Abstract
Resveratrol (RSV) exerts several beneficial effects on metabolism and metaflammation-related diseases, including diabetes and non-alcoholic fatty liver disease (NAFLD). The purpose of this study is to investigate whether RSV affects pathophysiology of diabetes and NAFLD as well as hepatic autophagy in a rodent model of diet induced obesity (DIO). DIO was induced in a subset of C57BL/6J mice fed a high fat (HF, 45% kcal fat) diet. After six weeks of HF diet treatment, RSV (8 mg/kg/day) was administered via an osmotic pump for a period of four weeks. Therefore, the experimental groups were as follows: 1) lean control (CON), 2) HF diet-induced obese control (HF), and 3) HF_RSV. Body weight and food intake were monitored daily. Fasting glucose, insulin, and adiponectin in serum and lipid profiles in serum and liver were analyzed. In addition, the autophagic process was investigated using transmission electron microscopy (TEM). Body weight and food intake were not affected by RSV treatment. Impaired glucose control accompanied by DIO was recovered with RSV as shown by lower levels of fasting serum glucose and insulin when compared with HF obese controls. In addition, RSV treatment resulted in increased levels of serum adiponectin, however, indices of lipid profile in serum and livers were reduced. Results of TEM analysis showed that a HF diet induced excessive autophagy with the presence of double-membrane autophagosomes, which was ameliorated by RSV. The regulatory effect of RSV on autophagy was confirmed by the altered LC3-II formation, which increased with a HF diet and was decreased by RSV treatment. These results suggest that RSV treatment improves glucose control and lipid profile and these beneficial effects may be mediated by an altered autophagic process.
Figures and Tables
Fig. 1
The effect of resveratrol (RSV) on double-membrane autophagosome formation and cell organelle morphology. RSV reduces the formation of double-membrane autophagosomes (gray arrows), and increase mitochondrial size and cristae content in livers. Original magnification: 10,000 × (A-C) and 25,000 × (D-F). CON: control, HF: high fat.
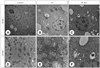
Fig. 2
The effect of resveratrol (RSV) on (A) number of autophagosomes and (B) mitochondrial DNA contents. Number of autophagosomes were analyzed from three separate images per each mouse liver. Mean ± SEM (n = 6 per group). Different letters within a variable are significantly different at p < 0.05. CON: control, HF: high fat.
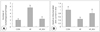
Fig. 3
Representative Western blot image of LC3. LC3-II formation was analyzed in livers of control (CON), high fat diet (HF), and HF with resveratrol (HF_RSV) treated-mice. Beta-actin as a loading control.
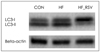
References
1. Rector RS, Thyfault JP, Wei Y, Ibdah JA. Non-alcoholic fatty liver disease and the metabolic syndrome: an update. World J Gastroenterol. 2008; 14(2):185–192.


2. Torres DM, Harrison SA. Diagnosis and therapy of nonalcoholic steatohepatitis. Gastroenterology. 2008; 134(6):1682–1698.


4. Madeo F, Tavernarakis N, Kroemer G. Can autophagy promote longevity? Nat Cell Biol. 2010; 12(9):842–846.


5. Mathew R, Karp CM, Beaudoin B, Vuong N, Chen G, Chen HY, Bray K, Reddy A, Bhanot G, Gelinas C, Dipaola RS, Karantza-Wadsworth V, White E. Autophagy suppresses tumorigenesis through elimination of p62. Cell. 2009; 137(6):1062–1075.


7. Nakahira K, Haspel JA, Rathinam VA, Lee SJ, Dolinay T, Lam HC, Englert JA, Rabinovitch M, Cernadas M, Kim HP, Fitzgerald KA, Ryter SW, Choi AM. Autophagy proteins regulate innate immune responses by inhibiting the release of mitochondrial DNA mediated by the NALP3 inflammasome. Nat Immunol. 2011; 12(3):222–230.


8. Zhang C, He Y, Okutsu M, Ong LC, Jin Y, Zheng L, Chow P, Yu S, Zhang M, Yan Z. Autophagy is involved in adipogenic differentiation by repressesing proteasome-dependent PPARγ2 degradation. Am J Physiol Endocrinol Metab. 2013; 305(4):E530–E539.


9. Singh R, Kaushik S, Wang Y, Xiang Y, Novak I, Komatsu M, Tanaka K, Cuervo AM, Czaja MJ. Autophagy regulates lipid metabolism. Nature. 2009; 458(7242):1131–1135.


10. Singh R, Cuervo AM. Lipophagy: connecting autophagy and lipid metabolism. Int J Cell Biol. 2012; 2012:282041.


11. Papáčková Z, Daňková H, Páleníčková E, Kazdová L, Cahová M. Effect of short- and long-term high-fat feeding on autophagy flux and lysosomal activity in rat liver. Physiol Res. 2012; 61:Suppl 2. S67–S76.


12. Signorelli P, Ghidoni R. Resveratrol as an anticancer nutrient: molecular basis, open questions and promises. J Nutr Biochem. 2005; 16(8):449–466.


14. Bertelli AA, Das DK. Grapes, wines, resveratrol, and heart health. J Cardiovasc Pharmacol. 2009; 54(6):468–476.


15. Brasnyó P, Molnár GA, Mohás M, Markó L, Laczy B, Cseh J, Mikolás E, Szijártó IA, Mérei A, Halmai R, Mészáros LG, Sümegi B, Wittmann I. Resveratrol improves insulin sensitivity, reduces oxidative stress and activates the Akt pathway in type 2 diabetic patients. Br J Nutr. 2011; 106(3):383–389.


16. Park CE, Kim MJ, Lee JH, Min BI, Bae H, Choe W, Kim SS, Ha J. Resveratrol stimulates glucose transport in C2C12 myotubes by activating AMP-activated protein kinase. Exp Mol Med. 2007; 39(2):222–229.


17. Sharma S, Misra CS, Arumugam S, Roy S, Shah V, Davis JA, Shirumalla RK, Ray A. Antidiabetic activity of resveratrol, a known SIRT1 activator in a genetic model for type-2 diabetes. Phytother Res. 2011; 25(1):67–73.


18. Kang W, Hong HJ, Guan J, Kim DG, Yang EJ, Koh G, Park D, Han CH, Lee YJ, Lee DH. Resveratrol improves insulin signaling in a tissue-specific manner under insulin-resistant conditions only: in vitro and in vivo experiments in rodents. Metabolism. 2012; 61(3):424–433.


19. Szkudelski T, Szkudelska K. Anti-diabetic effects of resveratrol. Ann N Y Acad Sci. 2011; 1215:34–39.


20. Hou X, Xu S, Maitland-Toolan KA, Sato K, Jiang B, Ido Y, Lan F, Walsh K, Wierzbicki M, Verbeuren TJ, Cohen RA, Zang M. SIRT1 regulates hepatocyte lipid metabolism through activating AMP-activated protein kinase. J Biol Chem. 2008; 283(29):20015–20026.


21. Picca A, Fracasso F, Pesce V, Cantatore P, Joseph AM, Leeuwenburgh C, Gadaleta MN, Lezza AM. Age- and calorie restriction-related changes in rat brain mitochondrial DNA and TFAM binding. Age (Dordr). 2012; Forthcoming.


22. Yoshino J, Conte C, Fontana L, Mittendorfer B, Imai S, Schechtman KB, Gu C, Kunz I, Rossi Fanelli F, Patterson BW, Klein S. Resveratrol supplementation does not improve metabolic function in nonobese women with normal glucose tolerance. Cell Metab. 2012; 16(5):658–664.


23. Chang CC, Chang CY, Wu YT, Huang JP, Yen TH, Hung LM. Resveratrol retards progression of diabetic nephropathy through modulations of oxidative stress, proinflammatory cytokines, and AMP-activated protein kinase. J Biomed Sci. 2011; 18(1):47.


24. Wang GL, Fu YC, Xu WC, Feng YQ, Fang SR, Zhou XH. Resveratrol inhibits the expression of SREBP1 in cell model of steatosis via Sirt1-FOXO1 signaling pathway. Biochem Biophys Res Commun. 2009; 380(3):644–649.


26. Kallwitz ER, McLachlan A, Cotler SJ. Role of peroxisome proliferators-activated receptors in the pathogenesis and treatment of nonalcoholic fatty liver disease. World J Gastroenterol. 2008; 14(1):22–28.


27. Masaki T, Chiba S, Tatsukawa H, Yasuda T, Noguchi H, Seike M, Yoshimatsu H. Adiponectin protects LPS-induced liver injury through modulation of TNF-alpha in KK-Ay obese mice. Hepatology. 2004; 40(1):177–184.
29. Morselli E, Mariño G, Bennetzen MV, Eisenberg T, Megalou E, Schroeder S, Cabrera S, Bénit P, Rustin P, Criollo A, Kepp O, Galluzzi L, Shen S, Malik SA, Maiuri MC, Horio Y, López-Otín C, Andersen JS, Tavernarakis N, Madeo F, Kroemer G. Spermidine and resveratrol induce autophagy by distinct pathways converging on the acetylproteome. J Cell Biol. 2011; 192(4):615–629.

