Abstract
PURPOSE
This study evaluated the initial stability of different implants placed above the bone level in different types of bone.
MATERIALS AND METHODS
As described by Lekholm and Zarb, cortical layers of bovine bone specimens were trimmed to a thickness of 2 mm, 1 mm or totally removed to reproduce bone types II, III, and IV respectively. Three Implant system (Brånemark System® Mk III TiUnite™, Straumann Standard Implant SLA®, and Astra Tech Microthread™-OsseoSpeed™) were tested. Control group implants were placed in level with the bone, while test group implants were placed 1, 2, 3, and 4 mm above the bone level. Initial stability was evaluated by resonance frequency analysis. Data was statistically analyzed by one-way analysis of variance in confidence level of 95%. The effective implant length and the Implant Stability Quotient (ISQ) were compared using simple linear regression analysis.
RESULTS
In the control group, there was a significant difference in the ISQ values of the 3 implants in bone types III and IV (P<.05). The ISQ values of each implant decreased with increased effective implant length in all types of bone. In type II bone, the decrease in ISQ value per 1-mm increase in effective implant length of the Brånemark and Astra implants was less than that of the Straumann implant. In bone types III and IV, this value in the Astra implant was less than that in the other 2 implants.
Dental implants are frequently used to restore partially or totally edentulous dental arch. Achievement and maintenance of initial stability is an important factor for implant success.1-3 Many efforts have been made to achieve good implant stability such as development of implants with different designs,4,5 alteration of surface characteristics,6 and modification of surgical technique. Recently, such attempts have shifted toward changes in overall implant design to improve initial stability,4 which is also influenced by bone quality. A report by Ostman et al.7 examined the correlation between bone quality and primary stability, concluding that initial stability was lower in soft bone types than in hard bone types, as well as in the posterior compared to the anterior jaw.8
Several methods have been developed to evaluate implant stability, including resonance frequency analysis (RFA), developed by Meredith et al.9 This method originally comprised an L-shaped transducer tightened to the abutment or implant. Two piezoceramic components attach to the vertical beam of the transducer: one is vibrated with a sinusoidal wave of 5 to 15 kHz and the first flexural resonance frequency is identified as the peak. Osstell™ (Integration Diagnostics AB, Göteborg, Sweden) presents the resonance frequency value as an Implant Stability Quotient (ISQ) value on a scale from 3,500 Hz (1 ISQ unit) to 8,500 Hz (100 ISQ units).10
Implants may be placed above the bone level in clinical situations such as reduced bone height, the mandibular alveolar nerve, or a vertical bone defect. There may be less initial stability in such situations than when the implant is placed in the same level with the bone. Meredith et al.11 reported that the total exposed implant length above the bone level influenced the resonance frequency value, referring to it as the effective implant length.
Although there are many studies on the initial stability of implants placed in level with the bone,4,7,8 but there are few on the initial stability of implants placed above the bone level. Therefore, this study evaluated the initial stability of implants with different designs placed above bone level in different types of bone. Initial stability of implant was evaluated using resonance frequency analysis.
Fresh bovine rib bones were trimmed to dimensions of 350 × 150 × 150 mm (length, width, height). As described by Lekholm and Zarb in 1985,12 the cortical layers of the bone specimens were trimmed to a thickness of 2 mm, 1 mm, or totally removed to reproduce bone types II, III, or IV, respectively.
The trimmed bone specimens were embedded in cold curing acrylic resin (Trayplast®, Vertex-Dental BV, Netherlands, 600 × 300 × 10 mm) to approximately 1/4 of their height (Fig. 1). The acrylic resin was mixed with a liquid-to-powder ratio of 1:5 (by volume) according to the manufacturer's instruction. After the mixture reached the initial setting stage, the specimens were immersed in cold 0.9% saline to eliminate the heat generated during the setting stage. The specimens were stored in the freezer and immersed in saline approximately 30 minutes before placement.
Three types of implants were tested: Brånemark System® Mk III TiUnite™ (Nobel Biocare™, Göteborg, Sweden), Straumann Standard Implant SLA® (Institut Straumann AG, Waldenburg, Switzerland), and Astra Tech Microthread™-OsseoSpeed™ (Astra Tech Dental, Mölndahl, Sweden). Brånemark System® Mk III TiUnite™ was 4.0 mm in diameter and 8.5 mm in length, Straumann Standard Implant SLA® was 4.1 mm in diameter and 8.0 mm in length with a 2.8-mm smooth neck section, and Astra Tech Microthread™-OsseoSpeed™ was 4.0 mm in diameter and 9.0 mm in length. We followed the manufacturer's instructions for site preparation and implant placement. First, ø2-mm start drill was used for all implants. For the Brånemark implant in bone types II and III, ø3.15-mm twist drill and counterbore were used in the final stage, while a screw tap was used in type II bone only. For the Straumann implant in bone types II and III, ø3.5-mm twist drill and ø4.1-mm tap drill were used in the final stage. For the Astra implant, ø3.7-mm tiger drill and ø4.0-mm cortical drill were used in the final stage in type II bone, while ø3.85-mm tiger drill was used in the final stage in type III bone. Final site preparations in type IV bone were done by osteotome (Osung MND Co., Seoul, Korea) for all implants: ø3.0-mm osteotome for the Brånemark implant and ø3.5-mm osteotome for Straumann and Astra implants.
In the control group, the platform of the Brånemark implant, the junction of the Straumann Implant's smooth neck section, and the top portion of the Astra implant's polished collar were placed in level with the bone. Drillings were done 1, 2, 3, and 4 mm short and implants were placed 1, 2, 3, and 4 mm above the bone level. As a result, the test groups had different effective implant lengths (Fig. 2). Ten implants of each type were placed at each effective implant length in the different bone types. Site preparation was performed at 1500 rpm and implant placement at 30 rpm with saline irrigation by INTRAsurg® 300 (KaVo dental GmbH, Biberach/Riβ, Germany).
Following implant placement, the resonance frequency was measured by Osstell™ mentor (Integration Diagnostics Ltd., Göteborg, Sweden) and Smartpeg™ (Integration Diagnostics Ltd., Göteborg, Sweden). Smartpeg™ was selected according to the Smartpeg™ reference list, manually tightened to the implant, and measurements were taken at points A and B (Fig. 3). The higher ISQ value of the two points was used.
One-way variance analysis was used to compare ISQ values of the implants. When a significant difference was found, Tukey's B multiple comparison test was performed. A P value of <0.05 was considered significant. A simple linear regression test was performed between ISQ value and effective implant length to reveal linear relationships.
The ISQ values of the different effective implant lengths of the Brånemark implant are compared in Table 1. The ISQ values were significantly different between different effective lengths in all types of bone (P=.000), decreasing as the effective implant length increased.
The results of simple linear regression are presented in Table 2. Simple linear regression was performed to ascertain whether changes in ISQ value could be explained by increased effective implant length, which was represented by r-squared in this statistical model. If r-squared = 1, changes in ISQ could indeed be explained by increased effective implant length. "Unstandardized coefficient B" represents the change in ISQ value for each 1-mm increase in effective implant length. This coefficient decreased from type II (-3.450) to type IV (-7.380) bone.
The ISQ values of the different effective implant lengths of the Straumann implant are compared in Table 3. The ISQ values were significantly different between different effective lengths in all types of bone (P=.000), generally decreasing as the effective implant length increased. The results of simple linear regression are presented in Table 4. The B coefficient decreased from type II (-5.160) to type IV (-6.420) bone.
The ISQ values of the different effective implant lengths of the Astra implant are compared in Table 5. The ISQ values were significantly different between different effective implant lengths in all types of bone (P=.000), decreasing as the effective implant length increased. The results of simple linear regression are presented in Table 6. The B coefficient value decreased from type II (-3.210) to type IV (-5.870) bone.
The ISQ values of the control group are compared. In type II bone, the ISQ values were not significantly different among the 3 implant types (P >.05). In type III bone, the ISQ value of the Brånemark implant was greater than that of the Straumann implant (P <.05). In type IV bone, the ISQ value of the Straumann implant was less than that of the other 2 implants (P=.000). In the 1-mm effective implant length group, ISQ values were not significantly different among the 3 implants in type II bone (P >.05). The ISQ values of the Straumann implant in bone types III and IV were significantly less than that of the other 2 implants (P=.000). In the 2-mm effective implant length group, the ISQ values of the Brånemark implant were significantly greater than that of the Straumann implant in bone types II and III (P <.05). The ISQ value of the Straumann implant was significantly less than that of other 2 implants in type IV bone (P=.002).
Implant stability is an important clinical parameter, especially when there are anatomical limitations such as the proximity of mandibular nerve. In that case, implant might be placed above the bone level and simultaneous bone graft could be done. In this study, implants were placed above the bone level to elucidate how the increased effective implant length affected the initial stability of implants with different designs. Although specifications differed among implants, this study focused on the relationship between effective implant length and initial stability.
Brånemark System® Mk III TiUnite™ is designed to place the platform in the same level with the bone. Although it has a self-tapping design, tapping procedure is recommended in type II bone. Straumann Standard Implant SLA® with a 2.8-mm smooth neck section has a non-self tapping design. Astra Tech Microthread™-OsseoSpeed™ has an approximately 3 mm-long Microthread™ in its upper portion that the manufacturer claims increase its functional load bearing capacity, resulting in optimal load distribution and preservation of marginal bone. Surgical procedures were performed according to manufacturer's instructions. In type IV bone, site preparations were done by the osteotome to maximize the initial stability.
There are several methods to evaluate implant stability. The percussion test13 is a simple method applied by tapping the fixture mount or healing abutment with a blunt instrument, but it does not yield detailed information on the range of possible stabilities. A cutting-resistance measurement can assess bone quality at the time of implant placement14-16 and inform the possibility of achieving good primary stability. Periotest® (Siemens AG, Bensheim, Germany) was introduced to measure implant mobility.17-23 It yields relatively low values in a narrow range when measuring stable implants,19 and results are influenced by the handpiece angulation, the vertical measuring point on the implant abutment, and the horizontal distance between the handpiece and the implant.24
The removal torque test immediately after the placement is used to evaluate the probability of achieving initial stability. Niimi et al. 25 used this method to evaluate the bone quality and the cortical bone thickness of different sites. They reported a significant correlation between cortical bone thickness and removal torque.
Resonance frequency analysis was first presented by Meredith et al.9 This method allows clinical measurements of implant stability11,26,27 and can be used as a prognostic determinant28 to individualize implant treatment with regard to healing periods, the type of prosthetic construction and if one- or two-staged procedures should be used.29 In addition, the change in implant stability during loading can be monitored. The resonance frequency value is influenced by the stiffness of bone, the implant components, and the bone-implant interface9 as well as by the distance from the transducer to the first bone-implant contact.11,26,27,29 In this study, ISQ value was used as a parameter of implant initial stability.
The ISQ value of the Straumann implant was approximately 10 units lower than the other implants measured by the original device (Osstell™), a difference attributable to the supracrestal shoulder of the implant.30 The Osstell™ mentor compensated for this phenomenon by keeping the total effective implant length equal, allowing for direct comparison of the ISQ values of different implants.
Sennerby and Meredith29 reported that ISQ values were lower in implants placed above bone level even though they have the same stability. The authors found this difference to be approximately 2-3 ISQ units per 1 mm effective implant length (2 in softer bone and 3 in harder bone). Meredith et al.27 reported a strong correlation between supracrestal implant height and RFA value.
R-squared values of all implants were high in all bone types in this study, suggesting that the changes in ISQ value could be explained by this linear regression model.
The ISQ values of the Brånemark implant decreased with increasing effective implant length. The B coefficient represents the change in ISQ value for each 1 mm increase in effective implant length. The B coefficient was 3.450 in type II bone, 5.660 in type III bone, and 7.380 in type IV. These findings are similar to those of Sennerby and Meredith29 (a decrease of 3 ISQ units per 1 mm in hard bone), although this relationship does not extend to bone types III and IV. This means that in implants placed above the bone level, initial stability was further decreased in bone types III and IV (6-7 ISQ units per 1 mm) and these findings were contrary to the study of Sennerby and Meredith29 This trend in bone types and ISQ values held in the other 2 implants.
In type II bone, the B coefficients of the Brånemark (3.450) and Astra (3.210) implants were smaller than that of Straumann implant (5.160). This may be due to the non-self tapping design of the Straumann implant. In bone types III and IV, the B coefficient of the Astra implant was smaller than that of the other 2 implants. This result supports the efficacy of the smaller pitch design in achieving initial stability in implants placed above bone level in born types III and IV. Unstandardized B coefficients of the implants are summarized in Fig. 4.
ISQ values of the 3 implants were compared to elucidate whether implant design affected initial stability at a certain effective implant length. In the control group, type II bone ISQ values were not significantly different between implant types. In bone types III and IV, however the ISQ value of the Straumann implant was significantly less than that of other implants.
These results suggest that implant design did not affect the initial stability of implants where bone quality was good.
The initial stability of implants placed above the bone level was affected by the implant design and bone quality itself. The initial stability was much affected by the implant design in bone types III, IV and the implant design such as the short pitch interval was beneficial to the initial stability of implants placed above the bone level.
References
1. Zarb GA, Albrektsson T. Osseointegration: A requiem for the periodontal ligament. Int J Periodontic Restorative Dent. 1991; 11:88–91.
2. Friberg B, Jemt T, Lekholm U. Early failures in 4,641 consecutively placed Brånemark dental implants: a study from stage 1 surgery to the connection of completed prostheses. Int J Oral Maxillofac Implants. 1991; 6:142–146. PMID: 1809668.
3. Jaffin RA, Berman CL. The excessive loss of Brånemark fixtures in type IV bone: a 5-year analysis. J Periodontol. 1991; 62:2–4. PMID: 2002427.
4. O'Sullivan D, Sennerby L, Meredith N. Measurements comparing the initial stability of five designs of dental implants: a human cadaver study. Clin Implant Dent Relat Res. 2000; 2:85–92. PMID: 11359268.
5. West JD, Oates TW. Identification of stability changes for immediately placed dental implants. Int J Oral Maxillofac Implants. 2007; 22:623–630. PMID: 17929524.
6. Albrektsson T, Wennerberg A. Oral implant surfaces: Part 2-review focusing on clinical knowledge of different surfaces. Int J Prosthodont. 2004; 17:544–564. PMID: 15543911.
7. Ostman PO, Hellman M, Wendelhag I, Sennerby L. Resonance frequency analysis measurements of implants at placement surgery. Int J Prosthodont. 2006; 19:77–83. discussion 84. PMID: 16479765.
8. Balshi SF, Allen FD, Wolfinger GJ, Balshi TJ. A resonance frequency analysis assessment of maxillary and mandibular immediately loaded implants. Int J Oral Maxillofac Implants. 2005; 20:584–594. PMID: 16161743.
9. Meredith N, Alleyne D, Cawley P. Quantitative determination of the stability of the implant-tissue interface using resonance frequency analysis. Clin Oral Implants Res. 1996; 7:261–267. PMID: 9151590.


10. Osstell™ mentor Resonance Frequency Analyser. User's manual. Sweden: Integration Diagnostics Ltd..
11. Meredith N, Book K, Friberg B, Jemt T, Sennerby L. Resonance frequency measurements of implant stability in vivo. A crosssectional and longitudinal study of resonance frequency measurements on implants in the edentulous and partially dentate maxilla. Clin Oral Implants Res. 1997; 8:226–233. PMID: 9586467.
12. Adell R, Lekholm U, Brånemark PI. Brånemark PI, Zarb GA, Albrektsson T, editors. Surgical procedures. Tissue integrated prostheses. Osseointegration in clinical dentistry. 1985. Chicago: Quintessence;p. 199–210.
13. Misch CE. Contemporary Implant Dentistry. 1993. St Louis, Mo: CV Mosby;p. 327–354.
14. Johansson P, Strid KG. Assessment of bone quality from cutting resistance duringimplant surgery. Int J Oral Maxillofac Implants. 1994; 9:279–288.
15. Friberg B, Sennerby L, Roos J, Johansson P, Strid CG, Lekholm U. Evaluation of bone density using cutting resistance measurements and microradiography: an in vitro study in pig ribs. Clin Oral Implants Res. 1995; 6:164–171. PMID: 7578792.


16. Friberg B, Sennerby L, Roos J, Lekholm U. Identification of bone quality in conjunction with insertion of titanium implants. A pilot study in jaw autopsy specimens. Clin Oral Implants Res. 1995; 6:213–219. PMID: 8603112.


17. Carr AB, Papazoglou E, Larsen PE. The relationship of Periotest values, biomaterial, and torque to failure in adult baboons. Int J Prosthodont. 1995; 8:15–20. PMID: 7710620.
18. Manz M, Morris HF, Ochi S. Dental Implant Clinical Group (Planning Committee). An evaluation of the Periotest system. Part I: Examiner reliability and repeatability of readings. Implant Dent. 1992; 1:142–146. PMID: 1288806.
19. Olivé J, Aparicio C. Periotest method as a measure of osseointegrated oral implant stability. Int J Oral Maxillofac Implants. 1990; 5:390–400. PMID: 2094658.
20. van Steenberghe D, Quirynen M. Reproducibility and detection thresthold of periimplant diagnostics. Adv Dent Res. 1993; 7:191–195. PMID: 8260007.
21. van Steenberghe D, Tricio J, Naert I, Nys M. Damping characteristics of bone-to-implant interfaces. A clinical study with the Periotest device. Clin Oral Implants Res. 1995; 6:31–39. PMID: 7669865.
22. Teerlinck J, Quirynen M, Darius P, van Steenberghe D. Periotest: an objective clinical diagnosis of bone apposition toward implants. Int J Oral Maxillofac Implants. 1991; 6:55–61. PMID: 1843494.
23. Schulte W, d'Hoedt B, Lukas D, Muhlbradt L, Scholz F, Bretschi J, Frey D, Gudat H, Konig M, Markl M. Periotest - a new measurement process for periodontal function. Zahnarztl Mitt. 1983; 73:1229–1240. PMID: 6588690.
24. Derhami K, Wolfaardt JF, Faulkner G, Grace M. Assessment of the periotest device in baseline mobility measurements of craniofacial implants. Int J Oral Maxillofac Implants. 1995; 10:221–229. PMID: 7744442.
25. Niimi A, Ozeki K, Ueda M, Nakayama B. A comparative study of removal torque of endosseous implants in the fibula, iliac crest and scapula of cadavers: preliminary report. Clin Oral Implants Res. 1997; 8:286–289. PMID: 9586475.
26. Sennerby L, Meredith N. Resonance frequency analysis: measuring implant stability and osseointegration. Compend Contin Educ Dent. 1998; 19:493–498. 500502quiz 504. PMID: 9693511.
27. Meredith N, Shagaldi F, Alleyne D, Sennerby L, Cawley P. The application of resonance frequency measurements to study the stability of titanium implants during healing in the rabbit tibia. Clin Oral Implants Res. 1997; 8:234–243. PMID: 9586468.


28. Meredith N. Assessment of implant stability as a prognostic determinant. Int J Prosthodont. 1998; 11:491–501. PMID: 9922740.
29. Sennerby L, Meredith N. Analisi della Frequenza di Risonanza (RFA). Osteointegrazione e carico immediato. 2002. p. 19–32.
30. Zix J, Kessler-Liechti G, Mericske-Stern R. Stability measurements of 1-stage implants in the maxilla by means of resonance frequency analysis: a pilot study. Int J Oral Maxillofac Implants. 2005; 20:747–752. PMID: 16274149.
Fig. 2
Photographs of (A) Bråemark, (B) Straumann, and (C) Astra implants placed in level with the bone or placed 1, 2, 3, and 4 mm above the bone level in type II bone. (D) - (F) In type III bone and (G) - (I) in type IV bone.
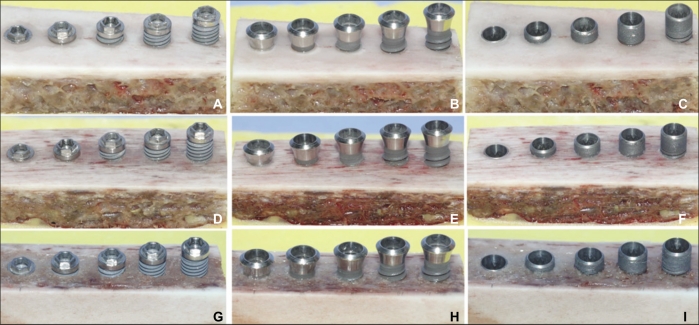
Fig. 4
Unstandardized coefficient B of each implant in different types of bone. This coefficient represents the change in ISQ value per 1 mm increase in effective implant length. T2 = type II bone, T3 = type III bone, and T4 = type IV bone.
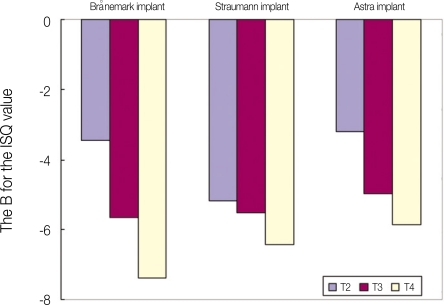