Abstract
BACKGROUND/OBJECTIVE
This study was designed to investigate how a Portulaca oleracea L. extract (POE) stimulates insulin secretion in INS-1 pancreatic β-cells.
MATERIALS/METHOD
INS-1 pancreatic β-cells were incubated in the presence of various glucose concentrations: 1.1 or 5.6, 16.7 mM glucose. The cells were treated with insulin secretagogues or insulin secretion inhibitor for insulin secretion assay using an insulin ELISA kit. In order to quantify intracellular influx of Ca2+ caused by POE treatment, the effect of POE on intracellular Ca2+ in INS-1 pancreatic β-cells was examined using Fluo-2 AM dye.
RESULTS
POE at 10 to 200 µg/mL significantly increased insulin secretion dose-dependently as compared to the control. Experiments at three glucose concentrations (1.1, 5.6, and 16.7 mM) confirmed that POE significantly stimulated insulin secretion on its own as well as in a glucose-dependent manner. POE also exerted synergistic effects on insulin secretion with secretagogues, such as L-alanine, 3-isobutyl-1-methylxanthine, and especially tolbutamide, and at a depolarizing concentration of KCl. The insulin secretion caused by POE was significantly attenuated by treatment with diazoxide, an opener of the K+ATP channel (blocking insulin secretion) and by verapamil (a Ca2+ channel blocker). The insulinotropic effect of POE was not observed under Ca2+-free conditions in INS-1 pancreatic β-cells. When the cells were preincubated with a Ca2+ fluorescent dye, Fluo-2 (acetoxymethyl ester), the cells treated with POE showed changes in fluorescence in red, green, and blue tones, indicating a significant increase in intracellular Ca2+, which closely correlated with increases in the levels of insulin secretion.
Insulin is a hormone secreted by pancreatic β-cells at a high blood glucose concentration. Insulin promotes absorption of glucose from blood by the liver and by adipose and skeletal muscle cells. A deficiency of insulin in diabetes leads to critical dysfunction in the target tissues [12]. The insulin secretion process in pancreatic β-cells functions as follows: when glucose concentration increases, glucose is translocated into pancreatic β-cells through glucose transport 2 and the ATP produced by glucose metabolism increases the intracellular ATP/ADP ratio. The increased amount of ATP causes cell membrane depolarization by blocking the pore channel that is a part of a K+ATP channel-dependent pathway. The membrane voltage changes due to depolarization, thus opening the voltage-gated Ca2+ channel, which is sensitive to the potential difference, and promotes intracellular influx of Ca2+. Increased intracellular Ca2+ concentration is known to increase insulin secretion by stimulating exocytosis in pancreatic β-cells [34].
In the prevention and treatment of type 2 diabetes, promoting insulin secretion is important for reducing hyperglycemia [5]. Sulfonylureas, insulin secretion-promoting drugs, interact with sulfonylurea receptor 1 (SUR1) to induce depolarization of the cell membrane by blocking the K+ATP channel-dependent pathway. This is a pharmacological action that promotes insulin secretion to lower the blood glucose level by driving an influx of Ca2+ into the cell [6]. Nonetheless, the consecutive use of synthetic drugs should be limited because these drugs may cause side effects such as hypoglycemia, gradual destruction of pancreatic β-cells, and endothelial-cell dysfunction [7]. Thus, recent studies have been carried out to find a natural product in herbs-to stimulate insulin secretion-that does not exert any side effects [89].
The genus Portulaca is an annual plant belonging to the family Portulacaceae. P. oleracea is widely distributed in temperate and tropical areas of the world and is known to be rich in alkaloids, terpenoids, coumarins, and flavonoids [1011]. A P. oleracea extract has various bioactivities such as antioxidant [12], hyperlipidemia [13], anti-inflammation [14], neuroprotective effects [15] and anti-hyperglycemia [16]. Although P. oleracea extract has shown an effect on anti-hyperglycemia, there was not any research to investigate the mechanism by which the effect appears. Especially, no research has been conducted to evaluate the effect of a P. oleracea extract on insulin secretion via a K+ATP channel dependent pathway in INS-1 pancreatic β-cells. Therefore, this study evaluated the effect of a P. oleracea extract on insulin secretion and the mechanism of its insulinotropic effect in pancreatic β-cells. In an experiment on insulin secretion, three different glucose concentrations were used as basal glucose (1.1 mM glucose), which is non-stimulatory glucose concentration, substimulatory glucose (5.6 mM) and stimulatory glucose (16.7 mM) concentration to investigate the effect of insulin secretion and whether the effect was glucose-dependent or not [17181920]. This study also used three different glucose concentration to evaluate the effect of P. oleracea extract on insulin secretion.
P. oleracea L. specimens were purchased from Hyosung Food Inc. (Hongcheon, Korea) in March 2017. These specimens were dried in a freeze-drying apparatus and ground into a fine powder. The powder was extracted with 10 volumes of 80% ethanol for 3 days at room temperature with daily changes of ethanol. This P. oleracea extract (POE) was filtered and concentrated to dryness in a rotary vacuum evaporator. After the extract was thoroughly dried for removal of the solvent, the dry powder was stored in a freezer (−80℃).
These cells were maintained in the RPMI 1640 medium supplemented with 10% of fetal bovine serum (FBS), streptomycin and penicillin (100 units/mL and 100 µg/mL, respectively) at 37℃ in a humidified atmosphere containing 5% of CO2 via transfer to new culture plates every 2 days. All the cell culture mediums were purchased from Hyclone (Hyclone, Logan, UT, USA).
Cell viability was assayed using a 3-(4, 5-dimethylthiazol-2-yl)-2, 5-diphenyltetrazolium bromide (MTT, Sigma, St. Louis, MO, USA) in a colorimetric MTT assay. INS-1 pancreatic β-cells (2 × 104/well) in a 96-well microplate were preincubated with glucose (1.1 or 16.7 mM) for 24 h. After that, the medium was replaced with the medium containing various concentrations of POE-0, 0.1, 0.2, 0.5, 1.0, or 2.0 mg/mL-with incubation for 24 h. An MTT solution (100 µL) was added into each well, then incubated for 4 h. When MTT is incubated with live cells, MTT is reduced by mitochondrial reductase to crystals of formazan. We added 100 µL of DMSO into each well and shook the plate for 15–20 min on a plate shaker. Next, we measured the absorbance at 540 nm on a microplate reader. This absorbance represents the amount of MTT reduced by the cells and is proportional to the number of viable cells in each well.
The INS-1 pancreatic β-cells in the subculture were collected and seeded at 2 × 104/well in a 96-well plate and incubated for 24 h at 37℃ in a 5% CO2 incubator to stabilize them. After cultivation, the medium was removed and the cells were washed twice with phosphate-buffered saline. To identify the mechanism of insulin secretion, INS-1 pancreatic β-cells were incubated in the presence or absence of POE under the following conditions, in the presence of various glucose concentrations: 1.1, 5.6, or 16.7 mM glucose. To measure the synergistic effect of POE on insulin secretion by INS-1 pancreatic β-cells, the cells were simultaneously incubated with L-alanine (10 mM, insulin secretion promoter), 3-isobutyl-1-methylxanthine (IBMX, 100 µM, β-cell K+ATP channel blocker), or tolbutamide (200 µM, cAMP activator). To determine whether insulin secretion is dependent on the K+ATP channel in INS-1 pancreatic β-cells, the cells were incubated with KCl (30 mM), verapamil (50 mM), or diazoxide (300 µM). The respective test compounds were added to Kreb's-Ringer bicarbonate (KRB buffer; 118 mM NaCl, 4.7 mM KCl, 2.5 mM CaCl2, 1.18 mM KH2PO4, 1.18 mM MgSO4, 25 mM NaHCO3, 10 mM HEPES, 0.1% BSA, pH 7.4), and then cultured for 1 h. After incubation, the supernatant was collected and centrifuged to completely eliminate the cells, and the insulin secretory function was measured by means of an insulin ELISA kit (Lingo Research, MO, USA). For the experiments, all reagents were purchased from Sigma-Aldrich (Sigma, St. Louis, MO, USA).
INS-1 pancreatic β-cells were cultured on glass slides for 24 h. The cells were treated with various concentration of glucose (1.1, 5.6, or 16.7 mM) for 48 h. The cells were loaded for 45 min at 37℃ with 1 µM Fluo-2 AM (Thermo Fisher Scientific, Waltham, MA, USA) in Hanks' Balanced Salt solution (HBSS) supplemented with streptomycin at 10 µg/mL and BSA at 0.1%, washed with the same medium (2 volumes), and allowed to equilibrate. The fluorescence was measured at 340-nm excitation and 380-nm emission using a confocal microscope (Zeiss LSM510NL0) with laser scanning. The images were acquired every second at an exposure time of 20 ms for 5–7 min. POE (200 µg/mL) or KCl (30 mM) were applied before acquisition of images in each sequence. The image sequences were analyzed in the ImageJ software (NIH, Bethesda, Maryland, USA). The position of cells on the coverslip was checked by overlaying the fluorescence and phase-contrast images. For the confocal microscope the following settings were used: × 40/1.30 oil Leitz Fluotar objective lens, excitation wavelength 488 nm, and a long-pass 515-nm emission filter [21]. The relative fluorescence changes (ΔF/Fo) were plotted as a function of time and integrated to determine the area under the curve [22].
The data are presented as mean ± standard deviation (SD). Statistical analysis was performed in the SAS software, version 9.1 (SAS Institute Inc., Cary, NC, USA). Student's t-test was employed for comparisons between the control and sample groups. Differences were evaluated by one-way analysis of variance (ANOVA), followed by post hoc Duncan's multiple-range test (with significance at P < 0.05).
To test whether POE exerts toxic effects on pancreatic β-cells, INS-1 pancreatic β-cells were treated with POE, and cell viability was measured by the MTT assay (Fig. 1). POE did not exert any cytotoxic effects at concentrations 0.1 to 2.0 mg/mL, indicating that the effects of POE on insulin secretion were not attributable to its toxicity.
To investigate the insulinotropic effect of POE and to determine whether the effect is glucose-dependent, the effects of POE (10 to 200 µg/mL) on insulin secretion were evaluated at 1.1 mM, 5.6 mM, or 16.7 mM in INS-1 pancreatic β-cells. Insulin secretion levels were 1.69, 2.02, and 2.26 ng per 3 × 105 cells per hour at glucose concentrations 1.1, 5.6, and 16.7 mM, respectively, without POE treatment. POE treatment at 10–200 µg/mL dose-dependently increased insulin secretion to 1.76–2.89 ng per 3 × 105 cells per hour at glucose concentration 1.1 mM, to 3.23-7.78 ng per 3 × 105 cells per hour at glucose concentration 5.6 mM, and to 3.61–12.55 ng per 3 × 105 cells per hour at glucose concentration 16.7 mM (Fig. 2). POE treatment at a concentration as low as 10 µg/mL in the cells stimulated insulin secretion although a maximal increase was observed at 200 µg/mL of POE. These data suggested that POE significantly stimulated insulin secretion in a glucose-dependent manner.
To identify the mechanism of the insulinotropic effect of POE, the K+ATP channel pathway was analyzed in the presence of an insulin secretion inhibitor in INS-1 pancreatic β-cells. The cells were treated with 50 mM verapamil (a Ca2+ channel blocker) or 300 µM diazoxide (an opener of the K+ATP channel), which binds to sulfonylurea receptor and opens the K+ATP channel to block the entry of Ca2+ into the cell. Verapamil or diazoxide treatment induced a significant reduction in insulin secretion at all glucose concentrations (P < 0.05; Fig. 3). Treatment with verapamil or diazoxide along with POE significantly decreased insulin secretion to 80.62% and 50.17% at 1.1 mM glucose, to 53.98% and 47.30% at 5.6 mM glucose, and to 46.69% and 32.59% at 16.7 mM glucose, respectively, as compared to the no-treatment control. This result indicated that the K+ATP channel may represent the main pathway of insulin secretion induced by POE.
To confirm that the K+ATP channel represented the main pathway of insulin secretion under the influence of POE, the effect of POE on insulin secretion was examined at a high concentration of K+ that induced membrane depolarization. The depolarizing concentration of KCl (30 mM) significantly increased insulin secretion at low or high glucose concentrations (Fig. 4). Insulin secretion in the presence of 30 mM KCl significantly increased to 6.02, 8.37, or 10.62 ng per 3 × 105 cells per hour at glucose concentrations 1.1, 5.6, and 16.7 mM, respectively. The treatment with POE along with 30 mM KCl significantly enhanced insulin secretion: to 11.44, 16.14, or 18.40 ng per 3 × 105 cells per hour at glucose concentrations 1.1, 5.6, and 16.7 mM, respectively. The combination of POE and KCl significantly increased insulin secretion: 1.90-, 1.92-, and 1.73-fold, respectively, as compared to KCl alone (P < 0.05).
The insulinotropic effects of POE in the presence of insulin secretagogues were investigated at glucose concentrations of 1.1, 5.6, and 16.7 mM. Treatment with POE along with L-alanine, tolbutamide, or IBMX increased insulin secretion 2.32-, 2.45-, and 2.51-fold as compared to the no-treatment control at 1.1 mM glucose (Fig. 5A), 2.01-, 3.73-, and 2.27-fold at 5.6 mM glucose (Fig. 5B), and 1.50-, 2.81-, and 1.22-fold at 16.7 mM glucose (Fig. 5C), respectively. POE significantly potentiated the insulinotropic effects of insulin secretagogues. Thus, a synergistic effect of the combination of POE with L-alanine, tolbutamide, or IBMX on the insulin secretion was observed. The increase in insulin secretion was most significantly enhanced by the combination of POE with tolbutamide, which is a K+ATP channel blocker.
An influx of extracellular Ca2+, which occurred after blocking of the K+ATP channel pathway and subsequent opening of the Ca2+ channel, plays an important role in stimulation of the insulin release by β-cells. To determine whether the insulinotropic effect of POE was due to an influx of extracellular Ca2+, the cells were treated with EGTA in KRB buffer, which provoked Ca2+ depletion in the cells. Insulin secretion was significantly enhanced in the presence of Ca2+ as the concentration of POE and glucose increased (Fig. 6). In contrast, the removal of Ca2+ from the extracellular fluid significantly decreased the insulinotropic effect of POE (P < 0.05). This finding indicated that the insulinotropic effect of POE is due to the Ca2+ influx from the extracellular fluid.
To quantify the intracellular influx of Ca2+ caused by POE treatment, we used the Fluo-2 AM dye that is widely employed to measure intracellular concentrations of Ca2+. The fluorescence of intracellular Ca2+ in INS-1 pancreatic β-cells treated with POE or KCl is shown in Fig. 7A. The vertical scale bar indicates the increase in intracellular Ca2+ concentration due to the treatments (Fig. 7B). The treatment with POE (200 µg/mL) significantly increased influx of intracellular Ca2+ to 225.82%, 282.41%, and 498.35% at glucose concentrations 1.1, 5.6, and 16.7 mM, respectively. Treatment with POE along with 16.7 mM glucose increased the vertical scale bar 4.9-fold over the control. The 16.7 mM glucose with 30 mM KCl treatment (positive control) induced intracellular Ca2+ influx by depolarization and showed a significant increase in Ca2+ concentration in the cells.
Type 2 diabetes is a disease in which hyperglycemia is caused by insulin secretion deficiency or insulin resistance [23]. Insulin is a key hormone alleviating hyperglycemia [2425]. The use of insulin secretagogues for the treatment of diabetes is common because of the ease of use and their ability to lower blood glucose levels [26]. Nevertheless, these agents promote insulin secretion even at low blood glucose levels, with the risk of hypoglycemic shock [27]. Accordingly various studies have been conducted to find a natural substance of plant origin for stimulation of insulin secretion without any side effects [28].
P. oleracea is an annual plant, which belongs to the family Portulacaceae and traditionally is consumed as a medicinal food by patients with diabetes [29]. A P. oleracea extract is reported to have various physiological activities such as an antihyperlipidemia and lipolysis effects [3031], and nephroprotective action [3233]. It is also reported to reduce the body weight, improve the impaired glucose tolerance and lipid metabolism and elevate insulin sensitivity [34]. However, its effect on insulin secretion via a K+ATP channel-dependent pathway is not known yet. Therefore, in this study, we investigated the effect of POE on insulin secretion and the intracellular mechanism underlying this effect in INS-1 pancreatic β-cells.
The results showed that POE dose-dependently stimulated insulin secretion in INS-1 pancreatic β-cells in the concentration range from 10 to 200 µg/mL. The insulinotropic effect of POE was dependent on the glucose concentration in the medium. The insulin secretion at 1.1 mM glucose was significantly lower than that at 5.6 and 16.7 mM glucose. Insulin secretion was also significantly stimulated as the concentration of POE was increased even at a basal glucose level. This result suggests that POE promotes insulin secretion on its own as well as in a glucose-dependent manner. Korean white ginseng stimulated glucose-dependent insulin secretion in pancreatic islet as an insulin secretagogue [35]. However, Korean red ginseng significantly stimulated insulin secretion by its own in basal glucose concentration. In other words, it acted as an initiator for insulin secretion, not as a potentiator in a glucose-dependent manner. It was reported that Korean red ginseng had a beneficial effect on the treatment of diabetes by stimulating insulin secretion by its own [36]. In addition, a phenyl alkyl ether derivative isolated from the Kalanchoe pinnata leaves showed insulin secretagogue action in glucose independent manner, which could be useful in treatment of diabetes [37].
To understand the mechanism of the insulinotropic effect of POE, we examined the K+ATP channel-dependent pathway in INS-1 pancreatic β-cells. Most insulin secretagogues promote insulin secretion by closing K+ATP channels in the cell membrane, thereby causing membrane depolarization and consequently activating voltage-gated Ca2+ influx into pancreatic β-cells [3839]. Diazoxide and verapamil are substances that open voltage K+ATP channels, block the voltage-gated Ca2+ influx, and consequently prevent insulin secretion [40]. Our study showed that treatment with diazoxide or verapamil along with POE inhibits Ca2+ influx by blocking depolarization and significantly decreases insulin secretion. These observations suggest that the insulinotropic effect of POE may be associated with depolarization of the cell membrane and with the closure of K+ATP channels in INS-1 pancreatic β-cells. This notion is supported by our finding that treatment with a depolarizing concentration of KCl along with POE significantly stimulated insulin secretion as compared to the treatment with KCl alone.
P. oleracea L. contains various kinds of compounds such as alkaloids, terpenoids, coumarins, and flavonoids [1011]. According to one study, resveratrol among flavonoids acts as an insulin secretagogue through membrane depolarization in a mouse β-cell line [41]. Moreover P. oleracea extract contains not only flavonoids but also triterpenoids [1142]. The compound 3β-hidroxihop-22(29)ene (3-BHO) is a triterpenoid and increases insulin secretion by regulating Ca2+ influx through the K+ATP channel-dependent pathway in islets [43]. The insulin secretory effects of POE may be due to the presence of active compounds such as flavonoids and triterpenoids in this extract.
In addition, POE significantly increased insulin secretion induced by insulin secretagogues, especially by tolbutamide, thereby closing K+ATP channels and inducing insulin secretion The closure of the K+ATP channel and the increase in intracellular Ca2+ concentration, plays a pivotal role in insulin secretion [38394445]. Calcium influx into pancreatic β-cells in particular, has been found to be important for promoting insulin secretion [4647]. Thus, we tested whether the insulinotropic effect of POE is due to the influx of extracellular Ca2+. The insulinotropic effect of POE was significantly attenuated by removal of Ca2+ from the extracellular fluid, but insulin secretion significantly increased in the presence of Ca2+ as POE and glucose concentrations increased. This result indicates that POE induces an influx of intracellular Ca2+ and stimulates insulin secretion in the pancreatic β-cell line. To demonstrate the intracellular Ca2+ influx by depolarization and to characterize functional activity of insulin secretion under the influence of Ca2+, intracellular Ca2+ visualization was performed. In relation to intracellular Ca2+, cells treated with POE showed changes in fluorescence in red, green and blue tones, indicating significant increase in intracellular Ca2+, which positively correlated with increases in the concentrations of insulin. The results indicate that POE induced depolarization and increased intracellular Ca2+ influx, which promoted insulin secretion.
This study shows that POE stimulates insulin secretion in a glucose concentration-dependent manner, and the stimulatory effect is due to intracellular Ca2+ influx via depolarization through the K+ATP channel-dependent pathway in INS-1 pancreatic β-cells. Our results suggest that POE may be useful as a potential natural substance for the treatment of type 2 diabetes by promoting insulin secretion. Nevertheless, further research is needed to identify the compounds in POE that are responsible for this effect.
Notes
References
1. Beardsall K, Yuen K, Williams R, Dunger D. Applied physiology glucose control. Curr Paediatr. 2003; 13:543–548.
2. Ferrer JC, Favre C, Gomis RR, Fernández-Novell JM, Garcia-Rocha M, de la Iglesia N, Cid E, Guinovart JJ. Control of glycogen deposition. FEBS Lett. 2003; 546:127–132. PMID: 12829248.


3. Düfer M, Haspel D, Krippeit-Drews P, Kelm M, Ranta F, Nitschke R, Ullrich S, Aguilar-Bryan L, Bryan J, Drews G. The KATP channel is critical for calcium sequestration into non-ER compartments in mouse pancreatic beta cells. Cell Physiol Biochem. 2007; 20:65–74. PMID: 17595516.


4. Tanaka T, Nagashima K, Inagaki N, Kioka H, Takashima S, Fukuoka H, Noji H, Kakizuka A, Imamura H. Glucose-stimulated single pancreatic islets sustain increased cytosolic ATP levels during initial Ca2+ influx and subsequent Ca2+ oscillations. J Biol Chem. 2014; 289:2205–2216. PMID: 24302735.
6. Henquin JC. Pathways in β-cell stimulus-secretion coupling as targets for therapeutic insulin secretagogues. Diabetes. 2004; 53(Suppl 3):S48–S58. PMID: 15561921.
7. Del Prato S, Pulizzi N. The place of sulfonylureas in the therapy for type 2 diabetes mellitus. Metabolism. 2006; 55:S20–S27.


8. Modak M, Dixit P, Londhe J, Ghaskadbi S, Devasagayam TPA. Indian herbs and herbal drugs for the treatment of diabetes. J Clin Biochem Nutr. 2007; 40:163–173. PMID: 18398493.
9. Hasani-Ranjbar S, Larijani B, Abdollahi M. A systematic review of Iranian medicinal plants useful in diabetes mellitus. Arch Med Sci. 2008; 4:285–292.
10. Liu XF, Zheng CG, Shi HG, Tang GS, Wang WY, Zhou J, Dong LW. Ethanol extract from Portulaca oleracea L. attenuated acetaminophen-induced mice liver injury. Am J Transl Res. 2015; 7:309–318. PMID: 25901199.
11. Zhou YX, Xin HL, Rahman K, Wang SJ, Peng C, Zhang H. Portulaca oleracea L.: a review of phytochemistry and pharmacological effects. BioMed Res Int. 2015; 2015:925631. PMID: 25692148.
12. Lee AS, Lee YJ, Lee SM, Yoon JJ, Kim JS, Kang DG, Lee HS. Portulaca oleracea ameliorates diabetic vascular inflammation and endothelial dysfunction in db/db mice. Evid Based Complement Alternat Med. 2012; 2012:741824. PMID: 22474522.
13. Xu X, Yu L, Chen G. Determination of flavonoids in Portulaca oleracea L. by capillary electrophoresis with electrochemical detection. J Pharm Biomed Anal. 2006; 41:493–499. PMID: 16516429.


14. Chan K, Islam MW, Kamil M, Radhakrishnan R, Zakaria MN, Habibullah M, Attas A. The analgesic and anti-inflammatory effects of Portulaca oleracea L. subsp. sativa (Haw.) Celak. J Ethnopharmacol. 2000; 73:445–451. PMID: 11090998.


15. Abdel Moneim AE. The neuroprotective effects of purslane (Portulaca oleracea) on rotenone-induced biochemical changes and apoptosis in brain of rat. CNS Neurol Disord Drug Targets. 2013; 12:830–841. PMID: 23844694.
16. Gu JF, Zheng ZY, Yuan JR, Zhao BJ, Wang CF, Zhang L, Xu QY, Yin GW, Feng L, Jia XB. Comparison on hypoglycemic and antioxidant activities of the fresh and dried Portulaca oleracea L. in insulin-resistant HepG2 cells and streptozotocin-induced C57BL/6 J diabetic mice. J Ethnopharmacol. 2015; 161:214–223. PMID: 25523372.
17. Niu B, Li C, Su H, Li Q, He Q, Liu L, Xue Y, Shen T, Xia X. Glucagon-like peptide-1 receptor agonist exendin-4 protects against interleukin-1 β-mediated inhibition of glucose-stimulated insulin secretion by mouse insulinoma β cells. Exp Ther Med. 2017; 14:2671–2676. PMID: 28947919.
18. Tanaka Y, Tran PO, Harmon J, Robertson RP. A role for glutathione peroxidase in protecting pancreatic beta cells against oxidative stress in a model of glucose toxicity. Proc Natl Acad Sci U S A. 2002; 99:12363–12368. PMID: 12218186.
19. Kim BM, Min BH, Park IS. Transplantation of Langerhans islet into digestive organs of the diabetic rat. Korean J Anat. 1990; 32:869–881.
20. Nenquin M, Szollosi A, Aguilar-Bryan L, Bryan J, Henquin JC. Both triggering and amplifying pathways contribute to fuel-induced insulin secretion in the absence of sulfonylurea receptor-1 in pancreatic β-cells. J Biol Chem. 2004; 279:32316–32324. PMID: 15175349.


21. Leibiger B, Moede T, Schwarz T, Brown GR, Köhler M, Leibiger IB, Berggren PO. Short-term regulation of insulin gene transcription by glucose. Proc Natl Acad Sci U S A. 1998; 95:9307–9312. PMID: 9689076.


22. Díaz-Flores M, Angeles-Mejia S, Baiza-Gutman LA, Medina-Navarro R, Hernández-Saavedra D, Ortega-Camarillo C, Roman-Ramos R, Cruz M, Alarcon-Aguilar FJ. Effect of an aqueous extract of Cucurbita ficifolia Bouché on the glutathioneredox cycle in mice with STZ-induced diabetes. J Ethnopharmacol. 2012; 144:101–108. PMID: 22960550.
23. Kitabchi AE, Umpierrez GE, Miles JM, Fisher JN. Hyperglycemic crises in adult patients with diabetes. Diabetes Care. 2009; 32:1335–1343. PMID: 19564476.


24. Pirart J. Diabetes mellitus and its degenerative complieations: a prospective study of 4400 patients observed between 1947 and 1973. Diabetes Care. 1978; 1:168–188.
25. Dennis JW, Laferté S, Waghorne C, Breitman ML, Kerbel RS. Beta 1-6 branching of Asn-linked oligosaccharides is directly associated with metastasis. Science. 1987; 236:582–585. PMID: 2953071.
26. Turner RC, Holman RR. Lessons from UK prospective diabetes study. Diabetes Res Clin Pract. 1995; 28(Suppl):S151–S157. PMID: 8529508.


27. Bander A. Mechanism of action of hypoglycemic sulfonyl urea compounds, D 860 & BZ 55. Dtsch Med Wochenschr. 1959; 84:996–1002. PMID: 13652690.
28. Assad T, Khan RA, Feroz Z. Evaluation of hypoglycemic and hypolipidemic activity of methanol extract of Brassica oleracea. Chin J Nat Med. 2014; 12:648–653. PMID: 25263975.


29. El-Sayed MI. Effects of Portulaca oleracea L. seeds in treatment of type-2 diabetes mellitus patients as adjunctive and alternative therapy. J Ethnopharmacol. 2011; 137:643–651. PMID: 21718775.


30. Movahedian A, Ghannadi A, Vashirnia M. Hypocholesterolemic effects of purslane extracts on serum lipids in rabbits fed with high cholesterol levels. Int J Pharmacol. 2007; 3:285–289.
31. Shehata MMSM, Soltan SSA. The effects of purslane and celery on hypercholesterolemic mice. World J Diary Food Sci. 2012; 7:212–221.
32. Karimi G, Khoei A, Omidi A, Kalantari M, Babaei J, Taghiabadi E, Razavi BM. Protective effect of aqueous and ethanolic extracts of Portulaca oleracea against cisplatin induced nephrotoxicity. Iran J Basic Med Sci. 2010; 13:31–35.
33. Hozayen W, Bastawy M, Elshafeey H. Effects of aqueous purslane (Portulaca oleracea) extract and fish oil on gentamicin nephrotoxicity in albino rats. Nat Sci. 2011; 9:47–62.
34. Lan S, Fu-er L. Effects of Portulaca oleracea on insulin resistance in rats with type 2 diabetes mellitus. Chin J Integr Med. 2003; 9:289–292.


35. Kimura M, Waki I, Chujo T, Kikuchi T, Hiyama C, Yamazaki K, Tanaka O. Effects of hypoglycemic components in ginseng radix on blood insulin level in alloxan diabetic mice and on insulin release from perfused rat pancreas. J Pharmacobiodyn. 1981; 4:410–417. PMID: 7026762.


36. Kim K, Kim HY. Korean red ginseng stimulates insulin release from isolated rat pancreatic islets. J Ethnopharmacol. 2008; 120:190–195. PMID: 18773949.
37. Patil SB, Dongare VR, Kulkarni CR, Joglekar MM, Arvindekar AU. Antidiabetic activity of Kalanchoe pinnata in streptozotocin-induced diabetic rats by glucose independent insulin secretagogue action. Pharm Biol. 2013; 51:1411–1418. PMID: 23865837.
38. Trube G, Rorsman P, Ohno-Shosaku T. Opposite effects of tolbutamide and diazoxide on the ATP-dependent K+ channel in mouse pancreatic beta-cells. Pflugers Arch. 1986; 407:493–499. PMID: 2431383.
39. Thams P, Anwar MR, Capito K. Glucose triggers protein kinase A-dependent insulin secretion in mouse pancreatic islets through activation of the K+ATP channel-dependent pathway. Eur J Endocrinol. 2005; 152:671–677. PMID: 15817925.
40. Grill V, Radtke M, Qvigstad E, Kollind M, Björklund A. Beneficial effects of K-ATP channel openers in diabetes: an update on mechanisms and clinical experiences. Diabetes Obes Metab. 2009; 11(Suppl 4):143–148.


41. Chen WP, Chi TC, Chuang LM, Su MJ. Resveratrol enhances insulin secretion by blocking K(ATP) and K(V) channels of beta cells. Eur J Pharmacol. 2007; 568:269–277. PMID: 17573071.


42. Xin HL, Xu YF, Hou YH, Zhang YN, Yue XQ, Lu JC, Ling CQ. Two novel triterpenoids from Portulaca oleracea L. Helv Chim Acta. 2008; 91:2075–2080.
43. Castro AJ, Cazarolli LH, de Carvalho FK, de Luz G, Altenhofen D, dos Santos AR, Pizzolatti MG, Silva FR. Acute effect of 3beta-hidroxihop-22(29)ene on insulin secretion is mediated by GLP-1, potassium and calcium channels for the glucose homeostasis. J Steroid Biochem Mol Biol. 2015; 150:112–122. PMID: 25843210.
44. Hannan JM, Marenah L, Ali L, Rokeya B, Flatt PR, Abdel-Wahab YH. Ocimum sanctum leaf extracts stimulate insulin secretion from perfused pancreas, isolated islets and clonal pancreatic beta-cells. J Endocrinol. 2006; 189:127–136. PMID: 16614387.
45. Guldstrand M, Grill V, Björklund A, Lins PE, Adamson U. Improved beta cell function after short-term treatment with diazoxide in obese subjects with type 2 diabetes. Diabetes Metab. 2002; 28:448–456. PMID: 12522324.
46. Herchuelz A, Malaisse WJ. Regulation of calcium fluxes in pancreatic islets: association between calcium and insulin release. J Physiol. 1978; 283:409–424. PMID: 364009.
47. Hellman B, Sehlin J, Täljedal IB. Calcium and secretion: distinction between two pools of glucose-sensitive calcium in pancreatic islets. Science. 1976; 194:1421–1423. PMID: 795030.


Fig. 1
Cytotoxic effect of POE in INS-1 pancreatic β-cells
INS-1 pancreatic β-cells were treated with various concentrations (0.1, 0.5, 1.0 and 2.0 mg/mL) of POE for 24 h, and cell viability was measured via MTT assay. Each value was expressed as mean ± SD in triplicate experiments. NS: non-significant. POE: Portulaca oleracea L. extract.
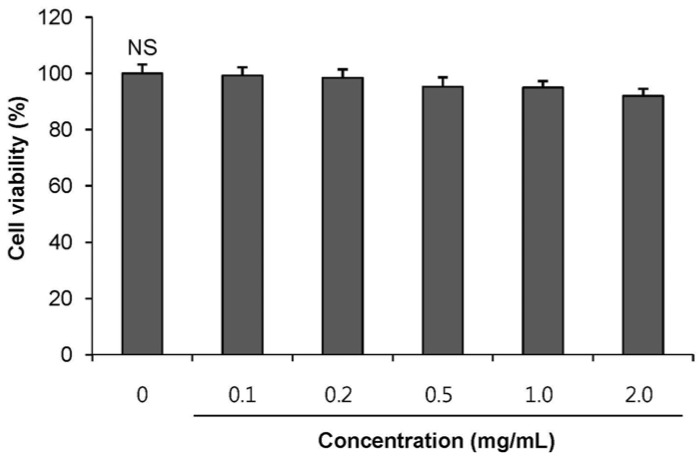
Fig. 2
Effects of the POE on insulin secretion from INS-1 pancreatic β-cells
Cells were incubated with various concentration of POE for 1 h to induce insulin secretion in 1.1 mM (basal glucose level), 5.6 mM (normal glucose level) and 16.7 mM glucose (hyperglycemic condition). Each value was expressed as mean ± SD in triplicate experiments. a~f Values denoted by different letters are significantly different (P < 0.05). POE: Portulaca oleracea L. extract.
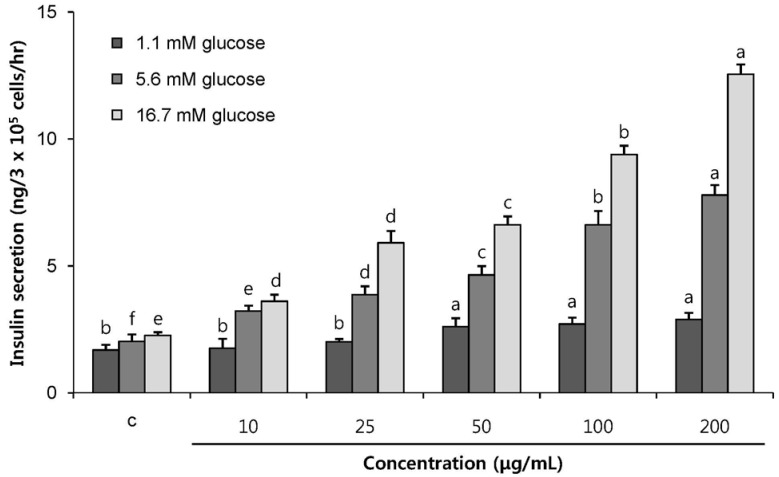
Fig. 3
Effect of POE on insulin secretion from INS-1 pancreatic β-cells in the presence of insulin secretion inhibitor; verapamil and diazoxide
Cells were incubated with or without POE (200 µg/mL) for 1 h in the presence of 1.1, 5.6 or 16.7 mM glucose. The inhibitory effect of verapamil (Vera, 50 mM) and diazoxide (Diaz, 300 µM) on POE-induced increase in insulin secretion was assessed. Each value was expressed as mean ± SD in triplicate experiments. A~B, a~c Values denoted by different letters are significantly different (P < 0.05). POE: Portulaca oleracea L. extract.
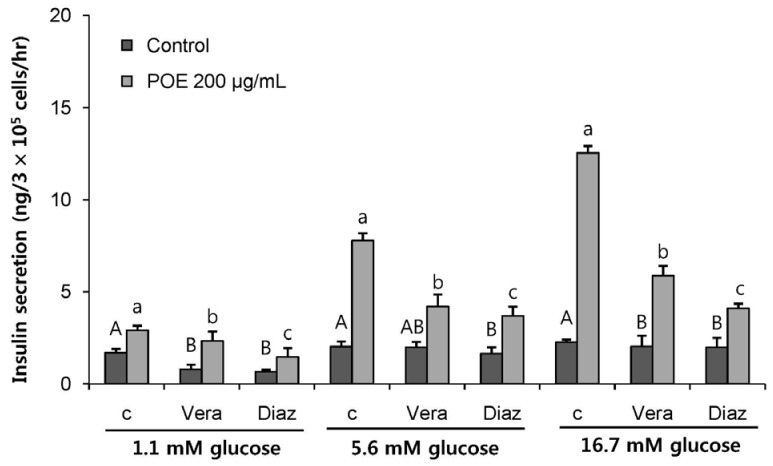
Fig. 4
Effect of POE on insulin secretion from INS-1 pancreatic β-cells in the presence of KCl
Cells were incubated with or without POE (200 µg/mL) for 1 h in the presence of 1.1, 5.6 or 16.7 mM glucose, respectively. The stimulating activity of KCl (30 mM) on POE-induced increase in insulin secretion was examined. Each value was expressed as mean ± SD in triplicate experiments. Significantly different from control at *
P < 0.05. POE: Portulaca oleracea L. extract.
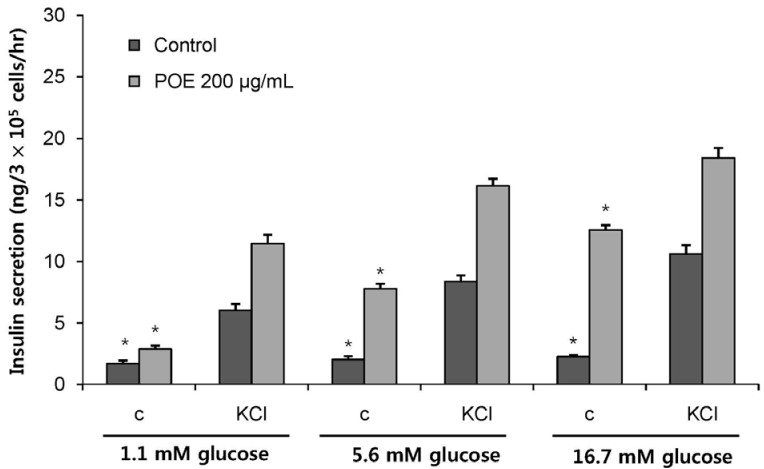
Fig. 5
Effect of insulin secretagogues (L-alanine, IBMX, and tolbutamide) on POE-induced insulin secretion from INS-1 pancreatic β-cells.
Cells were incubated with L-alanine (10 mM), IBMX, (100 µM) and tolbutamide (200 µM) for 1 h in the presence or absence of POE (200 µg/mL). (A) 1.1 mM was basal glucose levels. (B) 5.6 mM was used for normal glucose level. (C) 16.7 mM was for hyperglycemic conditions. Each value was expressed as mean ± SD in triplicate experiments. A~B, a~c Values denoted by different letters are significantly different (P < 0.05). POE: Portulaca oleracea L. extract.
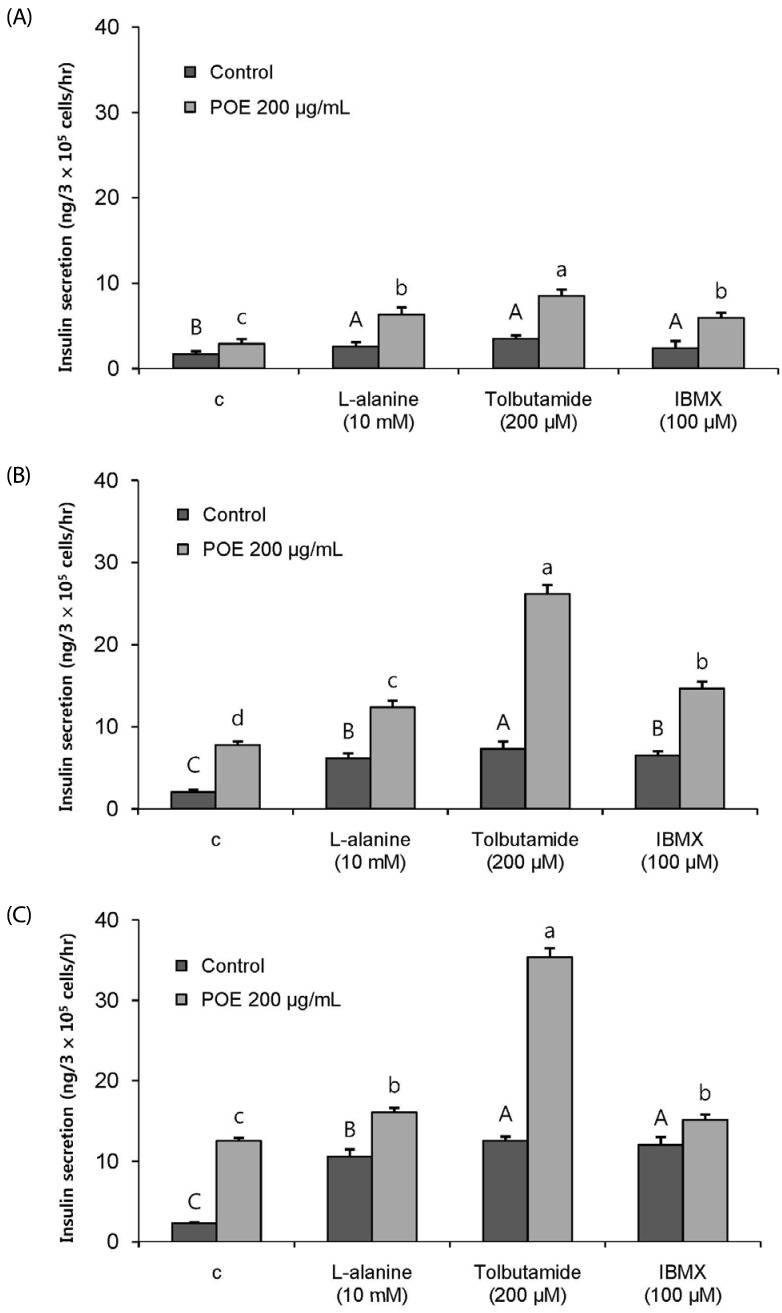
Fig. 6
Effect of the POE on insulin secretion from INS-1 pancreatic β-cells in the presence and the absence of Ca2+
(A) 1.1 mM was basal glucose levels. (B) 5.6 mM was used for normal glucose level. (C) 16.7 mM was for hyperglycemic conditions. Each value was expressed as mean ± SD in triplicate experiments. A~E, a~d Values denoted by different letters are significantly different (P < 0.05). POE: Portulaca oleracea L. extract.
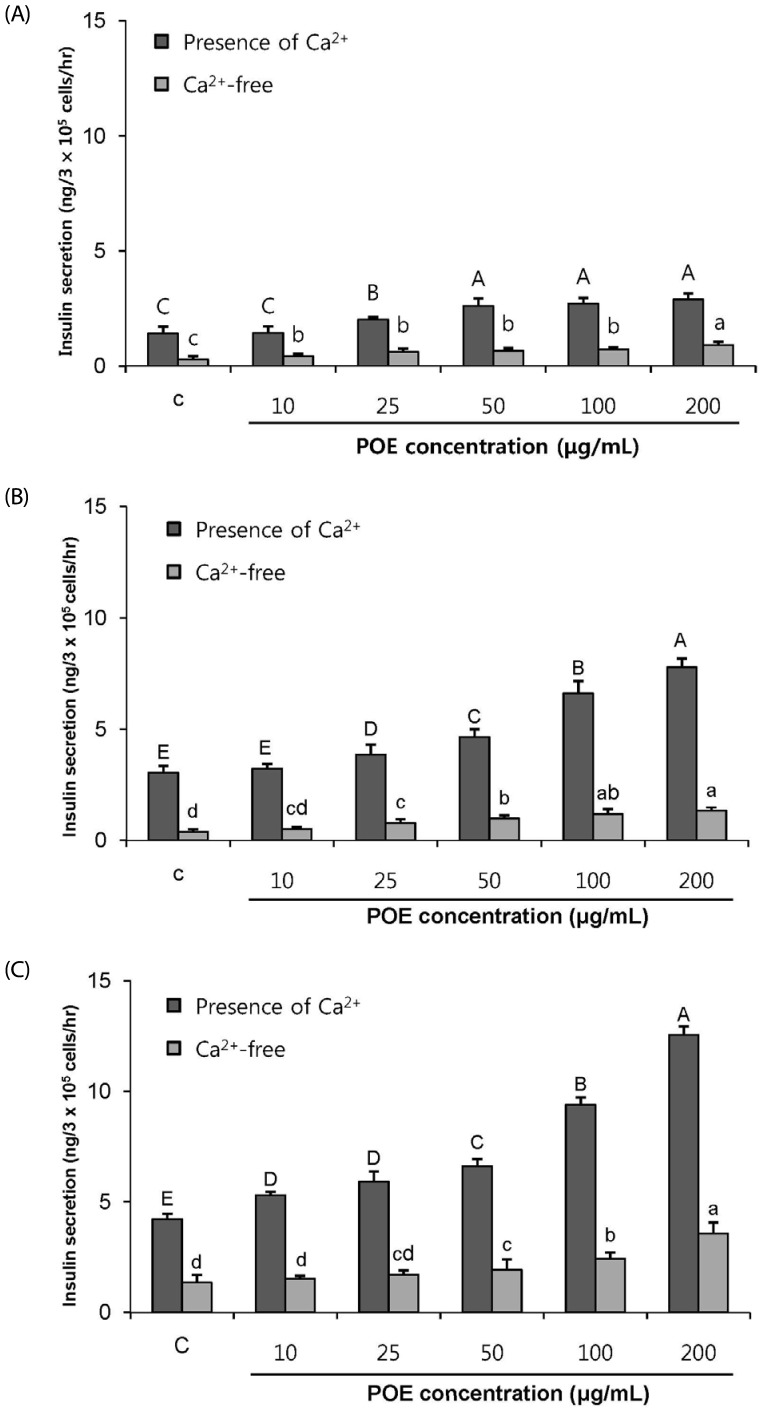
Fig. 7
The effect of POE on intracellular Ca2+ in INS-1 pancreatic β-cells
Cells were treated with POE (200 µg/ml) in various glucose concentration (1.1, 5.6 and 16.7 mM glucose). (A) The fluorecence of intracellular Ca2+ cells due to treatment. The fluorescence of the INS-1 pancreatic β-cells in the bright and dark field that shows changes in red, green and blue tone. Red and Blue correspond to high and low Ca2+ concentrations, respectively. (B) The concentration (%) of intracellular Ca2+. Each value was expressed as mean ± SD in triplicate experiments. a–e Values denoted by different letters are significantly different (P < 0.05). POE: Portulaca oleracea L. extract.
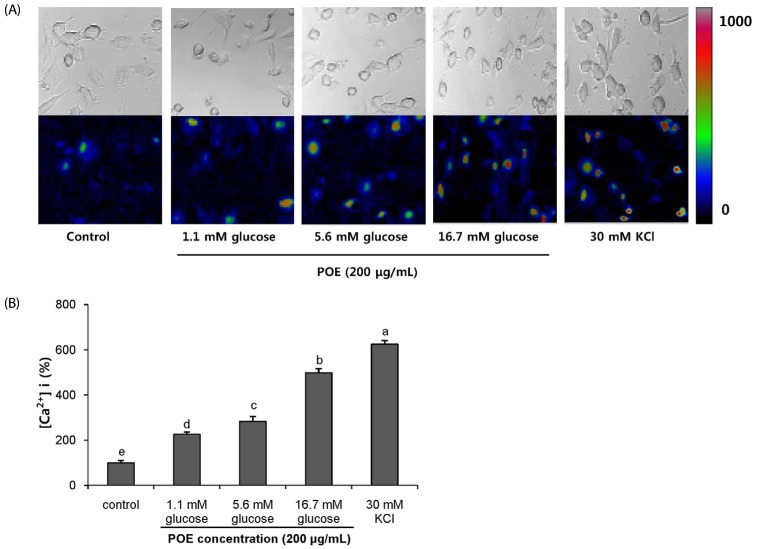