in the Varian, Elekta, and Siemens LINACs. Nuclides marked with “P” and “m” is nuclides with clearly identified peaks in the spectral analysis, and a minor peak with low counts per second (CPS) value on the spectrum, respectively. From the gamma spectroscopy analysis of the Varian LINAC, peaks of a total of eight nuclides, including 24Na, 56Mn, 60Co, and 82Br, were clearly identified. On weekends (Sat/Sun), the same nuclides as those detected on weekdays were identified, except for 24Na and 56Mn nuclides with half-lives of 15.02 and 2.579 hours.
In the case of gamma spectroscopy conducted on the Elekta LINAC, six nuclides were detected, including 56Mn, 124Sb, 184Re, 187W, and 196Au. The 56Mn nuclide was not detected on either Saturday or Sunday, and the 82Br nuclide was detected only in the Saturday measurement data.
In the case of gamma spectroscopy conducted on the Siemens LINAC, 11 nuclides were detected based on data measured on weekdays. In addition to the nuclides 24Na, 60Co, 99Mo, 122Sb, and 181W, the 28Al nuclide with a half-live of 2.245 minutes was detected. From the weekend measurements, the same results as the nuclides identified on weekdays were confirmed, except for 24Na, 28Al, and 56Mn nuclides which had half-lives equal to 15.02 hours, 2.245 minutes, and 2.579 hours, respectively.
Fig. 3 shows a graph of the dose rate measurement using the LINAC equipment, and the average values and errors in measurements on weekdays and weekends (Saturday/Sunday). From the measured dose rated for the three LINACs evaluated in this study, the value of the dose rate in front of the beam exit immediately after the end of treatment was 0.113 to 0.129 µSv/h. The maximum and minimum dose rates measured on weekends were 0.097 and 0.092 µSv/h, respectively. Compared with weekdays, there was no significant difference between the Saturday and Sunday measured data. The background dose rate was approximately 0.097±0.0031 µSv/h.
The dose rate measurement immediately after irradiation at 500 MU using 10 MV energy of the Varian equipment was 0.702 µSv/h, and it decreased abruptly to 0.406 µSv/h in 1 minute. Subsequently, the dose rate decreased, as shown in Fig. 4, and was measured at a level similar to the natural radiation dose rate within 10 minutes.
In this study, gamma spectroscopy was performed on the Varian, Elekta, and Siemens LINACs, and the dose rates were measured in front of the beam exit pathways. From the gamma spectroscopy outcomes, most nuclides with half-lives <100 days were detected, except for the 60Co, 65Zn, and 181W nuclides. Differences in nuclides from different manufacturers were confirmed. This is expected owing to differences in constituent elements and impurities of parts by manufacturers.
According to the Varian LINAC’s manufacturer’s blueprint, tungsten was suggested as the main material for the target, collimator, and planarization filter [11]. Based on the (γ, n) and (n, γ) reactions, energy peaks of 181W and 187W nuclides were clearly identified, and 58Co and 60Co nuclides generated by materials such as copper, nickel, and iron were detected.
The target of the Elekta LINAC was composed of tungsten and rhenium, and the collimator/jaw/multileaf collimator and flattening filter parts are composed of tungsten and iron, respectively [12,13]. It is believed that tungsten and cobalt-nuclides, including 184Re nuclides, were detected owing to (γ, n) and (n, γ) interactions.
Based on the manufacturer’s blueprint, Siemens LINAC components are composed of various elements including tungsten, iron, copper, krypton, manganese, and gold, and many nuclides were detected according to the radioactive process [14]. Among them, the 198Au nuclide yielded a significantly low CPS when detected by the Siemens LINAC. Thus, the peak would only be visible after measurements for a long time compared with the other measurements.
Other nuclides, such as 25Na, 82Br, 99Mo, 122Sb, and 124Sb seem to have been generated because of impurities other than the main material of the part [15,16]. Additionally, the peak for minor nuclides measured at extremely low CPS would have been more clearly identified if gamma spectroscopy was performed for periods >1 hour.
We compared the radionuclides identified in this study with those suggested by Fisher [17], Fisher et al. [18], and Fujibuchi et al. [19] according to the LINAC manufacturer. Compared with the nuclides identified by Fisher et al., 51Cr, 54Mn, 57Co, 57Ni, 59Fe, and 64Cu were not identified, but the results for other nuclides were confirmed to be consistent. The LINACs evaluated by Fisher et al. were equipped with an energy of 15 MV, and spectroscopy was performed for 2 hours after irradiation at an arbitrarily dose of 400 MU. Moreover, when comparing the results of this study with the results of gamma spectroscopy for the Varian LINAC evaluated by Fujibuchi et al. [19], 64Cu, 110mAg, 116mIn, 196Au, and 198Au nuclides were not identified. However, in this study, spectroscopy was conducted after irradiating with a 5,000 MU dose prior to measurement. The 28Al nuclide was predicted to be undetectable because the setup time in our experiment was longer than the half-life (which is equal to 2.245 min). This difference is considered to be due to the 10 MV energy used for the measurement and the measurement time (60 h).
From the measured dose rate, it was confirmed that there was no significant difference from the background dose rate at 0.092 to 0.129 µSv/level immediately after treatment on weekends. The LINACs used for the measurements conducted in this study employed energy beams at 4 and 6 MV at a ratio of 70% to 80%. It is considered that most of the equipment types which use the corresponding energy band generate very short-lived nuclides, and the dose rate drops within a short time. Based on this, it is expected that the level of radiation varies depending on the maximum energy used, and the effects of the period of use of the equipment, the number of patients per day, and the operating load are relatively small. Based on the results from this study, the LINAC intended to transferred to be installed in another institution (with a maximum rated energy of 10 MV), or transferred for disposal, the radiation effects on the operator after at least 10 minutes of continuous operation are expected to be considerably low.
In this study, a radioactivity measurement and evaluation system using a portable HPGe detector was established, and spectroscopy and dose rate LINAC measurements were performed at the beam’s exit pathway. Regarding the equipment which used energies of 4, 6, and 10 MV, the dose rate decreased to the natural radiation dose level within 10 minutes after the termination of treatment. Most of the nuclides were short-lived radionuclides with half-lives <100 days, except for 60Co, 65Zn, and 181W. Regarding the LINAC with a maximum energy of 10 MV intended to be installed at another institution or disposal, it is expected that the effects on the workers’ exposure will be minor if the equipment is transferred after at least 10 minutes after it was shut down. However, because the LINACs used in this study used 10 MV energy beams at a relatively small ratio, additional research must be conducted on equipment using energy beams ≥10 MV. In the future, we intend to conduct a comparison and analysis of radionuclides in conjunction with Monte Carlo simulations for LINACs using equipment.
Acknowledgements
The Nuclear Safety Research Program supported this work through the Korea Foundation of Nuclear Safety (KoFONS) using financial resources granted by the Nuclear Safety and Security Commission (NSSC) of the Republic of Korea (No. 2003013) and the National Research Foundation of Korea (NRF) grant funded by the Korean government (No. 2020M2D9A3094170).
Notes
Availability of Data and Materials
All relevant data are within the paper and its Supporting Information files.
Author Contributions
Conceptualization: Na Hye Kwon and Sang Hyoun Choi. Data curation: Na Hye Kwon and Young Jae Jang. Funding acquisition: Sang Hyoun Choi and Dong Wook Kim. Investigation: Jinsung Kim. Methodology: Kum Bae Kim and Jaeryong Yoo. Project administration: Sang Hyoun Choi. Validation: Jinsung Kim, So Hyun Ahn, and Jaeryong Yoo. Visualization: Young Jae Jang. Writing–original draft: Na Hye Kwon. Writing–review & editing: Na Hye Kwon and Sang Hyoun Choi.
References
1. Korea Institute of Nuclear Safety. 2020. Current status of medical radiation devices. Korea Institute of Nuclear Safety;Daejeon:
2. Kwon NH, Jang YJ, Kim DW, Shin DO, Kim KB, Kim JS, et al. 2020; Trend analysis on Korean and international management for activated material waste from medical linear accelerator. Prog Med Phys. 31:194–204. DOI: 10.14316/pmp.2020.31.4.194.


3. Çeçen Y, Gülümser T, Yazgan Ç, Dapo H, Üstün M, Boztosun I. 2017; Photoneutron flux measurement via neutron activation analysis in a radiotherapy bunker with an 18 MV linear accelerator. EPJ Web Conf. 153:07006. DOI: 10.1051/epjconf/201715307006.


4. Vichi S, Dean D, Ricci S, Zagni F, Berardi P, Mostacci D. 2020; Activation study of a 15MeV LINAC via Monte Carlo simulations. Radiat Phys Chem. 172:108758. DOI: 10.1016/j.radphyschem.2020.108758.


5. Lee DY, Park ET, Kim JH. 2017; Evaluate the activation of linear accelerator components and shielding wall through simulation. J Korea Contents Assoc. 17:69–76.
6. Patil B, Chavan S, Pethe SN, Krishnan R, Bhoraskar V, Dhole S. 2011; Estimation of neutron production from accelerator head assembly of 15 MV medical LINAC using FLUKA simulations. Nucl Instrum Methods Phys. 269:3261–3265. DOI: 10.1016/j.nimb.2011.04.013.
7. Morató S, Juste B, Miró R, Verdú G, Diez S. 2016. Aug. 16-20. Experimental validation of neutron activation simulation of a varian medical linear accelerator. Paper presented at: 2016 38th Annual International Conference of the IEEE Engineering in Medicine and Biology Society (EMBC). Orlando, USA: p. 5656–5659. DOI: 10.1109/EMBC.2016.7592010. PMID: 28269538.


8. Lee DY, Kim JH, Park ET. 2017; Assessment of human exposure doses received by activation of medical linear accelerator components. J Instrum. 12:P08022. DOI: 10.1088/1748-0221/12/08/P08022.


9. McMorrow A. 2019; Radiation shielding and bunker design. Biomed J Sci Tech Res. 18:13320–13330. DOI: 10.26717/BJSTR.2019.18.003106.


10. Lonski P, Taylor ML, Franich RD, Harty P, Kron T. 2012; Assessment of leakage doses around the treatment heads of different linear accelerators. Radiat Prot Dosimetry. 152:304–312. DOI: 10.1093/rpd/ncs049. PMID: 22511732.


11. Varian Medical Systems. Confidential data package. Monte Carlo data package high energy accelerator. Varian Medical Systems;Crowley:
12. Elekta. High energy accelerator Monte Carlo. Confidential data package. Elekta;Crowley:
13. Joint working group of medical societies related to clearance and radiotherapy in Japan. 2016. Society standard for radiotherapy management in radiotherapy equipment. Japanese Society for Radiation Oncology;Chuo:
14. Siemens. Monte Carlo simulation for teletherapy equipment. Confidential data. Siemens Healthineers;Crowley:
15. Terashima S, Kimura N, Seino M, Kubota M, Saito H, Hosokawa Y. 2015; Analysis using an NaI(Tl) detector for radioactive materials when dismantling a linear accelerator. Radiat Emerg Med. 4:47–52.
16. Konefał A, Orlef A, Bieniasiewicz M. 2016; Measurements of neutron radiation and induced radioactivity for the new medical linear accelerator, the Varian TrueBeam. Radiat Meas. 86:8–15. DOI: 10.1016/j.radmeas.2015.12.039.


17. Fischer HW, Tabot BE, Poppe B. 2006; Activation processes in a medical linear accelerator and spatial distribution of activation products. Phys Med Biol. 51:N461–N466. DOI: 10.1088/0031-9155/51/24/N02. PMID: 17148816.


18. Fischer HW, Tabot B, Poppe B. 2008; Comparison of activation products and induced dose rates in different high-energy medical linear accelerators. Health Phys. 94:272–278. DOI: 10.1097/01.HP.0000291945.15684.a7. PMID: 18301101.


19. Fujibuchi T, Obara S, Yamaguchi I, Oyama M, Watanabe H, Sakae T, et al. 2012; Induced radioactive nuclides of 10-MeV radiotherapy accelerators detected by using a portable HP-Ge survey meter. Radiat Prot Dosimetry. 148:168–173. DOI: 10.1093/rpd/ncr005. PMID: 21317145.


Fig. 1
Diagram showing the measurement procedure and evaluation of activation of medical linear accelerators (LINACs). HPGe, high-purity Germanium; CRM, certified reference material.
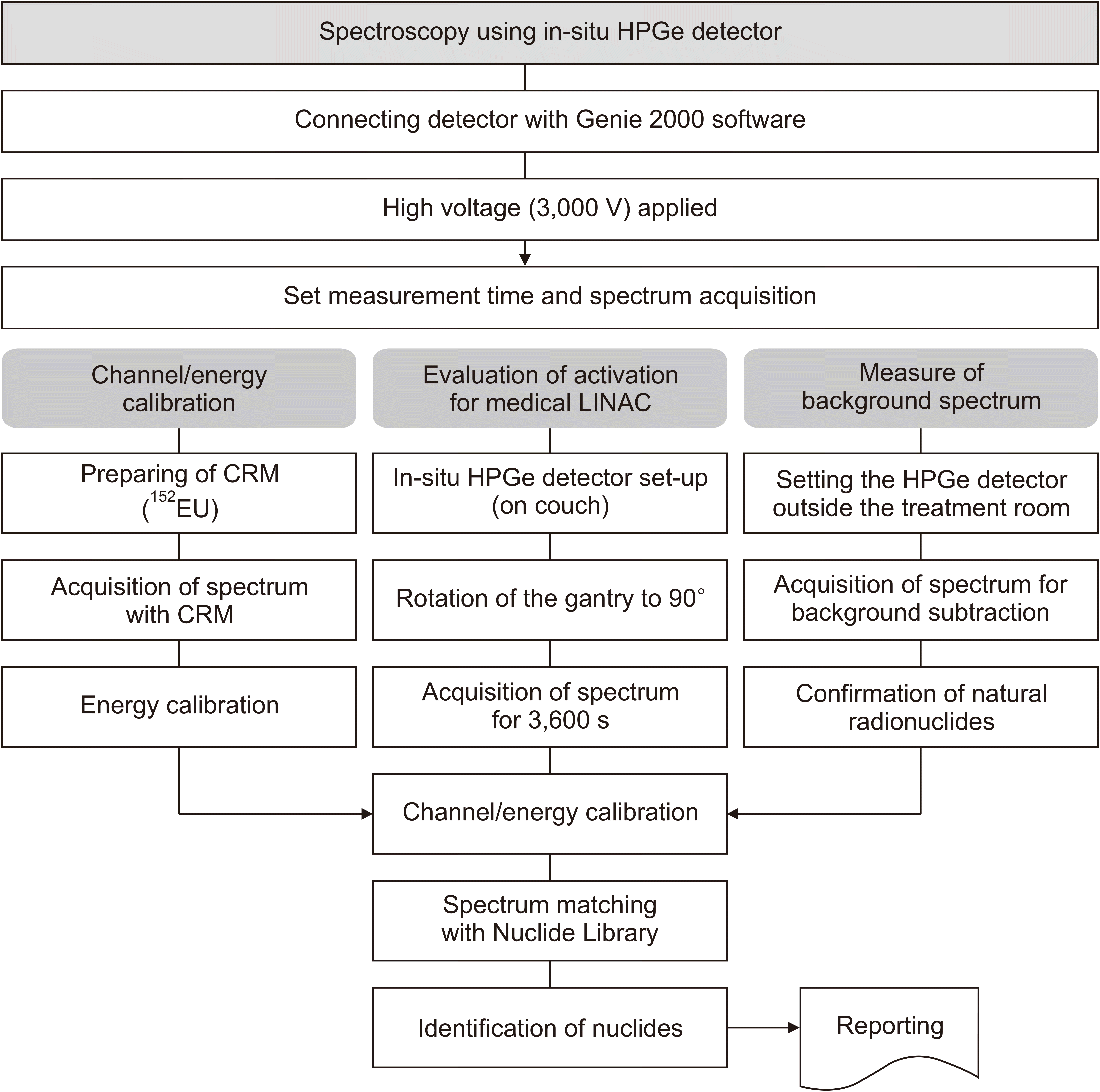
Fig. 2
Setup of the in-situ high-purity Germanium detector in front of the linear accelerator crosshair and relevant measurements.

Table 1
Information of medical linear accelerators evaluated herein
Table 2
Detected radionuclides of spectroscopy