Abstract
BACKGROUND/OBJECTIVES
Neuroinflammation plays critical role in neurodegenerative disorders, such as Alzheimer's disease (AD). We investigated the effect of three licorice varieties, Glycyrhiza uralensis, G. glabra, and Shinwongam (SW) on a mouse model of inflammation-induced memory and cognitive deficit.
MATERIALS/METHODS
C57BL/6 mice were injected with lipopolysaccharide (LPS; 2.5 mg/kg, intraperitoneally) and orally administrated G. uralensis, G. glabra, and SW extract (150 mg/kg/day). SW, a new species of licorice in Korea, was combined with G. uralensis and G. glabra. Behavioral tests, including the T-maze, novel object recognition and Morris water maze, were carried out to assess learning and memory. In addition, the expressions of inflammation-related proteins in brain tissue were measured by western blotting.
RESULTS
There was a significant decrease in spatial and objective recognition memory in LPS-induced cognitive impairment group, as measured by the T-maze and novel object recognition test; however, the administration of licorice ameliorated these deficits. In addition, licorice-treated groups exhibited improved learning and memory ability in the Morris water maze. Furthermore, LPS-injected mice had up-regulated pro-inflammatory proteins, such as inducible nitric oxide synthase (iNOS), cyclooxygenase-2, interleukin-6, via activation of toll like receptor 4 (TLR4) and nuclear factor-kappa B (NFκB) pathways in the brain. However, these were attenuated by following administration of the three licorice varieties. Interestingly, the SW-administered group showed greater inhibition of iNOS and TLR4 when compared with the other licorice varieties. Furthermore, there was a significant increase in the expression of brain-derived neurotrophic factor (BDNF) in the brain of LPS-induced cognitively impaired mice that were administered licorice, with the greatest effect following SW treatment.
There is an increase in the worldwide prevalence of neurodegenerative diseases due to an increasing life expectancy [1]. Alzheimer's disease (AD) is a major neurodegenerative disorder of the elderly that is characterized by progressive impairments in memory and cognitive abilities [1]. Previous research has characterized the neuropathology of AD as amyloid beta (Aβ) plaques and neurofibrillary tangles (NFTs) in the brain [23]. The mechanism underlying Aβ-related neurotoxicity is unknown; however, studies have reported oxidative stress from the over-production of reactive oxygen species (ROS) and reactive nitrogen species (RNS) [4]. Furthermore, Aβ-induced oxidative stress activates neuroinflammation by releasing pro-inflammatory mediators, such as inducible nitric oxide synthase (iNOS) and cyclooxygenase-2 (COX-2); and inflammatory cytokines, including interleukin (IL)-1, -6, and -1β [567]. Neuroinflammation in the central nervous system induces disturbances in neurotransmitters, a loss of neuronal cells, and cognitive deficits, which may eventually result in AD [67].
Lipopolysaccharide (LPS), an endotoxin isolated from Gramnegative bacteria, plays the important role in pathogenesis of inflammatory responses and neuronal damage both in vitro and in vivo [8910]. LPS stimulates pro-inflammatory signaling cascades via toll like receptor 4 (TLR4)-mediated signaling pathways and the activation of nuclear factor-kappa B (NF-κB) [1112]. Activated NF-κB up-regulates the expression of inflammatory mediators and cytokines [12]. Subsequently, many studies have focused on the development of therapeutic agents to prevent AD by regulating inflammatory reaction using LPS-induced models [101314].
Licorice species (Glycyrhiza species) are perennial plants belonging to the Leguminosae family [15]. Licorice is widely distributed in Asia, Europe, and the Americas. The licorice species, Glycyrhiza uralensis (G. uralensis) and G. glabra, are widely used in traditional Chinese medicine [15]. G. uralensis is found in Central Asia to the northeastern part of China and G. glabra is distributed from southern Europe to the northwestern part of China. These species are characterized by various pharmacological activities, including anti-oxidant, anti-inflammation, immune improvement, and anti-tumor effects [16171819]. Previous research has reported that licorice inhibits Aβ-induced neurotoxicity in a cellular model by modulating oxidant stress [20]. In addition, licorice contains glycyrrhizins, such as glycyrrhizic acid, and flavonoids, including liquiritin, liquiritigenin, and isoliquiritigenin [1621]. Glycyrrhizic acid is neuroprotective in different cognitive impairment-related cell and animal models via inhibition of oxidative and inflammatory damage [2223242526].
Shinwongam (SW), a new single licorice variety, was developed by the herbal crop research division at the National Institute of Crop Science (NICS), Rural Development Administration in Korea, by combining G. uralensis and G. glabra [27]. The glycyrrhizin content of SW is > 3.63% and its yield was twice as high as G. uralensis, which is most widely used in Traditional Chinese Medicine [2728]. To the best of our knowledge, no study has compared the effect of SW, G. uralensis, and G. glabra on cognition. Consequently, the present study assessed their effects on cognition in an LPS-induced inflammatory model.
SW, G. uralensis, and G. glabra were kindly provided by the herbal crop research division at the NICS (Eumseung, Korea). A voucher herbarium specimen has been deposited at the Korea Medicinal Resources Herbarium of the herbal crop research division. Dried slices of SW were extracted at room temperature for 72 h in 50% ethanol, and the solvent was evaporated in vacuo to create an extract with a yield of 18.5%. G. uralensis and G. glabra were also pulverized and extracted using the same method, giving extracts with yields of 12.6% and 18.3%, respectively.
Male C57BL/6 mice (7 weeks old, 19–21 g) were purchased from Orient Inc. (Seongnam, Korea). Mice were housed in plastic cages with a 12 h light-dark cycle. The room had standard laboratory humidity (50 ± 10%) and temperature (20 ± 2℃). During the experimental period, mice were provided with free access to a diet (5L79, Orient Inc., Seongnam, Korea), and water. All experimental procedures were permitted (Approval No. PNU-2017-1443) using the guidelines established by the Pusan National University Institutional Animal Care and Use Committee (PNU-IACUC).
Mice were separated into five groups (n = 6 in each group): Normal = 0.9% NaCl injected intraperitoneally + oral administration of water; Control = 2.5 mg/kg LPS (i.p.) + water (oral); GU = 2.5 mg/kg LPS (i.p.) + G. uralensis extract (150 mg/kg/day, oral); GB = 2.5 mg/kg LPS (i.p.) + G. glabra extract (150 mg/kg/day, oral); SW= 2.5 mg/kg LPS (i.p.) + SW extract (150 mg/kg/day, oral). We administered the licorice extracts orally by gastric gavage. LPS (Escherichia coli, serotype 055:B5, Sigma, St. Louis, MO, USA) was injected 3 times a week, and 4 h prior to behavior testing on the test day [2930]. The experimental schedule is shown in Fig. 1.
Spatial cognition ability was assessed by subjecting mice to a spontaneous T-maze test [31]. Briefly, the mice underwent a maze challenge with their natural tendency to enter the left or right routes. The mouse was placed in the T-maze day before performing the task (training day) and allowed to enter the left route for 2 min while the right route was blocked by the door. On the test day, the mouse was placed in the base arm of the T-maze and allowed to explore left (old route) or right (novel) route for 2 min. During this 2 min, both routes were open. Space perception (%) was evaluated as the ratio of right (novel) entries divided by the total number of arm entries, multiplied by 100.
This test was performed in a square black open-field apparatus (40 × 30 × 20 cm) [32]. Two identical objects (plastic bottles) were placed at fixed distances within the square field. The mice were then placed at the center of the square field, and the number of touches of each object was recorded during a 2-min period (training session). The mice were placed back into the same field at 24 h after the training session, but this time, one of the objects used during the training session was replaced with a new object (another plastic bottle). The mice were allowed to explore freely for 3 min, and the number of touches was recorded (test session). Object recognition (%) was calculated as a ratio of the amount of time spent exploring any one of the two original objects (training session) or the novel object (test session) divided by the total time spent exploring both objects.
This test was conducted according to the procedure established by Morris, with slight modifications [33]. The apparatus used in the Morris water maze test consisted of a dark plastic circular pool, 80 cm in diameter, surrounded by a 40 cm high wall, and randomly divided into quadrants. White poster color was added to the pool water to make it opaque, and the water temperature was maintained at 22 ± 1℃. An 8 cm diameter platform was placed 1 cm below the water surface in the middle of one quadrant. The position of the platform was unchanged during the training session. Four posters on the walls of the apparatus provided visual cues for navigation. Three training trials per day were conducted for 2 days. During the training trials, the mice were placed randomly in the water facing the pool wall and allowed to swim for a maximum of 60 s. The latency time required to find the platform was recorded. Mice that found the platform were allowed to rest there for 15 s. If they failed to find the platform within 60 s, they were placed on the platform for 15 s to help them remember. A probe trial of the Morris water maze test was performed 1 day after the 2 days of training. In the primary test, the experiment was performed as previously described. In the secondary test, the water was transparent and the number of times that it took the mouse to reach the platform, which was visible 1 cm above the surface of the water, was counted. Occupancy of the target quadrant (%) was calculated as the percentage of time spent in the target quadrant during a 60 s trial.
Brain tissues were rapidly removed and homogenized with RIPA buffer containing a protease inhibitor cocktail. The homogenates were centrifuged at 12,000 rpm (Combi 514R, Hanil Science Industrial Co., Ltd., Incheon, Korea) for 30 min at 4℃. After the supernatant was collected, the protein concentration was determined using a Bio-Rad protein assay kit. Equal amounts of total protein were separated by 10–13% sodium dodecyl sulfate polyacrylamide gel electrophoresis (SDS-PAGE). After electrophoresis, proteins were transferred to a polyvinylidene difluoride (PVDF) membrane (Millipore, MA, USA). Membranes were incubated with 5% skim milk dissolved in phosphate-buffered saline-tween 20 (PBS-T) for 60 min, followed by incubation with a primary antibody in PBS-T overnight at 4℃. The primary antibodies used were: TLR4, ß-actin (1:1000; Cell Signaling, Beverly, MA, USA), NF-κB p65 (phospho S536; 1:500; Abcam, Cambridge, UK), iNOS (1:2000; Calbiochem, Darmstadt, Germany), COX-2 (1:1000; Merk, Darmstadt, Germany), IL-6, and brain-derived neurotrophic factor (BDNF, 1:200; Santa Cruz, Texas, USA). In addition, the membranes were incubated with appropriate secondary antibodies (1:1000; Cell Signaling, Beverly, MA, USA) for 1 h. The immunocomplexes were visualized by pico-enhanced peroxidase detection (ELPIS Biotech, Daejeon, Korea), and bands were visualised with a Davinci-Chemiluminescent imaging system (CoreBio, Seoul, Korea).
Data are shown as mean ± standard deviation (SD). Data were assessed using one-way analysis of variance (ANOVA and Duncan's test for multiple comparisons. Student's t-test was used to compare groups in the T-maze and novel object recognition tests. Statistical significance was considered if P < 0.05.
The effects of the three varieties of licorice on spatial memory in LPS-induced cognitive deficient mice were shown in Fig. 2. In contrast to the non-LPS treated normal group, there was no significant difference in exploration of the old and novel route LPS-treated control group; however, G. uralensis- and SW-treated groups showed significantly increased numbers of entries into the novel vs old route (37.08% vs 62.92% and 35.42% vs 64.58%, respectively). These findings indicate that G. uralensis and SW alleviated the LPS-induced spatial memory deficit.
Fig. 3 shows the effect of three licorice varieties on object recognition following LPS-induced impairment. There was no significant change in the recognition of old and new objects in the LPS-injected control group, indicating that the injection of LPS led to cognitive dysfunction in object recognition. However, the non-LPS injected normal group spent a significantly higher percentage of time exploring of the novel object when compared with the familiar object (42.17% vs 57.83%). Similarly, administration of G. glabra and SW led to a significantly higher exploration of the novel object (41.88% vs 58.13% and 35.42% vs 64.58%, respectively). These findings suggest that the administration of G. glabra or SW can ameliorate LPS-induced object recognition impairment and SW is more effective than G. glabra.
We determined whether the LPS-induced long-term memory deficit was improved by the administration of licorice. The LPS-injected mice were trained to find the hidden platform for 2 days in a Morris water maze. On the final day, the LPS-injected mice group took a significantly longer time to reach the platform when compared with the normal group (Fig. 4). However, administration of G. uralensis, G. glabra, or SW reduced the time taken to find the hidden platform, although this did not reach statistical significance. In addition, we measured the time taken to reach the hidden and the exposed platforms on the final day of the water maze test. The LPS treated-control group showed a significantly increased latency time when compared with the non-treated normal group (Fig. 5A). However, administration of licorice reduced the time to reach the hidden platform. To exclude the influence of the visual or exercise ability of mice, we tested the latency to reach the exposed platform; however we found no statistically significant difference between the groups (Fig. 5B).
The effects of three varieties of licorice on pro-inflammatory proteins in the brain were shown in Fig. 6. The expression of the pro-inflammatory proteins, TLR4, NF-kB p65, iNOS, and COX-2 were significantly increased in the LPS-injected control group when compared with the normal group. LPS induced an inflammatory reaction in the brain; however, administration of G. uralensis, G. glabra, and SW suppressed pro-inflammation mediators. Furthermore, SW-administrated groups showed significantly attenuated expression of TLR4 and iNOS.
As show in Fig. 7, we investigated the effect of three licorice varieties on protein expression of inflammatory cytokines, IL-6, induced by LPS. The injection of LPS significantly up-regulated IL-6 protein expression, compared to the normal group. Furthermore, the administration of the three licorice varieties, GU, GB and SW groups, inhibited levels of IL-6.
We confirmed the effect of three licorice varieties on BDNF protein expression in the brain of LPS-induced mice. As shown in Fig. 8, the LPS-injected control group showed a decline of BDNF protein expression when compared with the normal group. Furthermore, BDNF expression was significantly up-regulated in the SW when compared with the other licorice treatments.
Neuroinflammation is a well-known cause of neurodegenerative disease, such as AD [6]. Aβ and NFTs are hallmarks in the AD brain. Furthermore, neuroinflammation can result in synaptic loss, neuronal cell death, and cognitive impairment [67]. Therefore, inflammation may play an important role in AD. Many studies have used inflammation-induced cognitive deficit models to search for protective and therapeutic agents against AD [67]. Intraperitoneal injection of LPS induces cognitive impairment in mice [810]. Several studies have determined the treatment of LPS increases inflammatory signals, accumulation of Aβ, and AD-like neuronal degeneration in the brain [91029]. Therefore, we investigated the protective effect of licorice and its mechanism underlying the amelioration of LPS-induced cognitive impairment in mice.
We confirmed the effect of licorice on spatial and novel object memory using the T-maze and novel objective recognition tests in the LPS-treated mice. These tests are widely used to evaluate cognitive ability, because the interests of mice are known to affect natural exploratory behaviors toward a novel route or object than old route or object [34]. Previous studies have shown that LPS-treated mice had reduced spatial memory in Y-maze test and the perception for a novel object in novel object recognition test [3536]. In our results, the G. uralensis and SW licorice-administered mice showed higher interest in the novel route or object when compared with the LPS-injected control group. Consequently, these results confirmed that G. uralensis and SW improve the LPS-induced impairment of spatial and novel object recognition memory.
To examine long-term cognitive ability, we conducted the Morris water maze test, which commonly used in rodents. LPS-injected mice showed learning and memory impairments in this test [37]; however, licorice administration led to a significant decrease in the time taken to reach the hidden platform during continuous training when compared with the LPS-induced control group. In addition, we evaluated the time taken to reach the exposed platform in each group. The results indicated that the protective activity from LPS-induced cognitive impairment was not related to visual or swimming abilities. Therefore, the present results suggested that administration of licorice improved long-term learning and memory in LPS-induced mice. It has been reported that licorice extract can improved spatial memory retention in behavioral tests, including the Morris water maze test [3839], which is consistent with our results. Therefore, we suggest that licorice may play a promising protective role against memory and cognitive deficits.
To elucidate the mechanisms underlying the protective effect of licorice on cognitive impairment, we measured the expression of different inflammatory proteins in the brain. TLR4 is activated by LPS and stimulates the NF-κB signaling pathway [8]. In addition, the NF-κB pathway releases inflammatory mediators, such as iNOS and COX-2, and increases inflammatory cytokines, such as IL-6, resulting in neuronal damage in the AD brain [640]. Previous reports have investigated LPS-injected cognitive impairment in mice and shown that there is an over-generation of iNOS, COX-2, and IL-6 by activating TLR4 and NF-kB [94142]. The present study showed that inflammation-related proteins were inhibited in licorice-administrated mice when compared with the LPS-treated group. In addition, licorice extract inhibited levels of LPS-stimulated pro-inflammatory mediators, such as nitric oxide, iNOS, COX-2, IL-1β, and IL-6 in vitro [4344]. Furthermore, the active compound in liquorice includes glycyrrhizin, liquiritin, and liquiritigenin, which have anti-inflammatory activities [4546].
BDNF is extensively expressed in the brain and plays an important role in the maintenance of the central nervous system, such as cognitive ability [47]. Studies have shown that BDNF is lower in the brains of AD patients when compared with healthy controls [474849]. In addition, neuroinflammation via LPS induces a significant decline in the expression of BDNF protein in the brain, resulting in cognitive dysfunction [50]. Previous studies suggest that the decline in BDNF in AD is associated with the activation of the NF-kB pathway in an LPS-injected mouse model [30]. We have confirmed this and further showed an increase in BDNF expression following treatment with three licorice varieties in LPS-induced mice. Therefore, we suggest that the improvement of cognitive ability following licorice treatment in LPS-injected mice is associated with the upregulation of BDNF.
In conclusion, we have investigated the protective effect of three varieties of licorice, G. uralensis, G. glabra, and SW, in LPS-induced cognitive impairment in mice. Licorice improved cognitive function, suppressed pro-inflammatory proteins, and enhanced the expression of BDNF. Furthermore, we found that SW, a new species of licorice in Korea, exerted a stronger anti-neuroinflammatory effect when compared with the other licorice extracts. These results suggest a beneficial role for the protection and therapeutic effect of licorice on cognitive loss, for example in AD or neuroinflammation.
References
1. Brookmeyer R, Johnson E, Ziegler-Graham K, Arrighi HM. Forecasting the global burden of Alzheimer's disease. Alzheimers Dement. 2007; 3:186–191. PMID: 19595937.


2. Price JL, Morris JC. Tangles and plaques in nondemented aging and “preclinical” Alzheimer's disease. Ann Neurol. 1999; 45:358–368. PMID: 10072051.


3. Butterfield DA. Amyloid beta-peptide (1-42)-induced oxidative stress and neurotoxicity: implications for neurodegeneration in Alzheimer's disease brain. A review. Free Radic Res. 2002; 36:1307–1313. PMID: 12607822.
4. Canevari L, Abramov AY, Duchen MR. Toxicity of amyloid beta peptide: tales of calcium, mitochondria, and oxidative stress. Neurochem Res. 2004; 29:637–650. PMID: 15038611.
5. Butterfield DA, Griffin S, Munch G, Pasinetti GM. Amyloid beta-peptide and amyloid pathology are central to the oxidative stress and inflammatory cascades under which Alzheimer's disease brain exists. J Alzheimers Dis. 2002; 4:193–201. PMID: 12226538.
6. DeLegge MH, Smoke A. Neurodegeneration and inflammation. Nutr Clin Pract. 2008; 23:35–41. PMID: 18203962.


7. Saijo K, Winner B, Carson CT, Collier JG, Boyer L, Rosenfeld MG, Gage FH, Glass CK. A Nurr1/CoREST pathway in microglia and astrocytes protects dopaminergic neurons from inflammation-induced death. Cell. 2009; 137:47–59. PMID: 19345186.


8. Lukiw WJ. Bacteroides fragilis lipopolysaccharide and inflammatory signaling in Alzheimer's disease. Front Microbiol. 2016; 7:1544. PMID: 27725817.


9. Qin L, Wu X, Block ML, Liu Y, Breese GR, Hong JS, Knapp DJ, Crews FT. Systemic LPS causes chronic neuroinflammation and progressive neurodegeneration. Glia. 2007; 55:453–462. PMID: 17203472.


10. Rosi S, Vazdarjanova A, Ramirez-Amaya V, Worley PF, Barnes CA, Wenk GL. Memantine protects against LPS-induced neuroinflammation, restores behaviorally-induced gene expression and spatial learning in the rat. Neuroscience. 2006; 142:1303–1315. PMID: 16989956.


11. Hanke ML, Kielian T. Toll-like receptors in health and disease in the brain: mechanisms and therapeutic potential. Clin Sci (Lond). 2011; 121:367–387. PMID: 21745188.


12. Café-Mendes CC, Garay-Malpartida HM, Malta MB, de Sá Lima L, Scavone C, Ferreira ZS, Markus RP, Marcourakis T. Chronic nicotine treatment decreases LPS signaling through NF-κB and TLR-4 modulation in the hippocampus. Neurosci Lett. 2017; 636:218–224. PMID: 27984197.


13. Ray B, Lahiri DK. Neuroinflammation in Alzheimer's disease: different molecular targets and potential therapeutic agents including curcumin. Curr Opin Pharmacol. 2009; 9:434–444. PMID: 19656726.


14. Jain NK, Patil CS, Kulkarni SK, Singh A. Modulatory role of cyclooxygenase inhibitors in aging- and scopolamine or lipopolysaccharide-induced cognitive dysfunction in mice. Behav Brain Res. 2002; 133:369–376. PMID: 12110471.


15. Liao WC, Lin YH, Chang TM, Huang WY. Identification of two licorice species, Glycyrrhiza uralensis and Glycyrrhiza glabra, based on separation and identification of their bioactive components. Food Chem. 2012; 132:2188–2193.


16. Hosseinzadeh H, Nassiri-Asl M. Pharmacological effects of Glycyrrhiza spp. and its bioactive constituents: update and review. Phytother Res. 2015; 29:1868–1886. PMID: 26462981.
17. Yang R, Yuan BC, Ma YS, Zhou S, Liu Y. The anti-inflammatory activity of licorice, a widely used Chinese herb. Pharm Biol. 2017; 55:5–18. PMID: 27650551.


18. Barfod L, Kemp K, Hansen M, Kharazmi A. Chalcones from Chinese liquorice inhibit proliferation of T cells and production of cytokines. Int Immunopharmacol. 2002; 2:545–555. PMID: 11962733.


19. Yo YT, Shieh GS, Hsu KF, Wu CL, Shiau AL. Licorice and licochalcone-A induce autophagy in LNCaP prostate cancer cells by suppression of Bcl-2 expression and the mTOR pathway. J Agric Food Chem. 2009; 57:8266–8273. PMID: 19711916.


20. Lee HK, Yang EJ, Kim JY, Song KS, Seong YH. Inhibitory effects of Glycyrrhizae radix and its active component, isoliquiritigenin, on Aβ(25-35)-induced neurotoxicity in cultured rat cortical neurons. Arch Pharm Res. 2012; 35:897–904. PMID: 22644857.


21. Nomura T, Fukai T. Phenolic constituents of licorice (Glycyrrhiza species). Fortschr Chem Org Naturst. 1998; 73:1–158. PMID: 9545874.


22. Ojha S, Javed H, Azimullah S, Abul Khair SB, Haque ME. Glycyrrhizic acid attenuates neuroinflammation and oxidative stress in rotenone model of Parkinson's disease. Neurotox Res. 2016; 29:275–287. PMID: 26607911.


23. Cherng JM, Lin HJ, Hung MS, Lin YR, Chan MH, Lin JC. Inhibition of nuclear factor κB is associated with neuroprotective effects of glycyrrhizic acid on glutamate-induced excitotoxicity in primary neurons. Eur J Pharmacol. 2006; 547:10–21. PMID: 16952351.


24. Song JH, Lee JW, Shim B, Lee CY, Choi S, Kang C, Sohn NW, Shin JW. Glycyrrhizin alleviates neuroinflammation and memory deficit induced by systemic lipopolysaccharide treatment in mice. Molecules. 2013; 18:15788–15803. PMID: 24352029.


25. Akman T, Guven M, Aras AB, Ozkan A, Sen HM, Okuyucu A, Kalkan Y, Sehitoglu I, Silan C, Cosar M. The neuroprotective effect of glycyrrhizic acid on an experimental model of focal cerebral ischemia in rats. Inflammation. 2015; 38:1581–1588. PMID: 25687639.


26. Guo J, Yang CX, Yang JJ, Yao Y. Glycyrrhizic acid ameliorates cognitive impairment in a rat model of vascular dementia associated with oxidative damage and inhibition of voltage-gated sodium channels. CNS Neurol Disord Drug Targets. 2016; 15:1001–1008. PMID: 27238153.
27. National Institute of Horticultural and Herbal Science (KR). 2014 Annual Reports. Wanju: National Institute of Horticultural and Herbal Science;2014. p. 58–59.
28. Wang X, Zhang H, Chen L, Shan L, Fan G, Gao X. Liquorice, a unique “guide drug” of traditional Chinese medicine: a review of its role in drug interactions. J Ethnopharmacol. 2013; 150:781–790. PMID: 24201019.


29. Lee JW, Lee YK, Yuk DY, Choi DY, Ban SB, Oh KW, Hong JT. Neuro-inflammation induced by lipopolysaccharide causes cognitive impairment through enhancement of beta-amyloid generation. J Neuroinflammation. 2008; 5:37. PMID: 18759972.


30. Gu SM, Park MH, Hwang CJ, Song HS, Lee US, Han SB, Oh KW, Ham YW, Song MJ, Son DJ, Hong JT. Bee venom ameliorates lipopolysaccharide-induced memory loss by preventing NF-kappaB pathway. J Neuroinflammation. 2015; 12:124. PMID: 26112466.


31. Montgomery KC. A test of two explanations of spontaneous alternation. J Comp Physiol Psychol. 1952; 45:287–293. PMID: 14946277.


32. Bevins RA, Besheer J. Object recognition in rats and mice: a one-trial non-matching-to-sample learning task to study ‘recognition memory’. Nat Protoc. 2006; 1:1306–1311. PMID: 17406415.


33. Morris R. Developments of a water-maze procedure for studying spatial learning in the rat. J Neurosci Methods. 1984; 11:47–60. PMID: 6471907.


34. Montgomery KC. Exploratory behavior and its relation to spontaneous alternation in a series of maze exposures. J Comp Physiol Psychol. 1952; 45:50–57. PMID: 14907933.


35. Lee YJ, Choi DY, Choi IS, Kim KH, Kim YH, Kim HM, Lee K, Cho WG, Jung JK, Han SB, Han JY, Nam SY, Yun YW, Jeong JH, Oh KW, Hong JT. Inhibitory effect of 4-O-methylhonokiol on lipopolysaccharide-induced neuroinflammation, amyloidogenesis and memory impairment via inhibition of nuclear factor-kappaB in vitro and in vivo models. J Neuroinflammation. 2012; 9:35. PMID: 22339795.


36. Miwa M, Tsuboi M, Noguchi Y, Enokishima A, Nabeshima T, Hiramatsu M. Effects of betaine on lipopolysaccharide-induced memory impairment in mice and the involvement of GABA transporter 2. J Neuroinflammation. 2011; 8:153. PMID: 22053950.


37. Sparkman NL, Martin LA, Calvert WS, Boehm GW. Effects of intraperitoneal lipopolysaccharide on Morris maze performance in year-old and 2-month-old female C57BL/6J mice. Behav Brain Res. 2005; 159:145–151. PMID: 15795008.


38. Sharifzadeh M, Shamsa F, Shiran S, Karimfar MH, Miri AH, Jalalizadeh H, Gholizadeh S, Salar F, Tabrizian K. A time course analysis of systemic administration of aqueous licorice extract on spatial memory retention in rats. Planta Med. 2008; 74:485–490. PMID: 18404595.


39. Dhingra D, Parle M, Kulkarni SK. Memory enhancing activity of Glycyrrhiza glabra in mice. J Ethnopharmacol. 2004; 91:361–365. PMID: 15120462.


40. Rubio-Perez JM, Morillas-Ruiz JM. A review: inflammatory process in Alzheimer's disease, role of cytokines. ScientificWorldJournal. 2012; 2012:756357. PMID: 22566778.


41. Borowski T, Kokkinidis L, Merali Z, Anisman H. Lipopolysaccharide, central in vivo biogenic amine variations, and anhedonia. Neuroreport. 1998; 9:3797–3801. PMID: 9875707.


42. Lukiw WJ, Bazan NG. Strong nuclear factor-kappaB-DNA binding parallels cyclooxygenase-2 gene transcription in aging and in sporadic Alzheimer's disease superior temporal lobe neocortex. J Neurosci Res. 1998; 53:583–592. PMID: 9726429.
43. Thiyagarajan P, Chandrasekaran CV, Deepak HB, Agarwal A. Modulation of lipopolysaccharide-induced pro-inflammatory mediators by an extract of Glycyrrhiza glabra and its phytoconstituents. Inflammopharmacology. 2011; 19:235–241. PMID: 21328091.


44. Wu TY, Khor TO, Saw CL, Loh SC, Chen AI, Lim SS, Park JH, Cai L, Kong AN. Anti-inflammatory/Anti-oxidative stress activities and differential regulation of Nrf2-mediated genes by non-polar fractions of tea Chrysanthemum zawadskii and licorice Glycyrrhiza uralensis. AAPS J. 2011; 13:1–13. PMID: 20967519.


45. Schröfelbauer B, Raffetseder J, Hauner M, Wolkerstorfer A, Ernst W, Szolar OH. Glycyrrhizin, the main active compound in liquorice, attenuates pro-inflammatory responses by interfering with membrane-dependent receptor signalling. Biochem J. 2009; 421:473–482. PMID: 19442240.


46. Yu JY, Ha JY, Kim KM, Jung YS, Jung JC, Oh S. Anti-Inflammatory activities of licorice extract and its active compounds, glycyrrhizic acid, liquiritin and liquiritigenin, in BV2 cells and mice liver. Molecules. 2015; 20:13041–13054. PMID: 26205049.
47. Bekinschtein P, Cammarota M, Katche C, Slipczuk L, Rossato JI, Goldin A, Izquierdo I, Medina JH. BDNF is essential to promote persistence of long-term memory storage. Proc Natl Acad Sci U S A. 2008; 105:2711–2716. PMID: 18263738.


48. Saura CA, Valero J. The role of CREB signaling in Alzheimer's disease and other cognitive disorders. Rev Neurosci. 2011; 22:153–169. PMID: 21476939.


49. Song JH, Yu JT, Tan L. Brain-derived neurotrophic factor in Alzheimer's disease: risk, mechanisms, and therapy. Mol Neurobiol. 2015; 52:1477–1493. PMID: 25354497.


50. Schnydrig S, Korner L, Landweer S, Ernst B, Walker G, Otten U, Kunz D. Peripheral lipopolysaccharide administration transiently affects expression of brain-derived neurotrophic factor, corticotropin and proopiomelanocortin in mouse brain. Neurosci Lett. 2007; 429:69–73. PMID: 17976910.


Fig. 1
Experimental schedule
Mice were orally administered with licorice extracts, including G. uralensis, G. glabra, and SW at concentration of 150 mg/kg/day for 7 days. 4 h prior to behavioral testing, mice received LPS injections (2.5 mg/kg/day, i.p., 3 times a week). LPS: Lipopolysaccharide
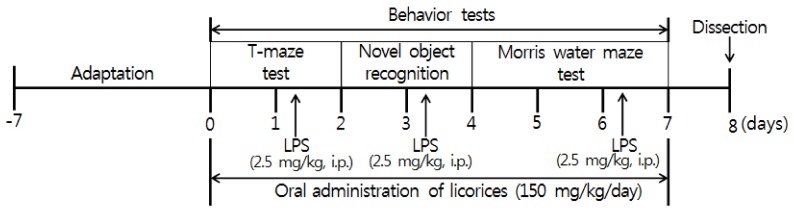
Fig. 2
The effects of licorice extracts on spatial memory, measured by the T-maze test
Data are presented as mean ± SD. * Means the space perception route cognitive abilities for familiar and novel routes are significantly different (P < 0.05) as determined by Student's t-test (n = 6 mice per group). Normal, 0.9% injection + oral administration of water; Control, LPS injection + oral administration of water; GU, LPS injection + oral administration G. uralensis (150 mg/kg/day); GB, LPS injection + oral administration G. glabra (150 mg/kg/day); SW, LPS injection + oral administration SW (150 mg/kg/day).
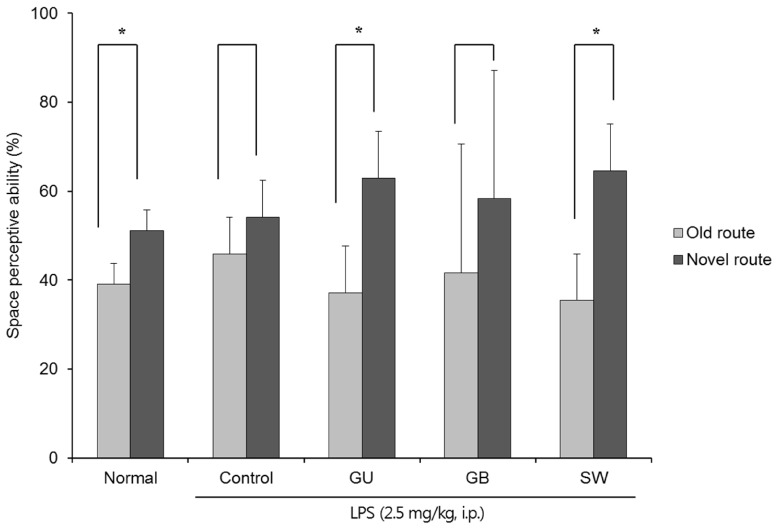
Fig. 3
The effects of licorice extracts on recognition memory in the novel object recognition test
Data are presented as mean ± SD. * Means of the space perception route cognitive abilities for familiar and novel routes are significantly different (P < 0.05) as determined by Student's t-test (n = 6 mice per group). Normal, 0.9% injection + oral administration of water; Control, LPS injection + oral administration of water; GU, LPS injection + oral administration G. uralensis (150 mg/kg/day); GB, LPS injection + oral administration G. glabra (150 mg/kg/day); SW, LPS injection + oral administration SW (150 mg/kg/day).
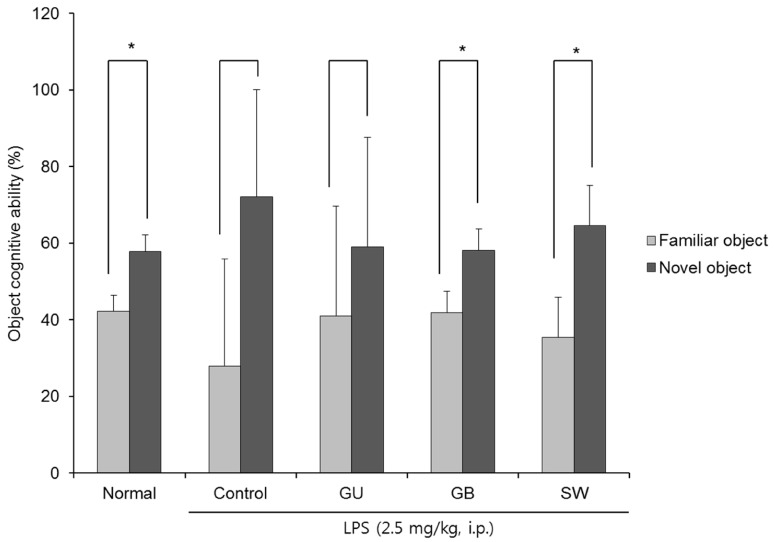
Fig. 4
The effects of licorice extracts on escape latency to the platform in the Morris water maze test
Data are presented as mean ± SD. a–b Means with different letters are significantly different (P < 0.05) as determined by Duncan's multiple tests (n = 6 mice per group). Normal, 0.9% injection + oral administration of water; Control, LPS injection + oral administration of water; GU, LPS injection + oral administration G. uralensis (150 mg/kg/day); GB, LPS injection + oral administration G. glabra (150 mg/kg/day); SW, LPS injection + oral administration SW (150 mg/kg/day).
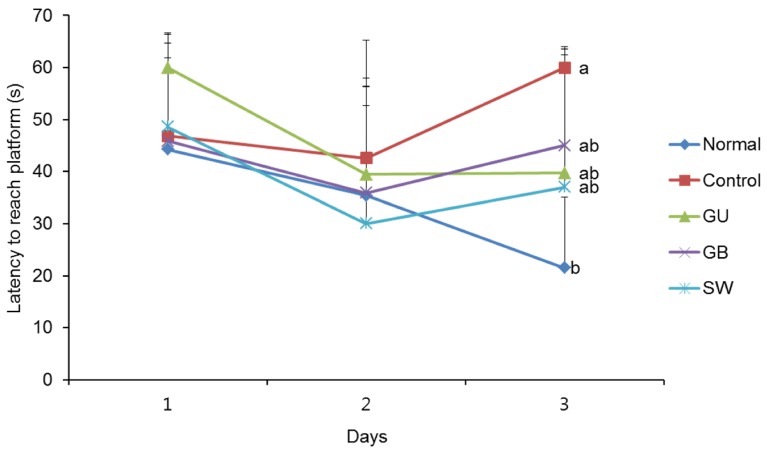
Fig. 5
The effects of licorice extracts on escape latency to reach a hidden platform (A) and exposed platform (B) in the Morris water maze test
Data are presented as mean ± SD. a–b Means with different letters are significantly different (P < 0.05) as determined by Duncan's multiple tests. NS indicates no significant differences among experimental groups (n = 6 mice per group). Normal, 0.9% injection + oral administration of water; Control, LPS injection + oral administration of water; GU, LPS injection + oral administration G. uralensis (150 mg/kg/day); GB, LPS injection + oral administration G. glabra (150 mg/kg/day); SW, LPS injection + oral administration SW (150 mg/kg/day).
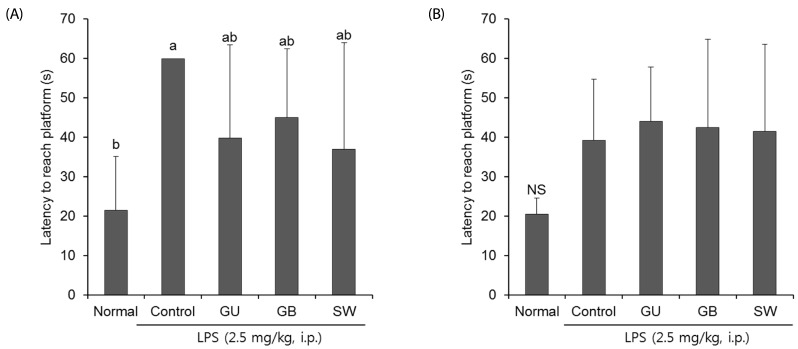
Fig. 6
The effects of licorice extracts on inflammation-related protein expressions including TLR4, NF-κB p65, iNOS and COX-2 in LPS-injected mice brain
Data are presented as mean ± SD. a–e Means with different letters are significantly different (P < 0.05) as determined by Duncan's multiple tests. Normal, 0.9% injection + oral administration of water; Control, LPS injection + oral administration of water; GU, LPS injection + oral administration G. uralensis (150 mg/kg/day); GB, LPS injection + oral administration G. glabra (150 mg/kg/day); SW, LPS injection + oral administration SW (150 mg/kg/day).
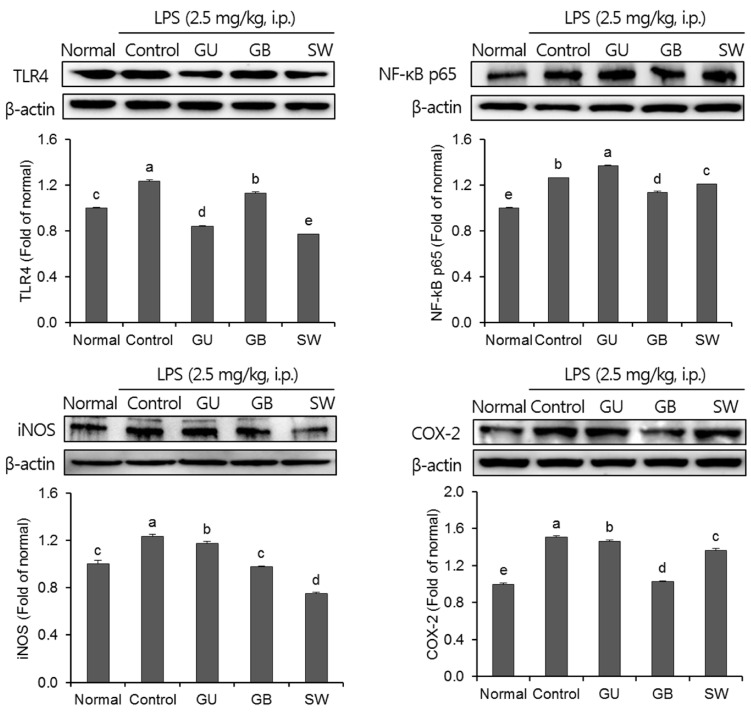
Fig. 7
The effects of licorice extracts on IL-6 protein expressions in LPS-injected mice brain
Data are presented as mean ± SD. a–e Means with different letters are significantly different (P < 0.05) as determined by Duncan's multiple tests. Normal, 0.9% injection + oral administration of water; Control, LPS injection + oral administration of water; GU, LPS injection + oral administration G. uralensis (150 mg/kg/day); GB, LPS injection + oral administration G. glabra (150 mg/kg/day); SW, LPS injection + oral administration SW (150 mg/kg/day).
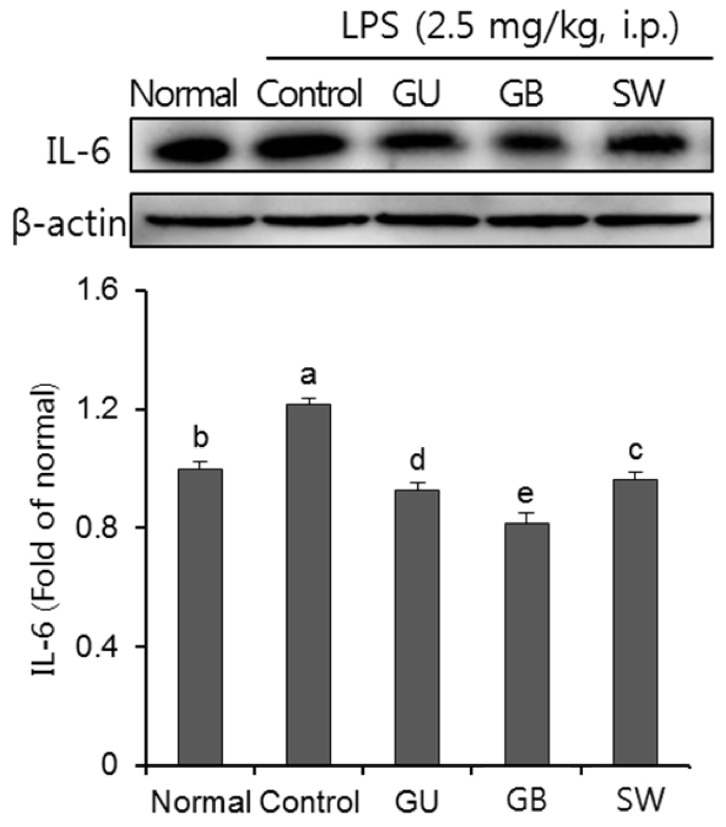
Fig. 8
The effects of licorice extracts on BDNF protein expressions in LPS-injected mice brain
Data are presented as mean ± SD. a–e Means with different letters are significantly different (P < 0.05) as determined by Duncan's multiple tests. Normal, 0.9% injection + oral administration of water; Control, LPS injection + oral administration of water; GU, LPS injection + oral administration G. uralensis (150 mg/kg/day); GB, LPS injection + oral administration G. glabra (150 mg/kg/day); SW, LPS injection + oral administration SW (150 mg/kg/day).
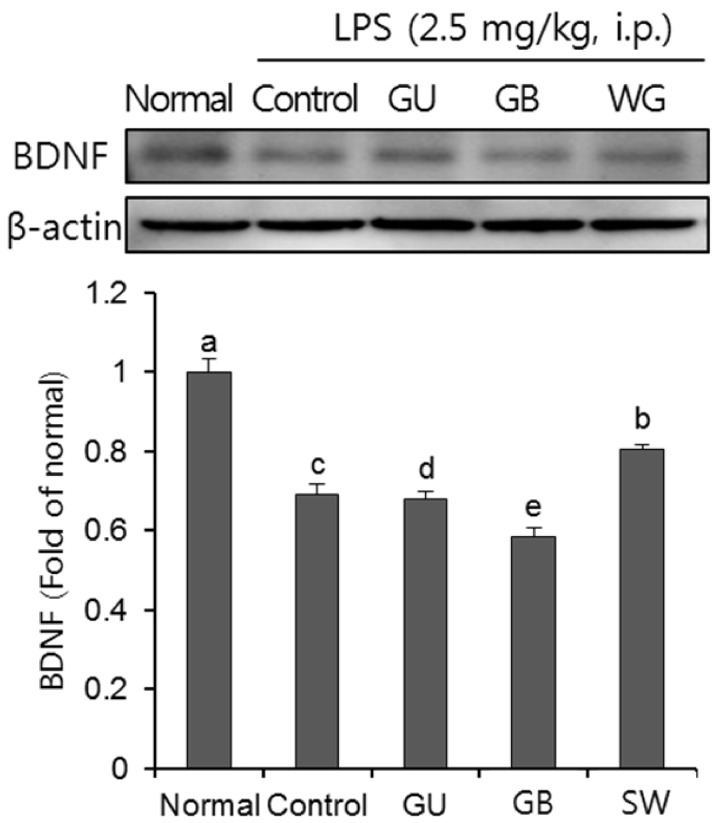