Abstract
Histone deacetylases (HDACs) are epigenetic regulators that regulate the histone tail, chromatin conformation, protein-DNA interaction, and even transcription. HDACs are also post-transcriptional modifiers that regulate the protein acetylation implicated in several pathophysiologic states. HDAC inhibitors have been highlighted as a novel category of anti-cancer drugs. To date, four HDAC inhibitors, Vorinostat, Romidepsin, Panobinostat, and Belinostat, have been approved by the United States Food and Drug Administration. Principally, these HDAC inhibitors are used for hematologic cancers in clinic with less severe side effects. Clinical trials are continuously expanding to address other types of cancer and also nonmalignant diseases. HDAC inhibition also results in beneficial outcomes in various types of neurodegenerative diseases, inflammation disorders, and cardiovascular diseases. In this review, we will briefly discuss 1) the roles of HDACs in the acquisition of a cancer's phenotype and the general outcome of the HDAC inhibitors in cancer, 2) the functional relevance of HDACs in cardiovascular diseases and the possible therapeutic implications of HDAC inhibitors in cardiovascular disease.
Histone deacetylases (HDACs) are the group of enzymes that remove the acetyl group from lysine residue. To date, 18 mammalian HDACs have been identified and are characterized into four classes: class I HDACs (HDACs 1, 2, 3, and 8), class II HDACs (HDACs 4, 5, 6, 7, 9, and 10), class IV (HDAC 11) and class III (sirtuin family: sirt1-sirt7). Class II HDACs are further divided into two subgroups: class IIa, which has a large C-terminus, and class IIb, which has two deacetylase domains. Class I, II, and IV HDACs need a zinc ion (Zn2+) and share a similar catalytic core for acetyl-lysine hydrolysis,1 while class III HDACs require a nicotinamide adenine dinucleotide for their enzyme activity.
Even though both class I and class II HDACs have a conserved HDAC domain, they have quite different characteristics. Class I HDACs are present ubiquitously, whereas class II HDACs are expressed in a tissue-specific manner relatively. The class I HDACs are located mainly in the nucleus, whereas class II HDACs undergo shuttling from the nucleus to the cytoplasm after phosphorylation by protein kinase C or by protein kinase D. The class IV HDAC, HDAC11 shares the catalytic domain both of class I and II HDACs. The specific role of class IV HDAC, however, still remains unclear.
Because of the Zn2+-dependent nature of the HDAC domain in class I, II, and IV HDACs, the intrinsic activity of HDACs is suppressed by inhibitors which occupy the catalytic core of the zinc-binding site.2 The specific motif of HDAC inhibitor, which fits into the tubular pocket where the Zn2+ existed originally, then interferes with the binding of the Zn2+. HDAC inhibitors can be categorized by the structure of their Zn2+-binding group: hydroxamic acids, carboxylic acid, benzamides, and cyclic peptides.3 For examples, potent non-selective HDAC inhibitors, trichostatin A (TSA) and suberanilohydroxamic acid (SAHA) are in the hydroxamic acid group, while valproate is a member of the carboxylic acid group, MS-275 is widely used benzamide group inhibitor, and Romidepsin is the representative inhibitor having a cyclic peptide structure.
HDACs have an important role both in transcription regulation and in protein modification. As HDACs remove the acetyl moiety from lysine residues at histone tails, which tighten the interaction between the positive-charged histones and the negative-charged DNA. Therefore, histone deacetylation leads to chromatin compaction and inhibits the binding of transcription machinery at the promoter region, which results in repression of mRNA synthesis. Besides the role in transcription-repression, HDACs also function as regulators in posttranslational modification (PTM). HDACs deacetylate non-histone proteins including both transcription factors, such as E2F, p53, c-Myc, and NF-κB, and signal mediators, such as Stat3, Smad7, and β-catenin which regulate cellular homeostasis.4 Since PTM determines protein activity, stability, subcellular localization, and protein-protein interactions, these diverse protein modifications by PTM modifiers are important for a proteins fate. PTM determines disease prognosis, and drugs that modulate PTM has been investigated as potentially therapeutic for several pathological disease. Imatinib mesylate successfully interrupts Abl kinase activity by blocking the tyrosine kinase domain and thereby induces apoptosis of malignant cells in chronic myeloid leukemia patients. HDACs are one of the important enzymes that regulate the PTM of other proteins, HDAC inhibitors also have therapeutic potential for refractory diseases. On the basis of the studies demonstrated that HDAC inhibition could reverse cardiovascular diseases, in the present review, we will discuss the role of HDAC and HDAC inhibitors as an important therapeutic target in cardiovascular disease beyond the anticancer properties of HDAC inhibitors.
Incidentally somatic mutations allowed cells to acquire novel capacities, which benefitted their survival. After the accumulation of aberrant phenotypes in single cell in sequential events, a malignant cancer cell can be developed. According to recent studies, many HDACs are increased in malignant cells and HDACs have been closely linked with acquisition of malignant phenotypes in cancerogenesis. Class I HDACs are considered to stimulate cell proliferation and survival based on preclinical and clinical studies. For example, increased expression of HDAC1 was reported in gastrointestinal cancer,56 prostate,7 and breast8 carcinomas. Aberrant upregulation of HDAC2 has been reported in uterine, cervical,9 and gastric10 cancers. HDAC2 is regarded as the responsible factor for the loss of APC expression in colorectal carcinoma.11 Other groups have found that HDAC3 or HDAC6 was also highly overexpressed in colon and breast cancer cells, respectively.612 HDACs expression is increased in solid tumors and in hematological cancers, which correlates with a poor prognosis. The functional relevance of aberrant overexpression of HDACs is somewhat diverse among the specific subtypes. Class I HDACs generally induce cell proliferation. Furthermore both HDAC1 and HDAC2 inhibit apoptosis of cancer cells. Other HDACs, HDAC3, 4, 5, and 8, tend to inhibit differentiation and HDAC4, 6, 9, and 10 are closely linked with cancer angiogenesis while class IIb HDACs, both HDAC6 and 10, provoke cell motility, which results in metastasis (Fig. 1). Inhibitors of HDAC targeting proliferation, differentiation, angiogenesis and migration are a potential cancer therapeutic strategy.
Uncontrolled proliferation is the most distinct phenotype in cancer. The p21 gene, a potent cell-cycle arrester, seems to be correlated with HDACs. The transcription level of p21 is significantly decreased by the upregulation of HDACs in many cancer cell types. Transient overexpression of HDAC2, HDAC3, or HDAC6 induces p21-promoter silencing and subsequent down-regulation of proteins in various cell types.61314 Similarly, an increase in p21-expression and thereby reduced adenoma formation is observed either by knockdown of HDAC2 or HDAC3 by the specific siRNA or by HDAC inhibitors such as sulforaphane,13 valproate (VPA),11 and sodium butyrate.15 Another important gene for proliferation, cyclin D1, which is overexpressed in cancers, is also decreased by treatment with TSA, a non-selective HDAC inhibitor.16
The cancer cells frequently remain undifferentiated status, which accords malignancy to the tumor cells. For the responsible mechanism, down-regulation of differentiation factor, GATA family, is pointed to in several cancers. Treatment with TSA in ovarian cancer cells robustly increases histone acetylation on promoters of GATA4 and GATA6.17 The restoration of both GATA4 and GATA6 results in differentiation of ovarian cancer cells. It may improve the survival rate of cancer patients because differentiated cancer cells are more susceptible to chemotherapy. HDACs have also been found to be involved in leukemogenesis. Runx1 is the definitive factor in hematopoietic linage differentiation. In the case of t(8;21), Runx1 fuses to ETO then generates the Runx1-ETO chimeric protein. Runx1-ETO (also known as AML1-ETO) chimera represses transcription both of p14ARF and c-fms by performing as a dominant negative actor against to wild-type of Runx1 which recruits HDACs for transcription-repressor complex.1819 BCL6, a BTB/POZ member which is critical for survival and differentiation, is the suppressed transcription factor both by class I and class II HDACs in B-cell lymphoma.20
Neoangiogenesis is required for both nutrient and oxygen supply in solid tumors and HDACs perform crucial roles in these processes. HDAC1 overexpression induces angiogenesis by secreting both vascular endothelial growth factors and hypoxia inducible factor-alpha. Immunohistochemical analysis reveals that HDAC1 is highly expressed in hypoxic regions of tumors whereas TSA treatment successfully inhibits hypoxia-induced neoangiogenesis, in vivo.21 Besides TSA, apicidin, and VPA are useful to prevent neoangiogenesis.2223
Metastasis, the spread cancer cells across tissues, occurs in many malignant tumors. Unfortunately, aberrant upregulation of HDACs allows an increase in distant metastasis of cancer cells. Forced expression of HDAC1, 6, or 8 increases cell motility in MCF-7 cells.24 E-cadherin, an important gene for cell-cell adhesion, is regulated by class I HDACs. HDAC1 and HDAC2 repress the transcription of E-cadherin correlated with Snail and mSin3A. TSA treatment abolishes Snail-mediated repression.25 HDAC3 also binds to the E-cadherin promoter after making a complex with PPARγ, and VPA depresses promoter binding of HDAC3-PPARγ which restores E-cadherin expression.26 Similarly, treatment of HDAC inhibitors using low doses suppresses local invasion of fibroblasts,27 B16-BL6 melanoma cell invasion of matrigels,28 and breast cancer cells.
Aneuploidy, or an abnormal number of chromosomes, is identified as a negative prognostic factor for epithelial malignancies. It is reported that aneuploidy is observed in approximately 90% of both solid tumors and hematopoietic neoplasias.29 HDAC2 was highly expressed in aneuploidy cell lines than diploid cell lines. HDAC2 nuclear immunopositive cells increased in aneuploidy colorectal cancer specimens than in diploid carcinomas.30 According to a previous study, HDAC inhibitors lead to a decrease in aneuploidy of malignant cells and an increase in euploidy. It is unclear, however, whether HDAC inhibition specifically induces apoptosis of cancer cells or induces recovery of euploidy.31
To date, the United States Food and Drug Administration (US FDA) has approved four HDAC inhibitors for the anti-cancer drugs: Vorinostat, Romidepsin, Belinostat, and Panobinostat (Table 1). Furthermore, more than five HDAC inhibitors are in clinical trial phase III including repositioning of already approved HDAC inhibitors.
Vorinostat (SAHA, trade name; Zolinza®) is a linear hydroxamate compound that was approved for the treatment of cutaneous T-cell lymphoma (CTCL) in October 2006. The objective response rate, which was determined by clinical responses, was 30%.32 Thrombocytopenia and anemia, however, were reported as severe hematologic side effects which was more frequently reported in the patients who received intravenous administration of Vorinostat.33 Several combination therapies of Vorinostat with a conventional regimen for various solid tumors are now in clinical trials up to phase III.
Romidepsin (FK228 or depsipeptide, trade name; Istodax®) is a cyclicpeptide HDAC inhibitor and was approved in November 2009. Originally the US FDA approved it for CTCL and decided to expand the indication to include peripheral T-cell lymphoma (PTCL) in November, 2011. The overall response rate was 34 % in CTCL34 and the objective response rate of PTCL was 25%.35 Additional clinical studies in solid tumors using combination therapy for pancreatic, breast, non-small cell lung cancer, and thyroid cancers are in progress.
Third FDA approval was given for the HDAC inhibitor in July 2014. Belinostat (PXD101, trade name; Beleodaq®) which is a hydroxamic acid compound licensed for the treatment of relapsed or refractory PTCL. The overall response rate was 26%.36 Like the other two FDA approved HDAC inhibitors, Belinostat is also in the clinical trial phase for solid tumors; anticancer effects against solid tumors, however, was disappointing. The effect concentration of Belinostat was not high enough because of an insufficient blood supply caused by its anti-angiogenic effects.37
The most recently approved HDAC inhibitor is Panobinostat (LBH-589, trade name; Farydak®). Panobinostat was licensed in February 2015 for the treatment of multiple myeloma. Objective responses of Panobinostat is 27%.38 Panobinostat is also a hydroxamic acid group. More cumulative data is necessary.
One carboxylate group HDAC inhibitor (VPA, as magnesium salt) and one benzamide HDAC inhibitor (Entinostat) are on phase III clinical trials. Wildly used as an anti-epileptic drug, VPA is in phase III trials for solid tumors such as cervical cancer or ovarian cancer, and even for spinal muscular atrophy. Entinostat (MS-275) is in phase III for hormone receptor-positive advanced breast cancer and is in phase II for lung cancer, breast cancer, and Hodgkin lymphoma.
Apparently, HDAC inhibitors induce cell cycle arrest and thereby apoptosis in cultured cancer cells. HDAC inhibitors not only increase the transcription level of p21, a potent cell cycle arrestor, but also activate the acetylation of p53. For general effects, HDAC inhibitors stimulate transcription of proapoptotic genes such as Bax, Bak and Apaf1.39
Anticancer properties in humans, however, seem to be somewhat limited. HDAC inhibitors non-specifically block angiogenesis, inflammation, and proliferation. Inhibition of tumor angiogenesis results in a failure of drug delivery to the solid tumors. Anti-inflammation activity also induces apoptosis of tumor-fighting immune cells. These unwanted side effects are regarded as possible mechanisms of the marginal effect against solid tumors. General side effects of HDAC inhibitors in chemotherapy, however, seem to be beneficial in chronic cardiovascular diseases such as atherosclerosis, myocardial infarction, arrhythmia, and transition of heart failure. A new era for HDAC inhibitors is now ready to extend to cardiovascular diseases.
Atherosclerosis is a chronic and a progressive disease of the arteries caused by abnormal accumulation of lipid droplets, inflammation of multifactorial cells, generation of a fibrous cap, and reactive proliferation of vascular smooth muscle cells. According to several studies, HDACs are closely linked with in the progression of atherosclerosis and HDAC inhibitors which successfully prevent the progression of atherosclerosis. Our group40 already suggested that the pan-HDAC inhibitor, scriptaid or TSA, was useful for the prevention of neointima formation from balloon injury. The transcription of p21WAF1/Cip1 was significantly increased in the HDAC inhibitor-treated group.4142 In contrast, a few groups found that HDAC inhibitors might stimulate atherogeneisis. It is noteworthy that HDAC inhibitors effectively suppress vascular smooth muscle cell proliferation, which seems to be effective for preventing atherosclerosis.
It is noteworthy that the characteristics of the certain HDAC inhibitor might result in these controversial outcomes. Global HDAC inhibitors, such as TSA, have a tendency to have a dual response: anti-inflammatory properties at low concentrations but pro-inflammatory effects at high concentrations.43 It is assumed that the role of diverse HDACs associated with single pathophysiology are quite different and the overall inhibition effect of HDAC inhibitors also somewhat varies according to its concentration. Targeted regulation of specific HDACs by their own inhibitor would be an ideal regimen.
Only a few reports have elucidated the therapeutic application of HDAC inhibitors in cardiac arrhythmia. TSA dramatically corrected atrioventricular conduction abnormalities in mouse hearts which were induced by a genetic disruption of HopX.44 The dramatic effect of TSA might be associated with the HopX-HDAC2 axis when considering that HopX directly recruits HDAC2.45
More direct evidence that HDACs are responsible for cardiac arrhythmia have also been suggested; myocytespecific ablation of both HDAC1 and HDAC2 results in an aberrant increase in the subunits of the calcium channel.46 Our group also detected that ion channels such as Scn3b (sodium) and Kcne1 (potassium) were dysregulated when HDAC2 was overexpressed. Dysfunction of Kcne1 may participate in Long QT syndrome and the loss of function of Scn3b is responsible for Burgada syndrome,47 which implicates that alteration of HDAC2 activity may lead to fatal cardiac arrhythmias.
Ventricular myocytes are supported oxygen and nutrients through the specialized circulation system: the coronary arteries. Myocytes, however, are vulnerable to damage even by short term ischemic events because of poor anastomosis in the coronary artery. Furthermore, the myocytes received alternate damage after revascularization. This serial injury is termed ischemia-reperfusion (I/R) injury.
HDAC inhibitors have been highlighted as promising drugs to reduce I/R injury, to ameliorate cardiac dysfunction, and to minimize infarction size.484950 An ex vivo study by use of the Langendorff system reveals that preconditioning of TSA preserves cardiac performance after I/R injury. Preconditioning by injection of TSA before the I/R injury reduces the infarction area and restores contractile dysfunction.48 Furthermore, HDAC inhibitors improve fatty acid oxidation by restoring PGC-1α in I/R injuries.51 To date, it is regarded that the major advantageous effects of HDAC inhibitors in I/R injury is mediated by inhibition of generation of immature vasculatures, by reducing inflammation, or by facilitation of energy metabolism.
HDAC inhibitors are also beneficial for minimizing the scar size of myocardial infarction (MI). The infarction area generated by permanent ligation of the left anterior descending artery is dramatically reduced by administration of HDAC inhibitors such as tributyrin, VPA, or TSA.495052 It has also been reported that administration of TSA for 2 months markedly prevented cardiac dysfunction and suppressed cardiac remodeling.52 Despite some contradictory reports about HDAC inhibitors in acute coronary syndromes, it is more commonly shown that HDAC inhibitors are effective both for preventing cardiac dysfunction and cardiac remodeling after MI.
Cardiac hypertrophy is a kind of adaptation to the increased hemodynamic demand from peripheral tissue or from another underlying diseases such as hypertension, valvular dysfunction, and MI.53 The initial adaption might be physiologic, however, cardiac hypertrophy is the beginning of the global remodeling of the heart. The roles of the HDACs in cardiac hypertrophy are being widely studied by a number of research groups including ours.45465455565758596061626364 Both classes of HDACs, class I and class IIa, are associated with the development of cardiac hypertrophy, however, they perform definitely opposite roles. Genetic ablation of HDAC2 results in resistance to various hypertrophic stimuli.64 Heart-specific overexpression of HDAC2 itself induces cardiac hypertrophy.5564 Although HDAC2 clearly provokes cardiac hypertrophy, the protein levels of HDAC2 are not altered during the process. The intrinsic activity of HDAC2 is increased in response to hypertrophic stimuli by the activated-CK2α1.5556 As for class I HDACs, there has been no clear evidence of class I HDACs other than HDAC2 in cardiac hypertrophy found. Only HDAC3, however, might allow for a transient proliferative potential to cardiomyocyte in the perinatal period.65
By contrast, class IIa HDACs negatively regulates cardiac hypertrophy. Global deletion of HDAC962 or HDAC559 shows an exaggeration of hypertrophic phenotypes. In basal conditions, class IIa HDACs capture MEF2 and interfere with the binding to its motif which results in the suppression of the transcription activity of MEF2. Class IIa HDACs are recognized by a shuttling molecule named 14-3-3 after phosphorylation by PKC/PKD or CaMKII and undergo shuttling out from the nucleus to the cytoplasm. The redistribution of class IIa HDACs causes reactivation of arrested-fetal gene programs which are regulated by MEF2, resulting in cardiac hypertrophy.596166
Because those two classes of HDACs perform opposite functions, the overall efficacy of global HDAC inhibitors in cardiac hypertrophy is questioned. We58 and other research groups6063 have suggested that cardiac hypertrophy can be completely abolished either by non-specific HDAC inhibitors545860 or even by selective class I HDAC inhibitors.586367 To summarize this phenomenon, the anti-hypertrophic properties of the non-selective HDAC inhibitor are mediated by specific regulation of class I HDACs. In addition, recently our group suggested crosstalk between HDAC2 and class IIa HDACs in the development of cardiac hypertrophy. Acetylation of HDAC2 preceded phosphorylation and those modifications were mandatory for activation of HDAC2. HDAC5, a class IIa HDAC, functioned as an enzyme that regulated acetylation of HDAC2. HDAC2 was one of the important pro-hypertrophic mediators regulated by class IIa HDACs.68
A quite recent report clearly demonstrated the role of HDAC3 in high blood pressure and the therapeutic applications of HDAC inhibitors for hypertension control.69 One more report suggest that HDAC4 induces hypertension through vascular inflammation and TSA treatment dramatically ameliorates high blood pressure.70 Taken together, this data suggests that HDAC is a novel therapeutic target for regulation of hypertension.
Cardiac fibrosis associated with hypertrophy is notable in cardiac disease. Cardiac fibrosis results in a loss of elasticity and in insufficient dilation of the contractile chamber in the diastole phase, which is regarded as the major pathophysiology of heart failure with preserved ejection fractions (HFpEF).71 HDAC inhibitors also dramatically blocks cardiac fibrosis.555860 Fibrosis is directly inhibited by HDAC inhibitors rather than secondary changes after improving cardiac hypertrophy. More evidence enforces the postulation that HDAC inhibitors directly regulate transdifferentiation of fibroblasts to myofibroblasts.72 During scar formation, the HDAC2 protein amount is dramatically increased73 and renal fibrosis is also successfully controlled by HDAC inhibitors.7475
Very recently, the European society of cardiology and the American heart association alert the severity of HFpEF, respectively. They summarized the clinical outcomes of HFpEF patients during the last two decades who got the conventional regimen for heart failure with reduced ejection fraction (HFrEF). Strikingly, the standard strategy for HFrEF such as beta-blockers, angiotensin converting enzyme inhibitors/angiotensin receptor blockers, or aldosterone-antagonists did not sufficiently reduce the disease progression of HFpEF. According to rodent HFpEF, however, HDAC inhibition is the most promising and reproducible strategy to reverse and prevent HFpEF to normal heart. Therefore, HDAC inhibitors should be considered to start clinical trials for HFpEF.71
Besides general anti-neoplasmic potential, HDAC inhibitors also block angiogenesis. For example, it is well known that Vorinostat and TSA interfere with the sprouting of capillaries from aorta76 Even though the diverse physiologic activity of HDACs, it is generally accepted that class II HDACs such as HDAC4,77 HDAC5,78 HDAC6,7980 and HDAC78182 are pro-angiogenic.
In contrast with the general concept that HDAC inhibitors inhibit angiogenesis, several studies reported positive outcomes correlated with neoangiogenesis. Long-term treatments of VPA in cerebral infarction resulted in enhancement of neovascularization, reduction of infarction size, and alleviation of cerebral functions.83 Including these reports, many studies suggested that long-term treatment with HDAC inhibitors induces neovascularization.5284
In summary, the global effects of HDAC inhibitors in angiogenesis seem to be controversial. According to recent data, HDAC inhibitor preferentially regulates immature vascularization such as tumor vessels or acute ischemic sprouting.85 Long-term administration of HDAC inhibitors promote intact angiogenesis rather than weak and leaking vessels. More specific studies would be necessary to solve these problems.
It should be considered that in spite of the general benefits of HDAC inhibitor in the cardiovascular diseases, HDAC inhibition may cause harmful results at least in some conditions. For example, HDAC inhibition seems to aggravate the progression of vascular calcification. TSA86 and apicidin (Kwon et al., unpublished data) accelerated the calcification in vitro. E3 ligase MDM2 was dramatically increased in calcification-provocation stimuli, which induced polyubiquitination and subsequent degradation of HDAC1. The loss of activity of HDAC1 plays a crucial role in the progression of vascular calcification. Hence, HDAC inhibitors that reduce HDAC activity in vivo may accelerate vascular calcification. Considering that one of major side effect of Vorinostat is vascular calcification, HDAC inhibitor should be carefully administrated to patients who suffer from atherosclerosis or have a proatherogenic condition such as chronic renal failure or diabetes mellitus.
Besides cancer treatment, HDACs has been studied in various non-cancer diseases including neurodegenerative disease, inflammatory disease, and osteoporosis beyond cardiovascular diseases. For example, a Danish group has tested HDAC inhibitors as an HIV treatment.87 In neurological diseases, HDAC inhibitors improve the performance of learning and memory,88 Alzheimer disease,89 ischemic stroke,90 Huntington's disease.91
Several studies have implied that HDAC inhibitors may improve learning ability and memory formation. The brain slices which were acquired from Vorinostat-treated rat hippocampus showed increased synaptic functions ex vivo.9293 Moreover, RGFP966, a class I HDAC inhibitor, treated rats tended to be faster than the vehicle group in learning and in acute memory formation.94 More specifically, the synapse number of HDAC2 overexpression mice was significantly decreased. The learning impairment of HDAC2 overexpression mice was alleviated by chronic treatment with Vorinostat.95
HDAC inhibitors also might be noteworthy for testing the therapeutic potential of bone diseases such as osteoporosis and fractures. Both the protein stability and transcription level of the master regulator of bone formation, RUNX2, are specifically regulated by HDAC inhibitors.96 Furthermore, HDAC inhibitors suppress osteoarthritis using their anti-inflammation effects on joint inflammation and thereby cartilage degeneration.97
In this review, we summarize 1) the roles of HDAC in tumorigenesis and the current uses of HDAC inhibitors in cancer, 2) the functional relevance of HDACs and the therapeutic potentials of HDAC inhibitors in cardiovascular diseases (Fig. 2). According to clinical and nonclinical studies, HDAC inhibitors might result in cell-cycle arrest, apoptosis, differentiation, anti-inflammation, anti-invasion, and anti-proliferation. In short, HDAC inhibitors have general anti-tumor activities. Four HDAC inhibitor approved by the US FDA are beneficial to hematological malignancy but are having disappointing results in solid tumors as a single drug therapy. The limited effects on solid tumors seem to be a failure of the drug delivery into the cancer tissue because of insufficient blood supply caused by its anti-angiogenetic effect.37
HDAC inhibitors are also beneficial for preventing the disease progression of several cardiovascular diseases. According to studies using various HDAC inhibitors, several cardiovascular diseases would be benefit from novel indications for HDAC inhibitors including cardiac arrhythmia, myocardial infarction, global cardiac remodeling, HFpEF including hypertrophy, hypertension, and cardiac fibrosis. However, they may worsen vascular calcifications. Actually, thrombus is reported as a serious and quite frequent side effect of Vorinostat in patients.
To date, a great number of HDAC inhibitors are in clinical trials. Four drugs, Vorinostat, Romidepsin, Belinostat, and Panobinostat are already approved by the U.S. FDA for CTCL, PTCL, and multiple melanoma. Entinostat and VPA are in phase III trials for solid tumors. MGCD0103, PCI-24781, SB939, 4SC-201, ITF2357, CI-994, SRT501, and JNJ-26481585 are in phase II trials.
HDAC inhibitors represent a novel, promising regimen in various refractory diseases. Ubiquitous expression of various HDAC isoforms, however, should be noted when HDAC inhibitors are developed for certain diseases. Ideal HDAC inhibitors for cancer treatment must not block good HDACs but inhibit bad HDACs. In some case, HDAC10 is closely linked with suppression of cervical cancer metastasis.98 Hence, novel approaches should be considered to regulate bad HDACs specifically. We already reported that the inhibition of the HDAC2-activating enzyme, CK2α1, is as effective as an HDAC inhibitor in the development of cardiac hypertrophy. When regarding that HDACs generally regulated by post-translational modification novel therapeutics that target HDAC-regulating enzymes would be more ideal drugs. Novel therapeutics would be promising with the understanding of the certain PTM enzymes that regulate the specific HDACs.
ACKNOWLEDGEMENTS
The authors are grateful for critical comments by Dr. Hyun Kook of the Chonnam National University Medical School. This study was financially supported by Chonnam National University (2015-0663).
References
1. Holbert MA, Marmorstein R. Structure and activity of enzymes that remove histone modifications. Curr Opin Struct Biol. 2005; 15:673–680. PMID: 16263263.
2. Finnin MS, Donigian JR, Cohen A, Richon VM, Rifkind RA, Marks PA, et al. Structures of a histone deacetylase homologue bound to the TSA and SAHA inhibitors. Nature. 1999; 401:188–193. PMID: 10490031.
3. Smith KT, Workman JL. Histone deacetylase inhibitors: anticancer compounds. Int J Biochem Cell Biol. 2009; 41:21–25. PMID: 18845268.
4. Eom GH, Kook H. Posttranslational modifications of histone deacetylases: implications for cardiovascular diseases. Pharmacol Ther. 2014; 143:168–180. PMID: 24594235.
5. Choi JH, Kwon HJ, Yoon BI, Kim JH, Han SU, Joo HJ, et al. Expression profile of histone deacetylase 1 in gastric cancer tissues. Jpn J Cancer Res. 2001; 92:1300–1304. PMID: 11749695.
6. Wilson AJ, Byun DS, Popova N, Murray LB, L'Italien K, Sowa Y, et al. Histone deacetylase 3 (HDAC3) and other class I HDACs regulate colon cell maturation and p21 expression and are deregulated in human colon cancer. J Biol Chem. 2006; 281:13548–13558. PMID: 16533812.
7. Halkidou K, Gaughan L, Cook S, Leung HY, Neal DE, Robson CN. Upregulation and nuclear recruitment of HDAC1 in hormone refractory prostate cancer. Prostate. 2004; 59:177–189. PMID: 15042618.
8. Zhang Z, Yamashita H, Toyama T, Sugiura H, Ando Y, Mita K, et al. Quantitation of HDAC1 mRNA expression in invasive carcinoma of the breast. Breast Cancer Res Treat. 2005; 94:11–16. PMID: 16172792.
9. Huang BH, Laban M, Leung CH, Lee L, Lee CK, Salto-Tellez M, et al. Inhibition of histone deacetylase 2 increases apoptosis and p21Cip1/WAF1 expression, independent of histone deacetylase 1. Cell Death Differ. 2005; 12:395–404. PMID: 15665816.
10. Song J, Noh JH, Lee JH, Eun JW, Ahn YM, Kim SY, et al. Increased expression of histone deacetylase 2 is found in human gastric cancer. APMIS. 2005; 113:264–268. PMID: 15865607.
11. Zhu P, Martin E, Mengwasser J, Schlag P, Janssen KP, Göttlicher M. Induction of HDAC2 expression upon loss of APC in colorectal tumorigenesis. Cancer Cell. 2004; 5:455–463. PMID: 15144953.
12. Zhang Z, Yamashita H, Toyama T, Sugiura H, Omoto Y, Ando Y, et al. HDAC6 expression is correlated with better survival in breast cancer. Clin Cancer Res. 2004; 10:6962–6968. PMID: 15501975.
13. Myzak MC, Dashwood WM, Orner GA, Ho E, Dashwood RH. Sulforaphane inhibits histone deacetylase in vivo and suppresses tumorigenesis in Apc-minus mice. FASEB J. 2006; 20:506–508. PMID: 16407454.
14. Westendorf JJ, Zaidi SK, Cascino JE, Kahler R, van Wijnen AJ, Lian JB, et al. Runx2 (Cbfa1, AML-3) interacts with histone deacetylase 6 and represses the p21(CIP1/WAF1) promoter. Mol Cell Biol. 2002; 22:7982–7992. PMID: 12391164.
15. Archer SY, Johnson J, Kim HJ, Ma Q, Mou H, Daesety V, et al. The histone deacetylase inhibitor butyrate downregulates cyclin B1 gene expression via a p21/WAF-1-dependent mechanism in human colon cancer cells. Am J Physiol Gastrointest Liver Physiol. 2005; 289:G696–G703. PMID: 16160080.
16. Alao JP, Stavropoulou AV, Lam EW, Coombes RC, Vigushin DM. Histone deacetylase inhibitor, trichostatin A induces ubiquitin-dependent cyclin D1 degradation in MCF-7 breast cancer cells. Mol Cancer. 2006; 5:8. PMID: 16504004.
17. Caslini C, Capo-chichi CD, Roland IH, Nicolas E, Yeung AT, Xu XX. Histone modifications silence the GATA transcription factor genes in ovarian cancer. Oncogene. 2006; 25:5446–5461. PMID: 16607277.
18. Linggi B, Müller-Tidow C, van de Locht L, Hu M, Nip J, Serve H, et al. The t(8;21) fusion protein, AML1 ETO, specifically represses the transcription of the p14(ARF) tumor suppressor in acute myeloid leukemia. Nat Med. 2002; 8:743–750. PMID: 12091906.
19. Follows GA, Tagoh H, Lefevre P, Hodge D, Morgan GJ, Bonifer C. Epigenetic consequences of AML1-ETO action at the human c-FMS locus. EMBO J. 2003; 22:2798–2809. PMID: 12773394.
20. Lemercier C, Brocard MP, Puvion-Dutilleul F, Kao HY, Albagli O, Khochbin S. Class II histone deacetylases are directly recruited by BCL6 transcriptional repressor. J Biol Chem. 2002; 277:22045–22052. PMID: 11929873.
21. Kim MS, Kwon HJ, Lee YM, Baek JH, Jang JE, Lee SW, et al. Histone deacetylases induce angiogenesis by negative regulation of tumor suppressor genes. Nat Med. 2001; 7:437–443. PMID: 11283670.
22. Kim SH, Ahn S, Han JW, Lee HW, Lee HY, Lee YW, et al. Apicidin is a histone deacetylase inhibitor with anti-invasive and anti-angiogenic potentials. Biochem Biophys Res Commun. 2004; 315:964–970. PMID: 14985106.
23. Michaelis M, Michaelis UR, Fleming I, Suhan T, Cinatl J, Blaheta RA, et al. Valproic acid inhibits angiogenesis in vitro and in vivo. Mol Pharmacol. 2004; 65:520–527. PMID: 14978230.
24. Park SY, Jun JA, Jeong KJ, Heo HJ, Sohn JS, Lee HY, et al. Histone deacetylases 1, 6 and 8 are critical for invasion in breast cancer. Oncol Rep. 2011; 25:1677–1681. PMID: 21455583.
25. Peinado H, Ballestar E, Esteller M, Cano A. Snail mediates E-cadherin repression by the recruitment of the Sin3A/histone deacetylase 1 (HDAC1)/HDAC2 complex. Mol Cell Biol. 2004; 24:306–319. PMID: 14673164.
26. Annicotte JS, Iankova I, Miard S, Fritz V, Sarruf D, Abella A, et al. Peroxisome proliferator-activated receptor gamma regulates E-cadherin expression and inhibits growth and invasion of prostate cancer. Mol Cell Biol. 2006; 26:7561–7574. PMID: 17015477.
27. McGarry LC, Winnie JN, Ozanne BW. Invasion of v-Fos (FBR)-transformed cells is dependent upon histone deacetylase activity and suppression of histone deacetylase regulated genes. Oncogene. 2004; 23:5284–5292. PMID: 15107823.
28. Kuwajima A, Iwashita J, Murata J, Abe T. The histone deacetylase inhibitor butyrate inhibits melanoma cell invasion of Matrigel. Anticancer Res. 2007; 27:4163–4169. PMID: 18225587.
29. Weaver BA, Cleveland DW. Does aneuploidy cause cancer? Curr Opin Cell Biol. 2006; 18:658–667. PMID: 17046232.
30. Gemoll T, Roblick UJ, Szymczak S, Braunschweig T, Becker S, Igl BW, et al. HDAC2 and TXNL1 distinguish aneuploid from diploid colorectal cancers. Cell Mol Life Sci. 2011; 68:3261–3274. PMID: 21290163.
31. Olaharski AJ, Ji Z, Woo JY, Lim S, Hubbard AE, Zhang L, et al. The histone deacetylase inhibitor trichostatin a has genotoxic effects in human lymphoblasts in vitro. Toxicol Sci. 2006; 93:341–347. PMID: 16857700.
32. Mann BS, Johnson JR, Cohen MH, Justice R, Pazdur R. FDA approval summary: vorinostat for treatment of advanced primary cutaneous T-cell lymphoma. Oncologist. 2007; 12:1247–1252. PMID: 17962618.
33. Marks PA, Xu WS. Histone deacetylase inhibitors: potential in cancer therapy. J Cell Biochem. 2009; 107:600–608. PMID: 19459166.
34. Whittaker SJ, Demierre MF, Kim EJ, Rook AH, Lerner A, Duvic M, et al. Final results from a multicenter, international, pivotal study of romidepsin in refractory cutaneous T-cell lymphoma. J Clin Oncol. 2010; 28:4485–4491. PMID: 20697094.
35. Coiffier B, Pro B, Prince HM, Foss F, Sokol L, Greenwood M, et al. Results from a pivotal, open-label, phase II study of romidepsin in relapsed or refractory peripheral T-cell lymphoma after prior systemic therapy. J Clin Oncol. 2012; 30:631–636. PMID: 22271479.
36. Poole RM. Belinostat: first global approval. Drugs. 2014; 74:1543–1554. PMID: 25134672.
37. Cashen A, Juckett M, Jumonville A, Litzow M, Flynn PJ, Eckardt J, et al. Phase II study of the histone deacetylase inhibitor belinostat (PXD101) for the treatment of myelodysplastic syndrome (MDS). Ann Hematol. 2012; 91:33–38. PMID: 21538061.
38. Oki Y, Buglio D, Fanale M, Fayad L, Copeland A, Romaguera J, et al. Phase I study of panobinostat plus everolimus in patients with relapsed or refractory lymphoma. Clin Cancer Res. 2013; 19:6882–6890. PMID: 24097867.
39. Marchion D, Münster P. Development of histone deacetylase inhibitors for cancer treatment. Expert Rev Anticancer Ther. 2007; 7:583–598. PMID: 17428177.
40. Kee HJ, Kwon JS, Shin S, Ahn Y, Jeong MH, Kook H. Trichostatin A prevents neointimal hyperplasia via activation of Krüppel like factor 4. Vascul Pharmacol. 2011; 55:127–134. PMID: 21763782.
41. Findeisen HM, Gizard F, Zhao Y, Qing H, Heywood EB, Jones KL, et al. Epigenetic regulation of vascular smooth muscle cell proliferation and neointima formation by histone deacetylase inhibition. Arterioscler Thromb Vasc Biol. 2011; 31:851–860. PMID: 21233448.
42. Okamoto H, Fujioka Y, Takahashi A, Takahashi T, Taniguchi T, Ishikawa Y, et al. Trichostatin A, an inhibitor of histone deacetylase, inhibits smooth muscle cell proliferation via induction of p21(WAF1). J Atheroscler Thromb. 2006; 13:183–191. PMID: 16908950.
43. Halili MA, Andrews MR, Labzin LI, Schroder K, Matthias G, Cao C, et al. Differential effects of selective HDAC inhibitors on macrophage inflammatory responses to the Toll-like receptor 4 agonist LPS. J Leukoc Biol. 2010; 87:1103–1114. PMID: 20200406.
44. Ismat FA, Zhang M, Kook H, Huang B, Zhou R, Ferrari VA, et al. Homeobox protein Hop functions in the adult cardiac conduction system. Circ Res. 2005; 96:898–903. PMID: 15790958.
45. Kook H, Lepore JJ, Gitler AD, Lu MM, Wing-Man Yung W, Mackay J, et al. Cardiac hypertrophy and histone deacetylase-dependent transcriptional repression mediated by the atypical homeodomain protein Hop. J Clin Invest. 2003; 112:863–871. PMID: 12975471.
46. Montgomery RL, Davis CA, Potthoff MJ, Haberland M, Fielitz J, Qi X, et al. Histone deacetylases 1 and 2 redundantly regulate cardiac morphogenesis, growth, and contractility. Genes Dev. 2007; 21:1790–1802. PMID: 17639084.
47. Monteforte N, Napolitano C, Priori SG. Genetics and arrhythmias: diagnostic and prognostic applications. Rev Esp Cardiol (Engl Ed). 2012; 65:278–286. PMID: 22245453.
48. Zhao TC, Cheng G, Zhang LX, Tseng YT, Padbury JF. Inhibition of histone deacetylases triggers pharmacologic preconditioning effects against myocardial ischemic injury. Cardiovasc Res. 2007; 76:473–481. PMID: 17884027.
49. Granger A, Abdullah I, Huebner F, Stout A, Wang T, Huebner T, et al. Histone deacetylase inhibition reduces myocardial ischemia-reperfusion injury in mice. FASEB J. 2008; 22:3549–3560. PMID: 18606865.
50. Lee TM, Lin MS, Chang NC. Inhibition of histone deacetylase on ventricular remodeling in infarcted rats. Am J Physiol Heart Circ Physiol. 2007; 293:H968–H977. PMID: 17400721.
51. Ramjiawan A, Bagchi RA, Blant A, Albak L, Cavasin MA, Horn TR, et al. Roles of histone deacetylation and AMP kinase in regulation of cardiomyocyte PGC-1α gene expression in hypoxia. Am J Physiol Cell Physiol. 2013; 304:C1064–C1072. PMID: 23515531.
52. Zhang L, Chen B, Zhao Y, Dubielecka PM, Wei L, Qin GJ, et al. Inhibition of histone deacetylase-induced myocardial repair is mediated by c-kit in infarcted hearts. J Biol Chem. 2012; 287:39338–39348. PMID: 23024362.
53. Frey N, Olson EN. Cardiac hypertrophy: the good, the bad, and the ugly. Annu Rev Physiol. 2003; 65:45–79. PMID: 12524460.
54. Cho YK, Eom GH, Kee HJ, Kim HS, Choi WY, Nam KI, et al. Sodium valproate, a histone deacetylase inhibitor, but not captopril, prevents right ventricular hypertrophy in rats. Circ J. 2010; 74:760–770. PMID: 20208383.
55. Eom GH, Cho YK, Ko JH, Shin S, Choe N, Kim Y, et al. Casein kinase-2α1 induces hypertrophic response by phosphorylation of histone deacetylase 2 S394 and its activation in the heart. Circulation. 2011; 123:2392–2403. PMID: 21576649.
56. Kee HJ, Eom GH, Joung H, Shin S, Kim JR, Cho YK, et al. Activation of histone deacetylase 2 by inducible heat shock protein 70 in cardiac hypertrophy. Circ Res. 2008; 103:1259–1269. PMID: 18849323.
57. Kee HJ, Kook H. Krüppel-like factor 4 mediates histone deacetylase inhibitor-induced prevention of cardiac hypertrophy. J Mol Cell Cardiol. 2009; 47:770–780. PMID: 19729022.
58. Kee HJ, Sohn IS, Nam KI, Park JE, Qian YR, Yin Z, et al. Inhibition of histone deacetylation blocks cardiac hypertrophy induced by angiotensin II infusion and aortic banding. Circulation. 2006; 113:51–59. PMID: 16380549.
59. Chang S, McKinsey TA, Zhang CL, Richardson JA, Hill JA, Olson EN. Histone deacetylases 5 and 9 govern responsiveness of the heart to a subset of stress signals and play redundant roles in heart development. Mol Cell Biol. 2004; 24:8467–8476. PMID: 15367668.
60. Kong Y, Tannous P, Lu G, Berenji K, Rothermel BA, Olson EN, et al. Suppression of class I and II histone deacetylases blunts pressure-overload cardiac hypertrophy. Circulation. 2006; 113:2579–2588. PMID: 16735673.
61. Vega RB, Harrison BC, Meadows E, Roberts CR, Papst PJ, Olson EN, et al. Protein kinases C and D mediate agonist-dependent cardiac hypertrophy through nuclear export of histone deacetylase 5. Mol Cell Biol. 2004; 24:8374–8385. PMID: 15367659.
62. Zhang CL, McKinsey TA, Chang S, Antos CL, Hill JA, Olson EN. Class II histone deacetylases act as signal-responsive repressors of cardiac hypertrophy. Cell. 2002; 110:479–488. PMID: 12202037.
63. Gallo P, Latronico MV, Gallo P, Grimaldi S, Borgia F, Todaro M, et al. Inhibition of class I histone deacetylase with an apicidin derivative prevents cardiac hypertrophy and failure. Cardiovasc Res. 2008; 80:416–424. PMID: 18697792.
64. Trivedi CM, Luo Y, Yin Z, Zhang M, Zhu W, Wang T, et al. Hdac2 regulates the cardiac hypertrophic response by modulating Gsk3 beta activity. Nat Med. 2007; 13:324–331. PMID: 17322895.
65. Trivedi CM, Lu MM, Wang Q, Epstein JA. Transgenic overexpression of Hdac3 in the heart produces increased postnatal cardiac myocyte proliferation but does not induce hypertrophy. J Biol Chem. 2008; 283:26484–26489. PMID: 18625706.
66. McKinsey TA, Zhang CL, Olson EN. Activation of the myocyte enhancer factor-2 transcription factor by calcium/calmodulin-dependent protein kinase-stimulated binding of 14-3-3 to histone deacetylase 5. Proc Natl Acad Sci U S A. 2000; 97:14400–14405. PMID: 11114197.
67. Cavasin MA, Demos-Davies K, Horn TR, Walker LA, Lemon DD, Birdsey N, et al. Selective class I histone deacetylase inhibition suppresses hypoxia-induced cardiopulmonary remodeling through an antiproliferative mechanism. Circ Res. 2012; 110:739–748. PMID: 22282194.
68. Eom GH, Nam YS, Oh JG, Choe N, Min HK, Yoo EK, et al. Regulation of acetylation of histone deacetylase 2 by p300/CBPassociated factor/histone deacetylase 5 in the development of cardiac hypertrophy. Circ Res. 2014; 114:1133–1143. PMID: 24526703.
69. Lee HA, Lee DY, Cho HM, Kim SY, Iwasaki Y, Kim IK. Histone deacetylase inhibition attenuates transcriptional activity of mineralocorticoid receptor through its acetylation and prevents development of hypertension. Circ Res. 2013; 112:1004–1012. PMID: 23421989.
70. Usui T, Okada M, Mizuno W, Oda M, Ide N, Morita T, et al. HDAC4 mediates development of hypertension via vascular inflammation in spontaneous hypertensive rats. Am J Physiol Heart Circ Physiol. 2012; 302:H1894–H1904. PMID: 22389387.
71. Schiattarella GG, Hill JA. Inhibition of hypertrophy is a good therapeutic strategy in ventricular pressure overload. Circulation. 2015; 131:1435–1447. PMID: 25901069.
72. Guo W, Shan B, Klingsberg RC, Qin X, Lasky JA. Abrogation of TGF-beta1-induced fibroblast-myofibroblast differentiation by histone deacetylase inhibition. Am J Physiol Lung Cell Mol Physiol. 2009; 297:L864–L870. PMID: 19700647.
73. Fitzgerald O'Connor EJ, Badshah II, Addae LY, Kundasamy P, Thanabalasingam S, Abioye D, et al. Histone deacetylase 2 is upregulated in normal and keloid scars. J Invest Dermatol. 2012; 132:1293–1296. PMID: 22205303.
74. Noh H, Oh EY, Seo JY, Yu MR, Kim YO, Ha H, et al. Histone deacetylase-2 is a key regulator of diabetes- and transforming growth factor-beta1-induced renal injury. Am J Physiol Renal Physiol. 2009; 297:F729–F739. PMID: 19553350.
75. Marumo T, Hishikawa K, Yoshikawa M, Hirahashi J, Kawachi S, Fujita T. Histone deacetylase modulates the proinflammatory and -fibrotic changes in tubulointerstitial injury. Am J Physiol Renal Physiol. 2010; 298:F133–F141. PMID: 19906951.
76. Deroanne CF, Bonjean K, Servotte S, Devy L, Colige A, Clausse N, et al. Histone deacetylases inhibitors as anti-angiogenic agents altering vascular endothelial growth factor signaling. Oncogene. 2002; 21:427–436. PMID: 11821955.
77. Geng H, Harvey CT, Pittsenbarger J, Liu Q, Beer TM, Xue C, et al. HDAC4 protein regulates HIF1α protein lysine acetylation and cancer cell response to hypoxia. J Biol Chem. 2011; 286:38095–38102. PMID: 21917920.
78. Urbich C, Rössig L, Kaluza D, Potente M, Boeckel JN, Knau A, et al. HDAC5 is a repressor of angiogenesis and determines the angiogenic gene expression pattern of endothelial cells. Blood. 2009; 113:5669–5679. PMID: 19351956.
79. Kaluza D, Kroll J, Gesierich S, Yao TP, Boon RA, Hergenreider E, et al. Class IIb HDAC6 regulates endothelial cell migration and angiogenesis by deacetylation of cortactin. EMBO J. 2011; 30:4142–4156. PMID: 21847094.
80. Li D, Xie S, Ren Y, Huo L, Gao J, Cui D, et al. Microtubule-associated deacetylase HDAC6 promotes angiogenesis by regulating cell migration in an EB1-dependent manner. Protein Cell. 2011; 2:150–160. PMID: 21359602.
81. Mottet D, Bellahcène A, Pirotte S, Waltregny D, Deroanne C, Lamour V, et al. Histone deacetylase 7 silencing alters endothelial cell migration, a key step in angiogenesis. Circ Res. 2007; 101:1237–1246. PMID: 17947801.
82. Margariti A, Zampetaki A, Xiao Q, Zhou B, Karamariti E, Martin D, et al. Histone deacetylase 7 controls endothelial cell growth through modulation of beta-catenin. Circ Res. 2010; 106:1202–1211. PMID: 20224040.
83. Wang Z, Tsai LK, Munasinghe J, Leng Y, Fessler EB, Chibane F, et al. Chronic valproate treatment enhances postischemic angiogenesis and promotes functional recovery in a rat model of ischemic stroke. Stroke. 2012; 43:2430–2436. PMID: 22811460.
84. Zhang L, Qin X, Zhao Y, Fast L, Zhuang S, Liu P, et al. Inhibition of histone deacetylases preserves myocardial performance and prevents cardiac remodeling through stimulation of endogenous angiomyogenesis. J Pharmacol Exp Ther. 2012; 341:285–293. PMID: 22271820.
85. Ellis L, Hammers H, Pili R. Targeting tumor angiogenesis with histone deacetylase inhibitors. Cancer Lett. 2009; 280:145–153. PMID: 19111391.
86. Azechi T, Kanehira D, Kobayashi T, Sudo R, Nishimura A, Sato F, et al. Trichostatin A, an HDAC class I/II inhibitor, promotes Pi-induced vascular calcification via up-regulation of the expression of alkaline phosphatase. J Atheroscler Thromb. 2013; 20:538–547. PMID: 23518467.
87. Sachweh MC, Drummond CJ, Higgins M, Campbell J, Laín S. Incompatible effects of p53 and HDAC inhibition on p21 expression and cell cycle progression. Cell Death Dis. 2013; 4:e533. PMID: 23470540.
88. Fischer A, Sananbenesi F, Wang X, Dobbin M, Tsai LH. Recovery of learning and memory is associated with chromatin remodelling. Nature. 2007; 447:178–182. PMID: 17468743.
89. Qing H, He G, Ly PT, Fox CJ, Staufenbiel M, Cai F, et al. Valproic acid inhibits Abeta production, neuritic plaque formation, and behavioral deficits in Alzheimer's disease mouse models. J Exp Med. 2008; 205:2781–2789. PMID: 18955571.
90. Kim HJ, Rowe M, Ren M, Hong JS, Chen PS, Chuang DM. Histone deacetylase inhibitors exhibit anti-inflammatory and neuroprotective effects in a rat permanent ischemic model of stroke: multiple mechanisms of action. J Pharmacol Exp Ther. 2007; 321:892–901. PMID: 17371805.
91. Hockly E, Richon VM, Woodman B, Smith DL, Zhou X, Rosa E, et al. Suberoylanilide hydroxamic acid, a histone deacetylase inhibitor, ameliorates motor deficits in a mouse model of Huntington's disease. Proc Natl Acad Sci U S A. 2003; 100:2041–2046. PMID: 12576549.
92. Hanson JE, La H, Plise E, Chen YH, Ding X, Hanania T, et al. SAHA enhances synaptic function and plasticity in vitro but has limited brain availability in vivo and does not impact cognition. PLoS One. 2013; 8:e69964. PMID: 23922875.
93. Cuadrado-Tejedor M, Garcia-Barroso C, Sanzhez-Arias J, Mederos S, Rabal O, Ugarte A, et al. Concomitant histone deacetylase and phosphodiesterase 5 inhibition synergistically prevents the disruption in synaptic plasticity and it reverses cognitive impairment in a mouse model of Alzheimer's disease. Clin Epigenetics. 2015; 7:108. PMID: 26457123.
94. Bieszczad KM, Bechay K, Rusche JR, Jacques V, Kudugunti S, Miao W, et al. Histone deacetylase inhibition via RGFP966 releases the brakes on sensory cortical plasticity and the specificity of memory formation. J Neurosci. 2015; 35:13124–13132. PMID: 26400942.
95. Guan JS, Haggarty SJ, Giacometti E, Dannenberg JH, Joseph N, Gao J, et al. HDAC2 negatively regulates memory formation and synaptic plasticity. Nature. 2009; 459:55–60. PMID: 19424149.
96. Jeon EJ, Lee KY, Choi NS, Lee MH, Kim HN, Jin YH, et al. Bone morphogenetic protein-2 stimulates Runx2 acetylation. J Biol Chem. 2006; 281:16502–16511. PMID: 16613856.
97. Nasu Y, Nishida K, Miyazawa S, Komiyama T, Kadota Y, Abe N, et al. Trichostatin A, a histone deacetylase inhibitor, suppresses synovial inflammation and subsequent cartilage destruction in a collagen antibody-induced arthritis mouse model. Osteoarthritis Cartilage. 2008; 16:723–732. PMID: 18226559.
98. Song C, Zhu S, Wu C, Kang J. Histone deacetylase (HDAC) 10 suppresses cervical cancer metastasis through inhibition of matrix metalloproteinase (MMP) 2 and 9 expression. J Biol Chem. 2013; 288:28021–28033. PMID: 23897811.
FIG. 1
The role of HDACs in cancer biology. Generally HDACs participate in the expression of malignant phenotypes in cancer cells. Class I HDACs promote cell proliferation and inhibit both apoptosis and differentiation. Class II HDACs provoke tumor angiogenesis. HDAC6 specifically increase in cell motility which results in distant metastasis. The bar indicates suppression whereas the arrow depicts stimulation.
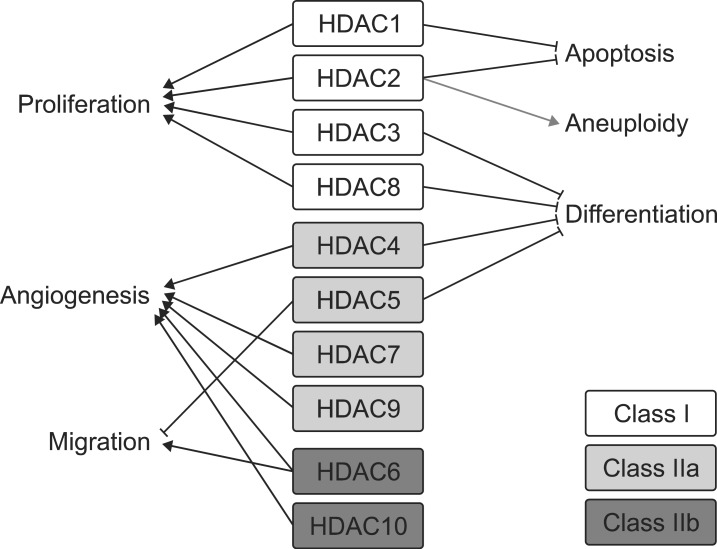
FIG. 2
The therapeutic potential of HDAC inhibitors in cardiovascular disease. HDAC inhibitors have been reported to show beneficial outcomes for cardiac arrhythmia, cardiac fibrosis, cardiac hypertrophy, and myocardial infarction. Although several debates in atherosclerosis, HDAC inhibitors also significantly reduce the progression of atherosclerosis. Vascular calcification, however, may be aggravated by HDAC inhibitors. The bar indicates suppression whereas the arrow depicts stimulation. The dash arrow reflects controversial effects.
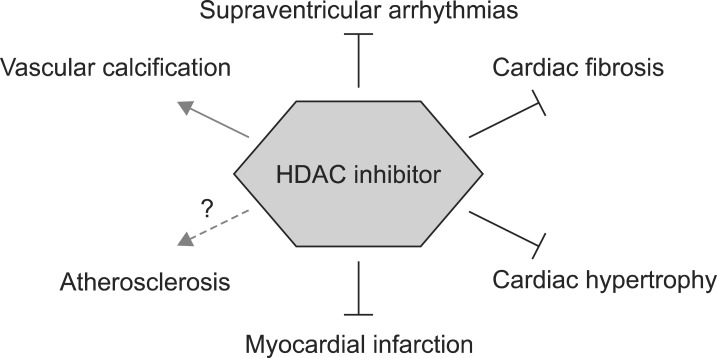
TABLE 1
HDAC inhibitors approved by United States Food and Drug Administration
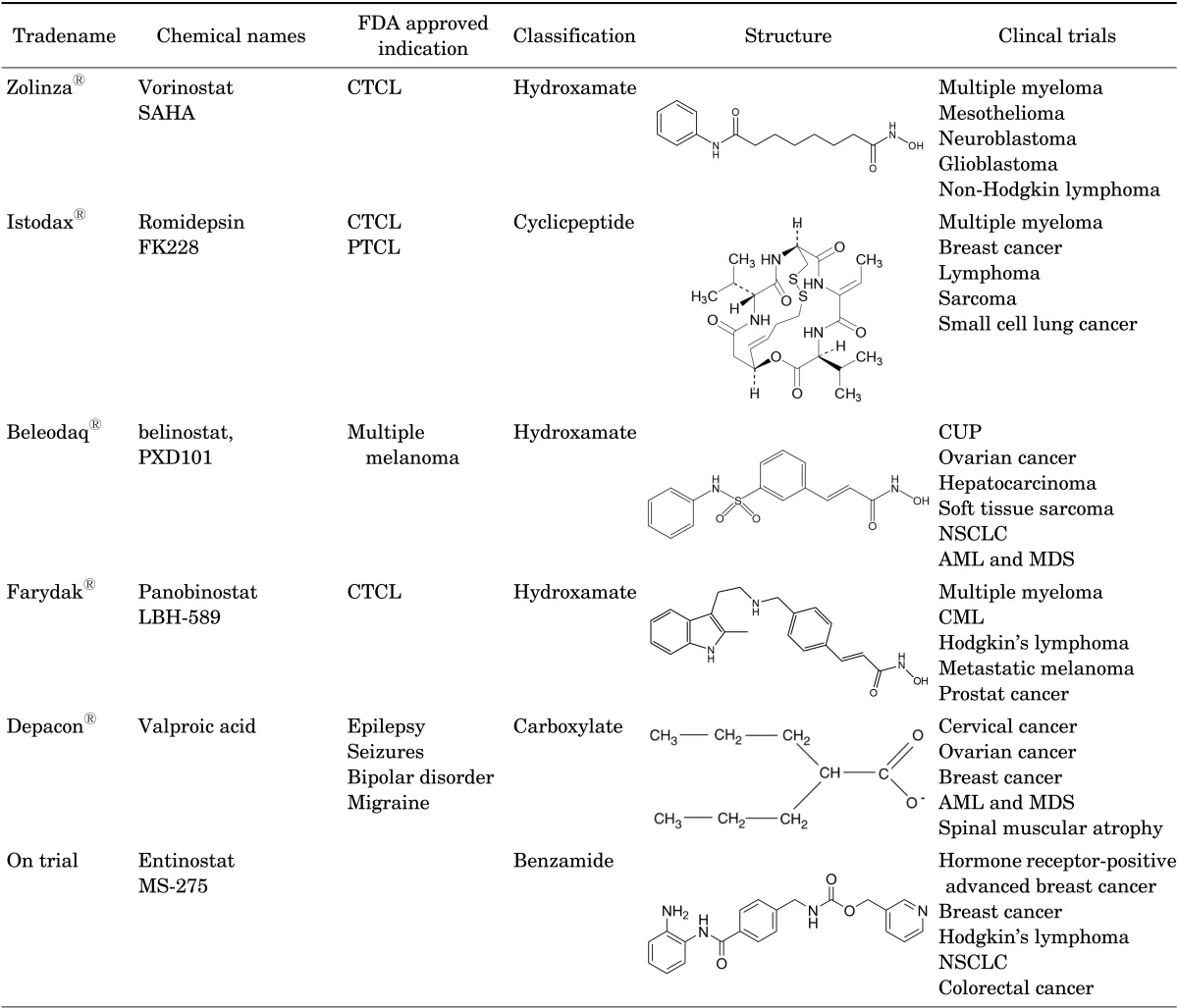
The FDA approved HDAC inhibitors are listed by chemical names, indications, classification, structures, and ongoing clinical trials. Approved Information was cited from Drugs@FDA and http://www.clinicaltrial.gov.
AML: acute myeloid leukemia, CML: chronic myeloid leukemia, CTCL: cutaneous T-cell lymphoma, CUP: carcinoma of unknown primary site, MDS: myelodysplastic syndrome, NSCLC: non-small cell lung cancer, PTCL: peripheral T-cell lymphoma.