Abstract
Type 1 citrullinemia (CTLN1) is an autosomal recessive inherited metabolic disorder caused by anargininosuccinicnate synthetase deficiency. The patient was a 38-year-old Korean woman who is a carrier for CTLN1 and her first baby was diagnosed with CTLN1. Preimplantation genetic diagnosis (PGD) for CTLN1 in day 3 embryos using polymerase chain reaction was performed for live birth of healthy baby who is no affected with CTLN1. One unaffected blastocyst was transferred. This resulted in a clinical pregnancy and the live birth of healthy male twin. They were confirmed to be unaffected with CTNL1 by post natal diagnosis. This is the first case report of the use of PGD for CTNL1.
Type 1 citrullinemia (CTLN1) is an autosomal recessive urea cycle disorder caused by single-gene mutation to ASS1, which is located on chromosome 9q34.1, and characterized by an argininosuccinic acid synthetase (ASS) deficiency [1,2]. In the urea cycle, ASS catalyses the conversion of citrulline and aspartate to argininosuccinate. An ASS1 deficiency demonstrates fatal clinical manifestations that are characterized by the neonatal or intermittent onset of hyperammonemia which leads to neonatal metabolic coma, mental retardation, and early death when untreated [3]. The incidence of ASS1 gene mutationis estimated to be 1:57,000 births [3,4]. Because of its high mortality, it is important to early diagnose or prevent CTLN1 through prenatal diagnostic techniques such as amniocentesisor chorionic villous sampling (CVS). However, even when then CTLN1 that is confirmed by prenatal diagnosis, there are only two options: termination or living with the disease. Because CTLN1 is a single-gene autosomal recessive mutation, we attempted preimplantation genetic diagnosis (PGD) to avoid pregnancy with affected baby. This report describes the first successful pregnancy and delivery following PGD using polymerase chain reaction (PCR) to diagnose CTLN1 in a carrier couple with a birth history of the child with CTLN1.
A 38-year-old Korean woman had a birth history of the child affected with CTLN1. Peripheral blood gene sequencing by PCR confirmed a homozygotic ASS1 (c.421-2A>G) gene mutation in the first baby. Also both the patient and her husband were confirmed as heterozygous ASS1 (c.421-2A>G) mutation carriersby peripheral blood gene sequencing.
The patient wanted to give birth to a normal baby without the ASS1 gene mutation and we provided counseling to the couple regarding the use of PGD in in vitro fertilization (IVF). The couple subsequently underwent IVF cycle that involved controlled ovarian stimulation using gonadotrophin-realeasing hormone antagonist multiple-dose protocol. Thirteen-oocytes were retrieved 36 hours after human chorionic gonadotropin (hCG) injection. Eight-oocytes were matured and suitable for intra-cytoplasmic sperm injection. Eight oocytes were fertilized of which five were suitable for blastomere biopsy in day 3 embryos. After blastomere biopsy, PCR were performed for ASS1 gene status assessment over 30 cycles. Each cycle consisted of denaturation at 94℃ for 30 seconds, annealing at 60℃ for 30 seconds, and extension at 72℃ for 45 seconds. PCR was carried out in a reaction volume of 10 µL, containing 1 µL WGA product, 10 pmol of each primer and GoTaq PCR Master Mix (Promega, Madison, WI, USA) in a thermocycler, GeneAmp PCR System 9700 (Applied Biosystems, Foster City, CA, USA). Subsequently, DNA sequencing reactions were performed using the same primer pairs, and the Big Dye Terminator V3.1 Cycle Sequencing kit (Applied Biosystems). Electrophoresis and analysis of sequencing reaction mixtures were achieved with an ABI3130xl Genetic Analyzer (Applied Biosystems). One embryo was found to be free of the mutation in ASS, there were found to be four heterozygotic carriers and one embryo failed to yield any result. One unaffected blastocyst was transferred into the uterine cavity (Fig. 1).
After the confirmation of successful pregnancy with monochorionic-diamniotic (MCDA) monozygotic twins using serum β-hCG and ultrasonography, CVS was performed to reconfirm the normal genotypes of the twin babies at 11 weeks of gestation. The results demonstrated normal 46, XY karyotypes without ASS1 mutations. Healthy male MCDA twins were delivered by cesarean sectionat 35 weeks gestation because of preterm premature rupture of fetal membranes. The twins were discharged with the mother without any complications.
CTLN1 requires a diagnosis to be made as soon as possible in addition to long-term surveillance and diligent nutritional and pharmacological management. Treatment consists of protein restriction and supplementation with L-arginine and sodium benzoate and/or phenylbutyrate to increase nitrogen excretion [5]. However, even with treatment, the morbidity and mortality associated with this disorder is high. The long-term consequences of the disease include mild to profound mental retardation, seizures, and growth restriction [3]. The long-term outcomes of CTLN1 remains unknown, however, because virtually no patient surviving into adulthood has been accurately documented [6]. In case of lethal mutation confirmed with prenatal genetic diagnosis using chorionic villous sampling or amniocentesis, pregnancy termination is legal, according to the 1st paragraph of the 14th article in the Mother and Child Health Law of Korea [7]. However, it can be traumatic experience to the patient and her family. For this reason, as is the case for other genetic diseases, there should be a fundamental solution, such as PGD, that ultimately prevents the occurrence of this disease [8].
Since the first reported use of PGD in 1990 [9], the technique has rapidly evolved and been widely applied [10]. According to the European Society for Human Reproduction and Embryology, there have been 27,630 reported IVF cycles with PGD, 5,187 clinical pregnancies and 4,140 deliveries between January 1997 and December 2007 [11].
PGD can be applied to treat any Mendelian disorder whose location is known. More than 200 different disorders have been tested, demonstrating high accuracy and safety [12]. PGD requires access to DNA from gametes or embryos <6 days after fertilization, when implantation occurs. There are 3 potential approaches: 1) polar body biopsy, 2) blastomere biopsy of six to eightcell cleaving embryoson the third day after fertilization, and 3) trophectoderm biopsy of the blastocysts. Diagnostic accuracy is very high (>99%) for both chromosomal abnormalities and single-gene disorders [12]. Genetic analysis for monogenic disease is performed using PCR, while chromosomal analysis is performed using fluorescence in situ hybridization (FISH) [12]. However, the traditional application of FISH using chromosome-specific probes for the detection of aneuploidy and translocations could be replaced by array comparative genome hybridization [13]. It is difficult to assess success rates for PGD because there is currently limited data available. The success rate of IVF with PGD is estimated to be lower than conventional IVF due to the following reasons: 1) enough eggs are not produced or fertilized in the first place, 2) embryos could be damaged during the analysis of genetic disease, and 3) all the embryos can be affected by the genetic disease.
In our present case, we actively prevented CTLN1 by performing PGD. This is the first case of using PGD to prevent CTLN1. This screening strategy allowed the birth of a healthy baby from an at-risk couple who was ethically opposed to other alternatives, such as prenatal testing, pregnancy termination, gamete donation, or adoption. Ultimately, the attitudes and preferences of the informed patients will determine their choices. IVF with PGD can be an appropriate treatment option for patients who have been diagnosed with any single gene disorders.
Figures and Tables
Fig. 1
The sequence of the mutation c.421-2A>G of ASS1 gene and the result of short tandem repeat (STR) microsatellite marker test of 9q34 (D9S195). The mutation site of the electrogram is indicated with red arrows. (A,B) Parents have heterozygote c.421-1A>G. (C) The proband has homozygote c.421-2A>G mutation of the first baby. (D) The preimplantation genetic diagnosis (PGD) blastomere shows normal sequence at c.421-2G site. The peak of capillary electrophoresis for STR marker is shown 306 bp in father and 314, 322 bp in mother respectively. The blastomere shows both 306 bp of father allele and 314 bp of mother allele, which rule out the allele drop phenomenon in the PGD process.
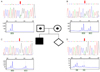
References
1. Engel K, Hohne W, Haberle J. Mutations and polymorphisms in the human argininosuccinate synthetase (ASS1) gene. Hum Mutat. 2009; 30:300–307.
2. Haberle J, Pauli S, Linnebank M, Kleijer WJ, Bakker HD, Wanders RJ, et al. Structure of the human argininosuccinate synthetase gene and an improved system for molecular diagnostics in patients with classical and mild citrullinemia. Hum Genet. 2002; 110:327–333.
3. Maestri NE, Clissold DB, Brusilow SW. Long-term survival of patients with argininosuccinate synthetase deficiency. J Pediatr. 1995; 127:929–935.
4. Brusilow SW, Maestri NE. Urea cycle disorders: diagnosis, pathophysiology, and therapy. Adv Pediatr. 1996; 43:127–170.
5. Singh RH. Nutritional management of patients with urea cycle disorders. J Inherit Metab Dis. 2007; 30:880–887.
6. Brunetti-Pierri N, Lamance KM, Lewis RA, Craigen WJ. 30-year follow-up of a patient with classic citrullinemia. Mol Genet Metab. 2012; 106:248–250.
7. No. 11441, Article 14-1. Mother and Child Health Law. 2012. 05. 23.
8. Batshaw ML, MacArthur RB, Tuchman M. Alternative pathway therapy for urea cycle disorders: twenty years later. J Pediatr. 2001; 138:1 Suppl. S46–S54.
9. Handyside AH, Kontogianni EH, Hardy K, Winston RM. Pregnancies from biopsied human preimplantation embryos sexed by Y-specific DNA amplification. Nature. 1990; 344:768–770.
10. Harper JC, Sengupta SB. Preimplantation genetic diagnosis: state of the art 2011. Hum Genet. 2012; 131:175–186.
11. Harper JC, Wilton L, Traeger-Synodinos J, Goossens V, Moutou C, SenGupta SB, et al. The ESHRE PGD Consortium: 10 years of data collection. Hum Reprod Update. 2012; 18:234–247.
12. Sermon K, Van Steirteghem A, Liebaers I. Preimplantation genetic diagnosis. Lancet. 2004; 363:1633–1641.
13. Simpson JL. Preimplantation genetic diagnosis at 20 years. Prenat Diagn. 2010; 30:682–695.