Abstract
BACKGROUND/OBJECTIVES
Hyperglycemia-induced hepatic damage has been recognized as one of the major cause of complications in diabetes. Hepatic complications are associated with inflammation and oxidative stress in diabetes. In this study, we investigated the hypothesis that gamma-tocopherol (GT) supplementation ameliorates NLRP3 inflammasome associated hepatic inflammation in diabetes.
MATERIALS/METHODS
Diabetes was induced by the intraperitoneal injection of alloxan (150 mg/kg. BW) in ICR mice. All mice were fed with a control diet (AIN-76A). After diabetes was induced (fasting glucose level ≥ 250 mg/dL), the mice were treated with tocopherol-stripped corn oil or GT-supplemented (35 mg/kg) corn oil, respectively, by gavage for 2 weeks.
RESULTS
GT supplementation reduced fasting blood glucose levels in diabetic mice relative to non-treated diabetic mice. Moreover, GT supplementation ameliorated hyperglycemia-induced hepatic damage by regulation of NOD-like receptor protein 3 (NLRP3)-inflammasome associated inflammation represented by NLRP3, apoptosis-associated speck-like protein containing a caspase-recruitment domain, caspase-1, nuclear factor-κB pathway as well as oxidative stress demonstrated by nuclear factor erythroid 2-related factor 2, NAD(P)H dehydrogenase quinone 1, catalase and glutathione-dependent peroxidase in diabetic mice.
CONCLUSION
The findings suggested that GT supplementation ameliorated hepatic damage by attenuating inflammation and oxidative stress in alloxan-induced diabetic mice. Taken together, GT could be a beneficial nutrient that can ameliorate inflammatory responses associated with NLRP3 inflammasome in hyperglycemia-induced hepatic damage.
Type 1 diabetes mellitus (T1DM) is characterized by chronic hyperglycemia resulting from the loss of pancreatic β-cell and altered glucose metabolism [12]. As a site of the first-pass metabolism, the liver is highly sensitive to oxidative damage caused by reactive intermediates such as reactive oxygen species (ROS) and reactive nitrogen species (RNS) [234]. Overproduction of ROS leads to imbalance in antioxidant defense system [45]. Oxidative stress reduces the proliferation of mature hepatocytes and repairing of hepatic damage [46].
In particular, ROS overproduction activates NOD-like receptor, pyrin domain-containing 3 (NLRP3)-inflammasome, consisting of NLRP3, adaptor proteins such as apoptosis-associated speck-like protein containing a caspase-recruitment domain (ASC) and pro-caspase-1 [78]. NLRP3 inflammasome participates in the pathogenesis of inflammation with specific proteinase such as caspase-1 which activates interleukin-1 beta (IL-1β) [8]. NLRP-3 inflammasome also contributes to chronic inflammation by activating nuclear factor kappa B (NFκB) and its downstream molecules such as cyclooxygenase (COX-2) and inducible nitric oxide synthase (iNOS) [89]. On the other hand, sirtuin-1 (SIRT-1), an NAD+-dependent protein deacetylase, is a metabolic modulator in several cells and organs, including the liver. SIRT-1 deacetylates p65 subunit of NFκB thereby suppressing NFκB signaling cascade [1011]. Hence, regulation of NLRP3 inflammasome related inflammation would be a good strategy for improvement of hepatic inflammation in DM. Another central target of ROS is nuclear factor (erythroid-derived 2)-like 2 (Nrf2), a key transcription factor maintaining cellular redox homeostasis [5]. Nrf2 modulates its down-stream mediators such as NAD(P)H dehydrogenase quinone 1 (NQO1) and catalase, which remove excessive ROS [5].
NLRP3 inflammasome also induces hepatocyte pyroptotic cell death and hepatic fibrosis [12]. Especially, inflammatory factors such as NFκB and tumor necrosis factor alpha (TNF-α) trigger apoptosis-related mediators such as caspase-3, caspase-8, Bax and iNOS [13]. Hepatic stellate cells (HSCs) rapidly activated into proliferative, contractile and fibrogenic myofibroblast cells in chronic inflammatory state [1213]. Activated HSCs which facilitate the expression of kinase C (PKC), alpha-smooth muscle actin (α-SMA), transforming growth factor beta 1 (TGFβ1), and collagen lead to proliferation, migration, and contractility causing hepatic fibrosis [13].
Vitamin E is known as an anti-oxidant and anti-inflammatory nutrient that prevents metabolic disorders, such as obesity, cancer, and diabetes [141516]. Gamma (γ)-tocopherol (GT) is commonly used in western diets. Although there are a few studies on the biological functions of GT other than alpha (α)-tocopherol (AT) in the body, studies have reported that the antioxidant and anti-inflammatory potentials of GT are considerable [17]. GT is known to lower the protein levels of COX-2, TNF-α, and IL-1β in diabetic renal damage and lipopolysaccharideactivated macrophages [181920]. Other studies suggested that GT could modulate inflammation and oxidative stress in subjects with metabolic syndrome [2021]. In addition, GT has an effect on protecting against advanced beta cell autoimmunity in young children [22]. Our previous study reported that short term GT supplementation showed beneficial effect on lowering fasting blood glucose (FBG) levels in DM mice [1819]. Furthermore, GT supplementation regulated hyperglycemia-induced acute kidney damage by amelioration of oxidative stress, inflammation, and fibrosis in T1DM [18].
Although GT exerts anti-oxidant and anti-inflammatory properties, it is under-evaluated compared to AT [23]. Beneficial effect of GT on diabetes should be carefully considered and investigated.
This study hypothesized that short-term GT supplementation protected hyperglycemia-induced hepatic damage through amelioration of inflammation associated with NLRP3 inflammasome, oxidative stress, apoptosis and fibrosis in alloxan-induced diabetic mice.
ICR male mice (4-week-old) were obtained from Nara Bio (Gyeonggi-do South Korea). Mice were lodged in individual cages at a constant temperature with a 12 h dark/light cycle. All the mice were given access to distilled water and food ad libitum. After an acclimation for 1 week, alloxan (150 mg/kg. BW. i.p) dissolved in saline was injected to induce diabetes in mice [13]. Non-diabetic mice were injected with only a saline vehicle. After 1 week, FBG levels in diabetic mice were measured after fasting by tail vein sampling. Mice with FBG > 250 mg/dL were considered as diabetes. All the experiments were accepted by the Kyung Hee University Institutional Animal Care and Use Committee [KHUASP (SE)-14-007].
All the mice were fed a modified AIN-76A rodent diet (Research Diets, New Brunswick, NJ, USA). The animals were assigned into three experimental groups; non-diabetic mice administered with tocopherol-stripped corn oil (MP Biomedicals Inc, Solon, OH, USA ) (CON), diabetic control mice administered with tocopherol-stripped corn oil (DMC) and diabetic mice administered with 35 mg/kg gamma-tocopherol (D-gamma-Tocopherol, 95%, Acros Organics, Geel, Belgium) dissolved in tocopherol- stripped corn oil (GT). Each group consisted of more than 6 mice. Dietary treatment was orally gavaged 5 times weekly for 2 weeks. During the treatment period, body weight, food intake and fasting blood glucose levels were recorded once a week. After the treatment, the animals were anesthetized by isoflurane. The hepatic tissues were collected two weeks after GT supplementation and stored at −80℃ until they were analysis.
The levels of glutamate oxaloacetate transaminase (GOT) and glutamate pyruvate transaminase (GPT) in plasma were measured using commercial kits (Bio-Clinical System, Gyeonggi-do, South Korea), according to the manufacturer's recommendations.
Hepatic tissues were homogenized using lysis buffer (20 mM Tri-HCl, 150 mM NaCl (pH 7.5), 10% NP40, 10% Na-deoxycholate stock solution, 100 mM EDTA and 10% SDS) with a mixture of protease inhibitors (1:200 v/v, Sigma-Aldrich) on ice. The tissue homogenates were centrifuged at 18,407 g for 30 min. For the preparation of nuclear fraction, hepatic tissues were homogenized in buffer A (0.6% Nonidet P 40, 150 mM NaCl, 10 mM HEPES (pH 7.9), 1 mMEDTA, 0.5 mM PMSF, leupeptin, pepstatin and aprotinin). The homogenates were centrifuged at 2,348 g for 10 min. The pelleted nuclei were resuspended in buffer B (25% glycerol, 20 mM HEPES (pH 7.9), 420 mM NaCl, 1.2 mM MgCl2, 0.2 mM EDTA, 0.5 mM dithiothreitol (DDT), 0.5 mM PMSF, benzamidine, leupeptin, pepstatin, and aprotinin) followed by incubation on ice for 20 min. The resulting supernatants were frozen at −80℃ until further use. Equal amounts of protein (30 ug) samples were separated with SDS-PAGE followed by blotting with specific primary antibodies, followed by incubation with corresponding secondary antibodies for 1h at room temperature. Detection was performed using the ECL luminol reagent. Subsequently, western blot images were recorded and quantified with the Syngene G box.
The results are presented as means ± standard error means (SEM). Significant differences were determined by one-way ANOVA followed by Duncan's multiple range test using SPSS (version 20.0 for Windows, SPSS Inc., Chicago, IL, USA). A probability level of P < 0.05 was considered statistically significant.
One week after alloxan injection, the diabetic mice had lower body weights than the CON group. The DMC group showed an increase in food intake compared with the CON group. GT supplementation did not normalize body weight and food intake in the diabetic mice regardless of the dietary treatment (Table 1). A significant increase in the levels of fasting blood glucose was observed in the DMC group relative to the CON group with a significant decrease after 2 weeks. Plasma GOT and GPT levels were measured as indicators of biomarkers of hepatic injury. GOT level in the DMC group was significantly higher compared with the CON group. GT treatment significantly lowered GOT level compared to the DMC group. There was no significant difference in GPT level between the groups. The GOT/GPT ratio in the DMC group was higher than the CON group. However, GT supplementation did not normalize the GOT/GPT ratio in the diabetic mice (Table 1).
The level of 4-hydroxynonenal (4-HNE) in hepatic tissue was used as a marker of lipid peroxidation. The level of 4-HNE was significantly higher in the DMC group relative to the CON group. GT treatment significantly lowered the 4-HNE level compared with the DMC group (Fig. 1A).
The protein levels of oxidative stress-related markers, such as nuclear Nrf2, catalase, GPx and NQO1 were significantly elevated in the DMC group compared to the CON group. GT treatment significantly declined the protein level of Nrf2 compared to that of the DMC group. The protein levels of NQO1, catalase, and GPx, the representative target genes of the Nrf2 pathway, were significantly lower in the GT group compared to the DMC group. The protein level of MnSOD was not different among the groups (Fig. 1B).
The protein levels of NLRP3 inflammasome-related markers, including NLRP3, ASC, pro-caspase-1, caspase-1, pro-IL-1β, and IL-1β were measured by western blot in hepatic tissue to investigate the roles of NLRP3 inflammasome. GT supplementation reduced the protein levels of NLRP3 inflammasome-related markers in the hyperglycemia-induced diabetic condition. As shown in Fig. 2, GT supplementation significantly lowered the protein level of NLRP3 compared to the DMC group. The protein level of ASC in the DMC group was higher than the CON group and GT supplement did not normalize the protein level of ASC. Moreover, the protein levels of pro-caspase-1, caspase-1, pro-IL-1β and IL-1β, which were higher in the DMC group compared to the CON group, were significantly lower in the GT group compared with the DMC group (Fig. 2).
The protein levels of inflammatory response related markers, including NFκB, TNF-α, SIRT1, MCP-1, IL-6, COX2 and iNOS were measured in hepatic tissue by western blot. GT supplementation reduced the protein levels of inflammation-related markers in the hyperglycemia-induced diabetic condition. As shown in Fig. 3, a significant increase in nuclear NFκB was observed in the DMC group compared to that of the CON group, while the GT treatment resulted in a decrease in NFκB in the diabetic mice. The protein levels of TNF-α, MCP-1, IL-6, iNOS, and COX2 were significantly higher in the DMC group relative to the CON group. However, significant decreases in the protein levels of TNF-α, MCP-1, iNOS, and COX2 were shown in the GT group compared with the DMC group. The protein level of IL-6 in the GT group was not normalized compared to that of the DMC group. There was no significant difference in the protein level of SIRT1 between the DMC group and the CON group. However, GT treatment significantly increased the protein level of SIRT1 compared to the DMC group (Fig. 3).
The protein levels of caspase-3 and caspase-8 were used to measure the markers related to hepatic apoptosis. The protein level of pro-caspase-8 in the DMC group was significantly higher than that of the CON group. The protein levels of caspase-3 and caspase-8 were higher in the DMC group relative to those of the CON group. However, the protein levels of caspase-3 and caspase-8 in the GT group were significantly lower than those of the DMC group (Fig. 4A, B). Moreover, the protein level of Bax was significantly higher in the DMC group relative to the CON group. The GT group showed a significantly lower protein level of Bax compared to the DMC group. The protein level of Bcl2 was not different among the groups. The Bax/Bcl2 ratio, regulating cell proliferation and cell cycling progression, was significantly higher in the DMC group compared to the CON group. The Bax/Bcl2 ratio was lower in the GT group compared to that of the DMC group (Fig. 4C).
The protein levels of α-SMA, TGFβ1, PKC and collagen were used as markers for hepatic fibrosis. The protein levels of TGFβ1, α-SMA, PKC and collagen in the DMC group were significantly higher compared with the CON group. The GT group showed significantly lower levels of α-SMA, TGFβ1, PKC and collagen than the DMC group (Fig. 5).
The current study aimed to examine the effect of GT supplementation on hyperglycemia-induced hepatic damage in T1DM mice. The results of this study demonstrated that GT effectively ameliorated inflammation via NLRP3 inflammasome, oxidative stress, apoptosis and fibrosis under diabetic condition.
In this study, GT supplementation significantly reduced FBG levels in accordance with our previous studies [1819]. Further, GT ameliorated hepatic malfunction demonstrated by GOT level in DM. Our results suggest that GT can be a potential nutrient attenuating hyperglycemia without extra hepatic burden. The results are very similar to the effects of AT in diabetes which has already been proven by previous studies [182425].
It is well-known that hyperglycemia-induced oxidative stress activates various signaling pathways that trigger hepatic damage in diabetes [2627]. In particular, oxidative stress induces Nrf2 which regulates transcription of antioxidant defense enzymes [27]. A previous study reported that GT exhibited a strong antioxidant property against lipid peroxidation induced by peroxynitrite in vitro [23]. Further, GT showed the beneficial effects on oxidative stress demonstrated by malondialdehydes, GPx, catalase, heme oxygenase-1 (HO-1) in diabetic renal damage [19]. Similarly, our study showed that GT supplementation reduced oxidative stress resulting in lower level of 4-HNE as well as Nrf2 and its downstream genes such as NQO1, catalase, and GPx in diabetic mice. The results suggest that GT can ameliorate hyperglycemia-induced hepatic oxidative stress as a potent antioxidant agent to trap ROS in DM.
Another sensitive marker of ROS is the NLRP3 inflammasome that is involved in hepatic inflammation [78]. NLRP3 facilitates the subsequent activation of caspase-1 by auto-cleavage and mature caspase-1 cleaves the preform of IL-1β, which leading to NFκB activation [78]. NFκB triggers gene expression of various inflammatory mediators including TNF-α, IL-1β, iNOS, and COX-2 through an amplification loop [2829]. Several studies have found that expression of these inflammatory markers were higher in diabetic hepatic tissues [2930]. Similarly, the current study demonstrated that diabetic mice showed higher hepatic protein levels of NLRP3 inflammasome, NFκB and its downstream proteins including TNF-α, MCP-1, iNOS, and COX-2 compared with the CON group. However, GT supplementation attenuated hepatic inflammatory response associated with the NLRP3 inflammasome in DM. Furthermore, GT supplementation increased the protein level of SIRT1 thereby suppressing NFκB activation in diabetes. It has been reported that GT modulated COX-2 activity in lipopolysaccharide-activated macrophages [24]. Moreover, GT showed anti-inflammatory properties compared to AT through inhibiting COX-2 and the ability to scavenge RNS [17]. Our previous study also demonstrated that supplementation both AT and GT showed similar beneficial effects on NFκB associated inflammatory response including IL-1β and MCP-1 in hyperglycemia-induced acute kidney inflammation [19]. Thus, it might be inferred that GT could be effective against hyperglycemia-induced hepatic inflammation through down-regulation of NLRP3 inflammasome and NFκB activation.
Oxidative stress and inflammation also promote apoptosis and fibrosis in DM [1213]. Inflammatory mediators such as TNF-α and iNOS initiate a novel pathway of programmed cell death termed pyroptosis and hepatic fibrosis, apparently causing hepatic damage in diabetes [9313233]. In addition, ROS triggers mitochondrial apoptosis by functioning with the Bcl2 family protein and activation of caspase-3 [3435]. The current study similarly showed that these apoptosis-related markers were increased. However, GT supplementation reduced hyperglycemia-induced apoptosis demonstrated by the reduced protein levels of caspase-3, caspase-8 and the Bax/Bcl2 ratio in diabetes. A previous study also showed that GT exhibits anti-proliferative and pro-apoptotic effects on cancer cells [36]. Furthermore, NLRP3 inflammasome triggers HSCs activation and transformation into myofibroblasts, which ultimately develop into collagen and other deposition of extracellular matrix (ECM) in the liver [1213]. Eventually, it causes granuloma and hepatic fibrosis in DM [37]. Findings of this study also showed that GT administration reduced the protein levels of α-SMA, TGFβ1, PKC, and collagen in diabetic mice, further supporting the potential therapeutic effect of GT on hepatic apoptosis and fibrosis in diabetes.
Taken together, the findings demonstrated that GT supplementation exerts the ameliorative effects on NLRP3 inflammasome activation which is involved in the pathogenesis of the inflammatory response following apoptosis and fibrosis in hyperglycemia induced hepatic damage. Therefore, GT would be considered as a beneficial nutrient for the prevention of hyperglycemia-induced hepatic damage in diabetic patients.
Figures and Tables
Fig. 1
Effect of GT supplementation on hepatic protein levels of 4-hydroxynonenal (4-HNE) (A) and oxidative stress related markers (B): nuclear Nrf2, NQO1, catalase, GPx and MnSOD in alloxan-induced diabetic mice (n = 6).
The hepatic protein was measured by western blot. A representative image is shown in the below panel, the bands were normalized to the band levels of α-tubulin (cytosol) or Lamin B1 (nucleus). Data are presented as means ± SEM. Values with the same superscript letter are not significantly different (P < 0.05). CON, non-diabetic mice; DMC, diabetic control mice; GT, diabetic mice supplemented with gamma-tocopherol 35 mg/kg BW.
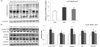
Fig. 2
Effect of GT supplementation on hepatic protein levels of NLRP3 inflammasome related markers: NLRP3, ASC, pro-caspase-1, caspase-1, pro-IL-1β and IL-1β in alloxan-induced diabetic mice (n = 6).
The hepatic protein was measured by western blot. A representative image is shown in the below panel, the bands were normalized to the band levels of α-tubulin (cytosol). Data are presented as means ± SEM. Values with the same superscript letter are not significantly different (P < 0.05). CON, non-diabetic mice; DMC, diabetic control mice; GT, diabetic mice supplemented with gamma-tocopherol 35 mg/kg BW.
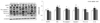
Fig. 3
Effect of GT supplementation on protein levels of inflammatory response related markers: NFκB, SIRT1, TNF-α, MCP-1, IL-6, iNOS and COX-2 in alloxan-induced diabetic mice (n = 6).
The hepatic protein was measured by western blot. A representative image is shown in the below panel, the bands were normalized to the band levels of α-tubulin (cytosol) or Lamin B1 (nucleus). Data are presented as means ± SEM. Values with the same superscript letter are not significantly different (P < 0.05). CON, non-diabetic mice; DMC, diabetic control mice; GT, diabetic mice supplemented with gamma-tocopherol 35 mg/kg BW.
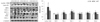
Fig. 4
Effect of GT supplementation on hepatic protein levels of apoptosis related markers: caspase-3 (A), caspase-8 (B) and Bax-Bcl2 ratio (C) in alloxan-induced diabetic mice (n = 6).
The hepatic protein was measured by western blot. A representative image is shown in the below panel, the bands were normalized to the band levels of α-tubulin (cytosol). Data are presented as means ± SEM. Values with the same superscript letter are not significantly different (P < 0.05). CON, non-diabetic mice; DMC, diabetic control mice; GT, diabetic mice supplemented with gamma-tocopherol 35 mg/kg BW.
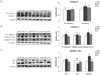
Fig. 5
Effect of GT supplementation on hepatic protein levels of fibrosis: α-SMA, TGFβ1, PKC and collagen in alloxan-induced diabetic mice (n = 6).
The hepatic protein was measured by western blot. A representative image is shown in the below panel, the bands were normalized to the band levels of α-tubulin (cytosol). Data are presented as means ± SEM. Values with the same superscript letter are not significantly different (P < 0.05). CON, non-diabetic mice; DMC, diabetic control mice; GT, diabetic mice supplemented with gamma-tocopherol 35 mg/kg BW.
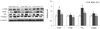
Notes
References
1. Pagano E, De Rosa M, Rossi E, Cinconze E, Marchesini G, Miccoli R, Vaccaro O, Bonora E, Bruno G, Observatory AD. The relative burden of diabetes complications on healthcare costs: the population-based CINECA-SID ARNO Diabetes Observatory. Nutr Metab Cardiovasc Dis. 2016; 26:944–950.


3. Käräjämäki AJ, Bloigu R, Kauma H, Kesäniemi YA, Koivurova OP, Perkiömäki J, Huikuri H, Ukkola O. Non-alcoholic fatty liver disease with and without metabolic syndrome: different long-term outcomes. Metabolism. 2017; 66:55–63.


4. Maritim AC, Sanders RA, Watkins JB 3rd. Diabetes, oxidative stress, and antioxidants: a review. J Biochem Mol Toxicol. 2003; 17:24–38.


5. Loboda A, Damulewicz M, Pyza E, Jozkowicz A, Dulak J. Role of Nrf2/HO-1 system in development, oxidative stress response and diseases: an evolutionarily conserved mechanism. Cell Mol Life Sci. 2016; 73:3221–3247.


6. Mohamed J, Nazratun Nafizah AH, Zariyantey AH, Budin SB. Mechanisms of diabetes-induced liver damage: the role of oxidative stress and inflammation. Sultan Qaboos Univ Med J. 2016; 16:e132–e141.


7. Hu C, Ding H, Li Y, Pearson JA, Zhang X, Flavell RA, Wong FS, Wen L. NLRP3 deficiency protects from type 1 diabetes through the regulation of chemotaxis into the pancreatic islets. Proc Natl Acad Sci U S A. 2015; 112:11318–11323.


8. Abderrazak A, Syrovets T, Couchie D, El Hadri K, Friguet B, Simmet T, Rouis M. NLRP3 inflammasome: from a danger signal sensor to a regulatory node of oxidative stress and inflammatory diseases. Redox Biol. 2015; 4:296–307.


9. Kang HH, Kim IK, Lee HI, Joo H, Lim JU, Lee J, Lee SH, Moon HS. Chronic intermittent hypoxia induces liver fibrosis in mice with diet-induced obesity via TLR4/MyD88/MAPK/NF-kB signaling pathways. Biochem Biophys Res Commun. 2017; 490:349–355.


10. Kauppinen A, Suuronen T, Ojala J, Kaarniranta K, Salminen A. Antagonistic crosstalk between NF-κB and SIRT1 in the regulation of inflammation and metabolic disorders. Cell Signal. 2013; 25:1939–1948.


11. Cao Y, Jiang X, Ma H, Wang Y, Xue P, Liu Y. SIRT1 and insulin resistance. J Diabetes Complications. 2016; 30:178–183.


12. Alegre F, Pelegrin P, Feldstein AE. Inflammasomes in liver fibrosis. Semin Liver Dis. 2017; 37:119–127.


14. Li J, Cordero P, Nguyen V, Oben JA. The role of vitamins in the pathogenesis of non-alcoholic fatty liver disease. Integr Med Insights. 2016; 11:19–25.


15. Lee H, Lim Y. Tocotrienol-rich fraction supplementation reduces hyperglycemia-induced skeletal muscle damage through regulation of insulin signaling and oxidative stress in type 2 diabetic mice. J Nutr Biochem. 2018; 57:77–85.


16. Smolarek AK, Suh N. Chemopreventive activity of vitamin E in breast cancer: a focus on γ- and δ-tocopherol. Nutrients. 2011; 3:962–986.


17. Jiang Q, Ames BN. Gamma-tocopherol, but not alpha-tocopherol, decreases proinflammatory eicosanoids and inflammation damage in rats. FASEB J. 2003; 17:816–822.


18. Shin H, Eo H, Lim Y. Similarities and differences between alpha-tocopherol and gamma-tocopherol in amelioration of inflammation, oxidative stress and pre-fibrosis in hyperglycemia induced acute kidney inflammation. Nutr Res Pract. 2016; 10:33–41.


19. Shin J, Yang SJ, Lim Y. Gamma-tocopherol supplementation ameliorated hyper-inflammatory response during the early cutaneous wound healing in alloxan-induced diabetic mice. Exp Biol Med (Maywood). 2017; 242:505–515.


20. Peh HY, Tan WS, Liao W, Wong WS. Vitamin E therapy beyond cancer: Tocopherol versus tocotrienol. Pharmacol Ther. 2016; 162:152–169.


21. Himmelfarb J, Kane J, McMonagle E, Zaltas E, Bobzin S, Boddupalli S, Phinney S, Miller G. Alpha and gamma tocopherol metabolism in healthy subjects and patients with end-stage renal disease. Kidney Int. 2003; 64:978–991.


22. Uusitalo L, Nevalainen J, Niinistö S, Alfthan G, Sundvall J, Korhonen T, Kenward MG, Oja H, Veijola R, Simell O, Ilonen J, Knip M, Virtanen SM. Serum alpha- and gamma-tocopherol concentrations and risk of advanced beta cell autoimmunity in children with HLA-conferred susceptibility to type 1 diabetes mellitus. Diabetologia. 2008; 51:773–780.


23. Jiang Q, Elson-Schwab I, Courtemanche C, Ames BN. gamma-tocopherol and its major metabolite, in contrast to alpha-tocopherol, inhibit cyclooxygenase activity in macrophages and epithelial cells. Proc Natl Acad Sci U S A. 2000; 97:11494–11499.


24. Roldi LP, Pereira RV, Tronchini EA, Rizo GV, Scoaris CR, Zanoni JN, Natali MR. Vitamin E (alpha-tocopherol) supplementation in diabetic rats: effects on the proximal colon. BMC Gastroenterol. 2009; 9:88.


25. Kim GH, Chung JW, Lee JH, Ok KS, Jang ES, Kim J, Shin CM, Park YS, Hwang JH, Jeong SH, Kim N, Lee DH, Kim JW. Effect of vitamin E in nonalcoholic fatty liver disease with metabolic syndrome: A propensity score-matched cohort study. Clin Mol Hepatol. 2015; 21:379–386.


26. Agca CA, Tuzcu M, Hayirli A, Sahin K. Taurine ameliorates neuropathy via regulating NF-κB and Nrf2/HO-1 signaling cascades in diabetic rats. Food Chem Toxicol. 2014; 71:116–121.


27. Gupte AA, Lyon CJ, Hsueh WA. Nuclear factor (erythroid-derived 2)-like-2 factor (Nrf2), a key regulator of the antioxidant response to protect against atherosclerosis and nonalcoholic steatohepatitis. Curr Diab Rep. 2013; 13:362–371.


28. Harijith A, Ebenezer DL, Natarajan V. Reactive oxygen species at the crossroads of inflammasome and inflammation. Front Physiol. 2014; 5:352.


29. Esposito K, Nappo F, Marfella R, Giugliano G, Giugliano F, Ciotola M, Quagliaro L, Ceriello A, Giugliano D. Inflammatory cytokine concentrations are acutely increased by hyperglycemia in humans: role of oxidative stress. Circulation. 2002; 106:2067–2072.


30. Li X, Gao Y, Xu H, Hou J, Gao P. Diabetes mellitus is a significant risk factor for the development of liver cirrhosis in chronic hepatitis C patients. Sci Rep. 2017; 7:9087.


31. Rath PC, Aggarwal BB. TNF-induced signaling in apoptosis. J Clin Immunol. 1999; 19:350–364.
32. Ingaramo PI, Ronco MT, Francés DE, Monti JA, Pisani GB, Ceballos MP, Galleano M, Carrillo MC, Carnovale CE. Tumor necrosis factor alpha pathways develops liver apoptosis in type 1 diabetes mellitus. Mol Immunol. 2011; 48:1397–1407.


33. Carnovale CE, Ronco MT. Role of nitric oxide in liver regeneration. Ann Hepatol. 2012; 11:636–647.


34. Zheng QY, Cao ZH, Hu XB, Li GQ, Dong SF, Xu GL, Zhang KQ. LIGHT/IFN-γ triggers β cells apoptosis via NF-κB/Bcl2-dependent mitochondrial pathway. J Cell Mol Med. 2016; 20:1861–1871.


35. Boumela I, Assou S, Aouacheria A, Haouzi D, Dechaud H, De Vos J, Handyside A, Hamamah S. Involvement of BCL2 family members in the regulation of human oocyte and early embryo survival and death: gene expression and beyond. Reproduction. 2011; 141:549–561.

