Abstract
Purpose
Our previous study demonstrated the hypoglycemic effects of mulberry (Morus alba L.) leaf and the underlying mechanisms. Here we explored the potency of mulberry twigs (TW) and root barks (RB) in postprandial hypoglycemic effects in vitro and in vivo.
Methods
The major components of TW and RB were determined by high performance liquid chromatography (HPLC). Alpha-glucosidase inhibition and glucose/fructose uptake inhibition in Caco-2 cells were determined for TW, RB, and their major components, followed by an oral sugar tolerance test (OSTT) in streptozotocin-induced diabetic rats. Male Wistar rats were fed a high-fat diet for 2 weeks and then a single dose of streptozotocin (35 mg/kg B.W) was administered by intraperitoneal injection. Rats with fasting blood glucose levels above 126 mg/dL were randomly divided into 5 groups (n = 8/group) for the following treatments by gavage for 4 weeks: vehicle (normal control and diabetic control), 200 mg/kg B.W of TW or RB or 100 mg/kg B.W of oxyresveratrol (OXY).
Results
OXY and mulberroside A were identified as the major components of TW and OXY, mongolicin, and kuwanon H for RB. A significant inhibitory activity on α-glucosidase was found for TW, RB, and OXY (p = 0.0099). There was a dose-dependent inhibition of TW and RB on the intestinal sugar uptakes in Caco-2 cells, showing a greater impact on fructose compared to glucose. The OSTT showed that TW and RB significantly delayed time to maximal concentration (p = 0.0088) and decreased maximal concentration (p = 0.0043) compared to the control group.
Conclusion
These results suggest that TW and RB may have a postprandial hypoglycemic effect, particularly in the case of high fructose or sucrose intake. OXY was suggested as a contributor to the hypoglycemic effect of TW and RB. Further studies are needed for the systemic effect of TW and RB in circulation.
Figures and Tables
Fig. 1
HPLC chromatograms of mulberry twigs (A) and root barks (B). Analytical conditions are described in the method section.
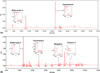
Fig. 2
Inhibitory effects of mulberry twigs, root barks, and their major components on α-glucosidase activity. Values are mean ± SE. Different letters indicate significant differences at the p < 0.05 level using Duncan's multiple range test.
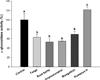
Fig. 3
Inhibitory effects of mulberry twigs, root barks, and oxyresveratrol on α-glucosidase activity with maltose (A) and sucrose (B) as substrates. Values are mean ± SE.
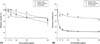
Fig. 4
The effects of mulberry twigs (A) and root barks (B) on cell viability of Caco-2 cells. Cell were incubated with mulberry twigs or root barks at different concentrations ranging from 0 to 1,000 µg/mL for 1 hr. Data was normalized to that of the vehicle. Values are mean ± SE. Different letters indicate significant differences at the p < 0.05 level using Duncan's multiple range test.
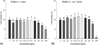
Fig. 5
Inhibitory effects of mulberry twigs and mulberry root barks on sugar uptake in Caco-2-cells: glucose (A) and fructose (B). Caco-2 cells were grown on 24-well plates and incubated in 10 mM Kreb's buffer containing [3H]-2-deoxyglucose or [14C]-fructose for 5 min at 37℃. Inhibition of [3H]-2-deoxyglucose uptake or [14C]-fructose uptake was measured at the increasing concentrations of mulberry twigs (●), mulberry root barks (○), by quantitation of radioactivity using scintillation counter. Values represent mean ± SE.
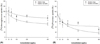
Fig. 6
Effects of mulberry extracts on postprandial blood glucose responses (AUC levels, C max, T max) in streptozotocin-incduced Diabetic Wistar rats (A) Day 0 or (B) Day 28. The inset graph is comparison of fasting blood glucose level between CON and DB CON. The animals received orally administered sucrose (2 g/kg B.W.) with mulberry twigs, root barks extract and OXY after overnight fasting for 12 h. Blood glucose levels were determined from tail blood samples at 0, 15, 30, 60, 90, and 120 min. CON, normal control (n = 13); DB CON, Diabetes control (n = 8); TW, Mulberry twigs extract 200 mg/kg B.W. (n = 7); RB, Mulberry root barks extract 200 mg/kg B.W. (n = 8); OXY, Oxyresveratrol 100mg/kg B.W. (n = 8); Values represent mean ± SE. Different letters indicate significant differences at the p < 0.05 level using repeated measure analysis of variance (ANOVA) with post hoc Duncan's multiple comparison tests. Asterisks in the inset graph denote significant differences relative to control group (p < 0.05 by Student's t-test).
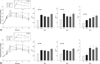
References
1. Task Force Team For Basic Statistical Study of Korean Diabetes Mellitus. Report of task force team for basic statistical study of Korean diabetes mellitus: diabetes in Korea 2007. 1st ed. Seoul: Goldfishery;2007. p. 1–57.
2. Lee SY, Park SL, Nam YD, Yi SH, Lim SI. Anti-diabetic effects of fermented green tea in KK-A y diabetic mice. Korean J Food Sci Technol. 2013; 45(4):488–494.
3. Jung JK, Park YK. Antidiabetic effect of So-Dang-Hwan in streptozotocin-induced diabetic rats. Korean J Herbol. 2009; 24(1):159–167.
4. Park KS, Ko SK, Chung SH. Comparisons of antidiabetic effect between Ginseng Radix alba, Ginseng Radix rubra and Panax Quinquefoli radix in MLD STZ-induced diabetic rats. J Ginseng Res. 2003; 27(2):56–61.
5. Salimifar M, Fatehi-Hassanabad Z, Fatehi M. A review on natural products for controlling type 2 diabetes with an emphasis on their mechanisms of actions. Curr Diabetes Rev. 2013; 9(5):402–411.


6. Zhu YP. Chinese materia medica: chemistry, pharmacology and applications. Boca Raton (FL): CRC Press;1998.
7. Chae JY, Lee JY, Hoang IS, Whangbo D, Choi PW, Lee WC, Kim JW, Kim SY, Choi SW, Rhee SJ. Analysis of functional components of leaves of different mulberry cultivars. J Korean Soc Food Sci Nutr. 2003; 32(1):15–21.
8. Lee WC, Kim AJ. The study on the functional materials and effects of mulberry leaf. Food Sci Ind. 2003; 36(3):2–14.
9. Kim JY, Chung HI, Jung KO, Wee JH, Kwon O. Chemical profiles and hypoglycemic activities of mulberry leaf extracts vary with ethanol concentration. Food Sci Biotechnol. 2013; 22(5):1443–1447.


10. Park JM, Bong HY, Jeong HI, Kim YK, Kim JY, Kwon O. Postprandial hypoglycemic effect of mulberry leaf in Goto-Kakizaki rats and counterpart control Wistar rats. Nutr Res Pract. 2009; 3(4):272–278.


11. Kim JY, Kwon HJ, Jung JY, Kwon HY, Baek JG, Kim YS, Kwon O. Comparison of absorption of 1-deoxynojirimycin from mulberry water extract in rats. J Agric Food Chem. 2010; 58(11):6666–6671.


12. Kwon HJ, Chung JY, Kim JY, Kwon O. Comparison of 1-deoxynojirimycin and aqueous mulberry leaf extract with emphasis on postprandial hypoglycemic effects: in vivo and in vitro studies. J Agric Food Chem. 2011; 59(7):3014–3019.
13. Kim JY, Choi BG, Jung MJ, Wee JH, Chung KH, Kwon O. Mulberry leaf water extract ameliorates insulin sensitivity in high fat or high sucrose diet induced overweight rats. J Korean Soc Appl Biol Chem. 2011; 54(4):612–618.
14. Chung HI, Kim J, Kim JY, Kwon O. Acute intake of mulberry leaf aqueous extract affects postprandial glucose response after maltose loading: Randomized double-blind placebo-controlled pilot study. J Funct Foods. 2013; 5(3):1502–1506.


15. Kim JY, Mok O, Won P, Won K. Mulberry leaf extract improves postprandial glucose response in prediabetic subjects: a randomized, double-blind placebo-controlled trial. J Med Food. 2015; 18(3):306–313.


16. Wei H, Morusalba L. 桑(Sang, White Mulberry). In : Liu Y, Wang Z, Zhang J, editors. Dietary Chinese Herbs: Chemistry, Pharmacology and Clinical Evidence. Wien: Springer;2015. p. 721–730.
17. Zhang Z, Shi L. Anti-inflammatory and analgesic properties of cismulberroside a from Ramulus mori. Fitoterapia. 2010; 81(3):214–218.


18. Ham I, Jeong E, Lee B, Choi H. The study on anti-hypertensive and anti-diabetic effect of mori ramulus. Korean J Herbol. 2008; 23(2):203–212.
19. Lim CY, Lim SH, Cho SI. Anti-oxidative effects of Mori folium, Mori ramulus and Mori cortex radidus. Thesis Collect Res Inst Korean Med. 2014; 22(2):93–101.
20. Yoon SH, Jung SY, Ha H. Hypoglycemic and enzyme effects of the water extract of Mori radicis cortex in streptozotocin-induced diabetic rats. J Korean Soc Hyg Sci. 2001; 7(2):119–123.
21. Choi SW, Lee YJ, Ha SB, Jeon YH, Lee DH. Evaluation of biological activity and analysis of functional constituents from different parts of mulberry (Morus alba L.) tree. J Korean Soc Food Sci Nutr. 2015; 44(6):823–831.


22. Choi SW, Jang YJ, Lee YJ, Leem HH, Kim EO. Analysis of functional constituents in mulberry (Morus alba L.) twigs by different cultivars, producing areas, and heat processings. Prev Nutr Food Sci. 2013; 18(4):256–262.


23. Jang YJ, Leem HH, Jeon YH, Lee DH, Choi SW. Isolation and identification of α-glucosidase inhibitors from Morus root bark. J Korean Soc Food Sci Nutr. 2015; 44(7):1090–1099.


24. Gerlier D, Thomasset N. Use of MTT colorimetric assay to measure cell activation. J Immunol Methods. 1986; 94(1-2):57–63.


25. Asano N, Oseki K, Tomioka E, Kizu H, Matsui K. N-containing sugars from Morus alba and their glycosidase inhibitory activities. Carbohydr Res. 1994; 259(2):243–255.
26. Park SY, Kim JS, Lee BH, Lim SC, Lee SN, Leem KH, Lee KM. Whitening effects of Mori ramulus, Mori cortex radicis and Mori folium herbal-acupuncture solution after fermentation and heating. J Korean Acupunct Moxibustion Soc. 2009; 26(1):91–98.
27. Kwon YI, Apostolidis E, Shetty K. In vitro studies of eggplant (Solanum melongena) phenolics as inhibitors of key enzymes relevant for type 2 diabetes and hypertension. Bioresour Technol. 2008; 99(8):2981–2988.


28. Krentz AJ, Bailey CJ. Oral antidiabetic agents: current role in type 2 diabetes mellitus. Drugs. 2005; 65(3):385–411.
29. Avignon A, Radauceanu A, Monnier L. Nonfasting plasma glucose is a better marker of diabetic control than fasting plasma glucose in type 2 diabetes. Diabetes Care. 1997; 20(12):1822–1826.


30. Lam SH, Chen JM, Kang CJ, Chen CH, Lee SS. α-Glucosidase inhibitors from the seeds of Syagrus romanzoffiana. Phytochemistry. 2008; 69(5):1173–1178.


31. Kim SM, Lee YM, Kim MJ, Nam SY, Kim SH, Jang HH. Effects of Agrimonia pilosa Ledeb. water extract on α-glucosidase inhibition and glucose uptake in C2C12 skeletal muscle cells. Korean J Food Nutr. 2013; 26(4):806–813.


32. Shim YJ, Doo HK, Ahn SY, Kim YS, Seong JK, Park IS, Min BH. Inhibitory effect of aqueous extract from the gall of Rhus chinensis on alpha-glucosidase activity and postprandial blood glucose. J Ethnopharmacol. 2003; 85(2-3):283–287.


33. Hogan S, Zhang L, Li J, Sun S, Canning C, Zhou K. Antioxidant rich grape pomace extract suppresses postprandial hyperglycemia in diabetic mice by specifically inhibiting alpha-glucosidase. Nutr Metab (Lond). 2010; 7(1):71.


34. Hidalgo IJ, Raub TJ, Borchardt RT. Characterization of the human colon carcinoma cell line (Caco-2) as a model system for intestinal epithelial permeability. Gastroenterology. 1989; 96(3):736–749.


35. Murota K, Shimizu S, Miyamoto S, Izumi T, Obata A, Kikuchi M, Terao J. Unique uptake and transport of isoflavone aglycones by human intestinal caco-2 cells: comparison of isoflavonoids and flavonoids. J Nutr. 2002; 132(7):1956–1961.


36. Oitate M, Nakaki R, Koyabu N, Takanaga H, Matsuo H, Ohtani H, Sawada Y. Transcellular transport of genistein, a soybean-derived isoflavone, across human colon carcinoma cell line (Caco-2). Biopharm Drug Dispos. 2001; 22(1):23–29.


37. Vaidyanathan JB, Walle T. Transport and metabolism of the tea flavonoid (−)-epicatechin by the human intestinal cell line Caco-2. Pharm Res. 2001; 18(10):1420–1425.
38. Walle UK, Galijatovic A, Walle T. Transport of the flavonoid chrysin and its conjugated metabolites by the human intestinal cell line Caco-2. Biochem Pharmacol. 1999; 58(3):431–438.


39. Kuo SM. Transepithelial transport and accumulation of flavone in human intestinal Caco-2 cells. Life Sci. 1998; 63(26):2323–2331.

