Abstract
BACKGROUND/OBJECTIVES
Physical exercise promotes energy producing pathways requiring thiamin and riboflavin as a coenzyme. Therefore, this study investigated the effects of regular exercise training on urinary excretion of thiamin and riboflavin.
MATERIALS/METHODS
Fifty rats were randomly assigned to one of two groups: non-exercise training (NT, n = 25) and regular exercise training (ET, n = 25) for 5 weeks. The rats performed moderate exercise on a treadmill (0.5-0.8 km/hour) for 30 min/day, 5 days/week. Twenty-four hour urine samples were collected at the end of the 0 week, 3rd week, and 5th week of training and thiamin and riboflavin were analyzed.
RESULTS
No significant differences in thiamin and riboflavin intakes for each week were observed between the NT and ET groups. Urinary thiamin excretion of each group was the highest at the 5th week compared to the levels at 0 and 3rd week. Urinary thiamin at the 5th week was significantly lower in the ET group than in the NT group. Urinary riboflavin excretion was increased by training duration, however, no difference was observed between NT and ET for each week. At 0 and 3rd week, no significant relationships were observed between dietary intake and urinary excretion of thiamin and riboflavin, however, at the 5th week, urinary excretion was significantly increased by dietary intake only in the NT group (P < 0.05). Thiamin excretion of both NT and ET groups was significantly increased with riboflavin excretion at the 5th week (P < 0.01).
CONCLUSION
Regular moderate exercise training increased urinary excretion of thiamin. Dietary intakes and urinary excretions of thiamin and riboflavin showed positive correlation in both the exercise training and non-exercise training groups as the exercise training period went by, while the correlations in the exercise training group were weaker than those in the non-exercise training group. Therefore, regular exercise training can alter the urinary excretion of thiamin and riboflavin in rats.
Potential ergogenic effects of B vitamins have been demonstrated and the practice of vitamin supplementation is widespread among both recreational athletes and competitive athletes. Many dietary supplement advocators have stressed the importance of adequate consumption of B vitamins such as thiamin and riboflavin, due to the involvement of these vitamins in processing carbohydrate and fats for energy production, an important consideration during exercise of varying intensity [1].
Thiamin, as thiamin pyrophosphate (TPP), plays a key role in carbohydrate and protein metabolism. TPP acts as a coenzyme in the conversion of pyruvate to acetyl-CoA and α-ketoglutarate to succinyl-CoA and is also involved in the decarboxylation of branched-chain amino acids, which increases with physical activity [2,3]. Almost half of thiamin in the body is stored in the muscles and thiamin is necessary for normal functioning of the skeletal and cardiac musculature [4,5]. Thus, thiamin may be a potentially limiting nutrient in physical activity. Riboflavin is an essential component of two coenzymes, flavin adenine dinucleotide (FAD) and flavin mononucleotide (FMN), which participate in the transfer of electrons in energy metabolism, amino acid metabolism, and steroid hormone production [2,4,6]. Riboflavin may enhance energy availability during oxidative metabolism [7].
Physical exercise demands a significant amount of energy turnover and the metabolic pathways that produce energy are stressed. Thus, it could increase the total daily needs for thiamin and riboflavin in active individuals. Exercise training also induces adaptations to stress during exercise and plays a key role in homeostasis associated with an increase in aerobic metabolism [8,9,10]. Recently, because regular physical exercise is associated with multiple health benefits related reduced risk for cardiovascular diseases, diabetes, and obesity, regular exercise is promoted in populations. Thus, the body turnover or requirements of micronutrients by regular physical exercise have become an important area of investigation. Direct evidence demonstrating that exercise training affects body turnover or loss of thiamin and riboflavin has been limited. In addition, despite the remarkable role of thiamin and riboflavin in energy turnover during exercise, the interaction between exercise and vitamins is poorly described in the literature. Therefore, the goals of this study were as follows: 1) to determine whether exercise training affects urinary excretion of thiamin and riboflavin and 2) to determine whether there is an association or compensation in urinary excretion between thiamin and riboflavin.
Fifty male Sprague-Dawley rats (Daehanbiolink Co., South Korea) weighing 95-105 g (4-week-old rats) were fed a diet which met the AIN-93 recommendations (American Institute of Nutrition). The AIN-93 diet contained 5.3 mg/kg of thiamin and 6.0 mg/kg of riboflavin, respectively. The study protocol was approved by the Committee on Animal Welfare Regulations of Duksung Women's University, Korea (2011-108). The vitamin intakes (thiamin and riboflavin) of rats were calculated with the amount of feeding and the composition of the diet. Animals were kept individually in stainless-steel cages in a room controlled for temperature (22 ± 1℃), relative humidity (50-60%), and light (12-hour light/dark cycle).
Fifty animals were randomly assigned to one of two groups: regular exercise training (ET, n = 25) or non-exercise training (NT, n = 25) for 5 weeks. For ET, the rats performed moderate exercise on a treadmill (Jungdo Bio & Plant, JD-A-09, Korea, 15° incline, 0.5-0.8 km/hour) for 30 minutes per day, 5 days per week regularly. Rats were forced to exercise using light electric shocks if they did not engage themselves in running on the treadmill [11]. No deaths occurred during or after exercise in the ET group. Twenty-four hour urine samples of all animals were collected over acetic acid at the end of the 0, 3rd, and 5th week of exercise training and stored at -70℃ until analysis.
Urinary vitamin concentrations were measured by HPLC with fluorometric detection for thiamin [12] and riboflavin [13]. The recoveries of added thiamin and riboflavin from urine were 99.4% and 96.7%, respectively. Detection limits of the assays were 0.0012 ng thiamin and 0.0084 ng riboflavin. In this study, all urine samples for each vitamin were extracted in duplicate.
All data were subjected to an analysis of variance (ANOVA), followed by Duncan's multiple range test using SAS version 9.1.3 (SAS Institute, Inc., Cary, North Carolina, US). In addition, differences between the NT and EX groups were determined using t-test. Pearson's correlations coefficients were calculated to determine correlations between intake and urinary excretion of thiamin and riboflavin. Multiple linear regression analysis was performed to determine the association of urinary thiamin and urinary riboflavin excretions by regular exercise training after adjustment for body weight and feed intake. Results were considered statistically significant at P < 0.05.
At 0 week, no significant difference in body weight was observed between the NT and ET groups; however, at the 3rd and 5th weeks, body weights of the NT group were higher than those of the ET group, respectively (Table 1). However, no significant differences in thiamin and riboflavin intake as well as feed intake and feed efficiency ratio were observed at each week.
Urinary thiamin excretion was increased over time in each group, and the highest excretion was observed at the 5th week compared to the levels at 0 and 3rd week (Fig. 1). Thiamin level at the 5th week was significantly lower in the ET group than in the NT group. Although no significant difference of urinary riboflavin excretion was observed between the NT and ET groups at each week, riboflavin excretions were increased by training duration (Fig. 2)
At 0 and 3rd week, no significant relationships were observed between dietary intake and urinary excretion of thiamin (Fig 3). At the 5th week, urinary thiamin excretions were increased by dietary intake in the NT (r = 0.67418, P = 0.0002) and ET groups (r = 0.35442, P = 0.0822). No significant relations between dietary intake and urinary excretion of riboflavin were observed at 0 and 3rd week, however at the 5th week, urinary excretion was significantly increased by dietary intake only in the NT group (r = 0.54408, P = 0.0049) (Fig. 4).
Table 2 shows the relationships of urinary thiamin and riboflavin in rats after adjustment for body weight and feed intake. At 0 week, no relations were observed between thiamin and riboflavin excretions. At the 3rd week, urinary thiamin excretion showed a significant increase with riboflavin excretion only in the NT group. Urinary thiamin excretions were significantly increased by increased urinary riboflavin excretion in the NT and ET groups at the 5th week.
This study showed that moderate exercise training affected the urinary excretion of thiamin and riboflavin and had a positive effect on the utilization of vitamins. The most valuable marker for assessing nutritional status is the measurement of vitamins in tissues and in the circulation; however, an alternative approach, but with obvious practical limitations, is the measurement of urinary excretion of thiamin and riboflavin, which is a simple reflection of an excess of current intake over tissue requirement. This approach has provided useful information regarding tissue saturation [14,15,16] under the circumstance of optimum nutritional status for thiamin and riboflavin. Thus, urinary vitamin excretion has been used as a biomarker for evaluation of vitamin status [17,18,19]. Urinary losses decrease when vitamin stores decrease.
Woolf and Manore [3] reviewed the possibilities that regular physical activity may alter the requirements for some micronutrients. First, the metabolic pathways that produce energy are stressed during physical exercise, thus requirements for some of the nutrients used in these pathways may increase. Second, biochemical adaptations that occur with training in the tissues of the body may increase requirements. Third, exercise may also increase the turnover or loss of a particular micronutrient in sweat, urine, or feces. Finally additional micronutrients may be required for repair and maintenance of the higher lean tissue mass of some active individuals. In addition, regular physical exercise increases oxygen availability to the skeletal muscle due to myoglobin formation, increased muscle capillarization, increase in the size and number of mitochondria, and increase in aerobic enzyme levels and activity.
In this study, the level of urinary excretion of thiamin was increased with passage of time in both the non-exercise training and exercise training group, but the extent of increase with time was less in the exercise training group compared to the non-exercise training group. These results suggest that biochemical adaptations that occur with exercise training in the tissues of the body might increase requirements for thiamin and excrete less thiamin in urine. It is not known whether thiamin coenzymes are catabolized and excreted or whether they are recycled after metabolic utilization. Exercise training has not been shown to alter the level of erythrocyte transketolase activity coefficient, a biochemical measure for thiamin status, in active individuals [20], however, endurance training subjects showed significantly lower plasma thiamin levels before and after exercise and decreased lactate levels during exercise [21]. Thiamin requirement is related to energy consumption particularly that derived from carbohydrate. In animal models, chronic exercise has been attributed a key role in tissue homeostasis, associated with increased aerobic metabolism. In previous reports, moderate exercise training resulted in animal's adapting to storage of more glycogen and reduced glycogen depletion for one hour and facilitating the mobilization and oxidation of fat and delayed onset of fatigue associated with low lactate levels [11,22,23] and thiamin derivative decreased oxidation of exogenous glucose at rest, but not during exercise [24].
Although no relations were observed between dietary intake and urinary excretion of thiamin at 0 week and 3rd week, positive associations of intake and urinary excretion at the 5th week were observed in the non-exercise training and exercise training groups. However, compared to the non-exercise training group, exercise training groups showed lower associations at the 5th week. Since the urinary vitamin levels reflect the previous several days of intake rather than one day of intake [25], the lowered associations between urinary excretion and dietary intake at the 5th week in the exercise training group might be the consequence of the exercise training. Regular exercise training increases muscle mass and decreases body fat deposition; thus, body composition may be different by exercise training despite no significant differences in total body weight [26,27]. More than 40% of the total body thiamin is stored in muscle tissue. In this study, more thiamin might be required for muscle tissue saturation as muscle tissue was increased in the exercise training group compared to the non-exercise training group. Also, as mentioned above, the consequence of reduced glycogen depletion with lower lactate level by exercise might induce the lower increase in urinary excretion of thiamin in the exercise training group.
Riboflavin may play an important role in exercise-induced biochemical adaptions [28,29]. Regular exercise promotes riboflavin accumulation [27] and FAD-dependent enzymes such as succinate dehydrogenase in muscle tissue [28]. Some studies have demonstrated a moderate rise in erythrocyte glutathione reductase activity coefficient, a biochemical measure for riboflavin status, as well as a decrease in urinary riboflavin excretion with an increase in physical activity [6,30,31]. In this study, no significant difference in dietary riboflavin intakes was observed between non-exercise training and exercise training groups. Thus, theoretically, urinary riboflavin excretion of the exercise training group should be affected by exercise training. However, in the current study, although the level of urinary excretion of riboflavin was increased with passage of time in both the non-exercise training and exercise training groups, the extent of increase in the exercise training group did not differ significantly from that of the non-exercise training group throughout the study. Both the non-exercise training group and exercise training group showed no associations until the 5th week and weak associations were determined between urinary excretion and dietary intake. This finding is consistent with the previous report, which showed that blood thiamin concentration decreased significantly, but riboflavin concentration was unchanged during intensive training in college swimmers [32]. In rats, exercise training during a period of 8 weeks had no effect on riboflavin-dependent parameters including urinary riboflavin excretion and erythrocyte glutathione reductase activity coefficients [27]. Thus, riboflavin status might not be altered by regular exercise training. However, exercise training might improve utilization of riboflavin in the body by decreased turnover of flavoproteins, decreased nonfunctional metabolism of riboflavin, and decreased urinary excretion, which should be determined in future research. In addition, AIN-93 diet contains 6.0 mg/kg of riboflavin. Hunter and Turkki [27] reported a riboflavin tissue saturation in both sedentary and exercised rats fed a marginal riboflavin diet (2.0 to 2.5 mg/kg diet) and no significant difference in the tissue riboflavin concentration was observed between two groups. Thus, dietary requirement of riboflavin in rats may be much lower than that of the AIN-93 diet. In the current study, due to a larger amount of riboflavin intake than the requirement of rats, there was no significant difference in urinary excretion between non-exercise training and exercise training groups. Also, an increased requirement by exercise training might promote absorption of riboflavin in the small intestine. More research with regard to riboflavin requirement and absorption of rats is needed.
Although the current study did not examine any biochemical events related to exercise training, some biochemical deduction may be derived from the relationships among urinary excretion of vitamins. In this study, urinary excretion of thiamin showed significant positive association with that of riboflavin at the 3rd and 5th week in the non-exercise training group, and in the exercise training group, a significant positive relationship was determined only at the 5th week, after adjustment for body weight and feed intake. There might be some effects of compensation with thiamin and riboflavin on metabolic functions. The effects might be affected by exercise training. Therefore, more research is needed regarding the compensation effects and relations of thiamin and riboflavin in metabolism related physiological adaptations of exercise training.
In conclusion, regular moderate exercise training increased urinary excretion of thiamin. Dietary intakes and urinary excretions of thiamin and riboflavin showed positive correlation in both exercise training and non-exercise training groups as the exercise training period went by, while the correlations in the exercise training group were weaker than those in the non-exercise training group. Therefore, regular exercise training can alter the urinary excretion of thiamin and riboflavin in rats. However, no significant differences in urinary excretion of thiamin and riboflavin were observed between exercise training and non-exercise training groups. To determine change of thiamin and riboflavin requirements by regular exercise and necessity for thiamin and riboflavin supplementation during exercise training, further research is required. In addition, future research is needed in order to investigate change of riboflavin utilization by exercise training and association of thiamin and riboflavin in metabolism for body adaptations of exercise training.
Figures and Tables
Fig. 1
The effect of regular exercise training on the urinary excretion of thiamin. abcA different superscript letter indicates significant difference at P < 0.05 in each group by a duration of regular exercise training. * Urinary thiamin levels were significant in the non-exercise training and exercise training groups at P < 0.05.
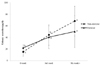
Fig. 2
The effect of regular exercise training on the urinary excretion of riboflavin. abcA different superscript letter indicates significant difference at P < 0.05 in each group by duration of regular exercise training. There were no significant differences of urine riboflavin between groups at 0, 3rd, and 5th week.
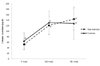
Fig. 3
The effect of regular exercise training on the relations between the intake and urinary excretion of thiamin
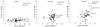
Fig. 4
The effect of regular exercise training on the relations between the intake and urinary excretion of riboflavin
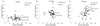
References
1. Williams MH. Dietary supplements and sports performance: introduction and vitamins. J Int Soc Sports Nutr. 2004; 1:1–6.


2. McCormick DB. Coenzymes, biochemistry. In : Dulbecco R, editor. Encyclopedia of Human Biology. 2nd ed. San Diego (CA): Academic Press;1997. p. 847–864.
3. Woolf K, Manore MM. B-vitamins and exercise: does exercise alter requirements? Int J Sport Nutr Exerc Metab. 2006; 16:453–484.


4. Johnson Gubler C. Thiamin. In : Machlin LJ, editor. Handbook of Vitamins: Nutritional, Biochemical, and Clinical Aspects. New York (NY): Marcel Dekker;1984. p. 245–295.
5. Al-Tamimi EK, Haub MD. Thiamin. In : Driskell JA, Wolinsky I, editors. Sports Nutrition: Vitamins and Trace Elements. 2nd ed. Boca Raton (FL): CRC Press;2006. p. 47–60.
6. Manore MM. Effect of physical activity on thiamine, riboflavin, and vitamin B-6 requirements. Am J Clin Nutr. 2000; 72:598S–606S.


7. Kreider RB, Wilborn CD, Taylor L, Campbell B, Almada AL, Collins R, Cooke M, Earnest CP, Greenwood M, Kalman DS, Kerksick CM, Kleiner SM, Leutholtz B, Lopez H, Lowery LM, Mendel R, Smith A, Spano M, Wildman R, Willoughby DS, Ziegenfuss TN, Antonio J. ISSN exercise & sport nutrition review: research & recommendations. J Int Soc Sports Nutr. 2010; 7:7.
8. Ravi Kiran T, Subramanyam MV, Asha Devi S. Swim exercise training and adaptations in the antioxidant defense system of myocardium of old rats: relationship to swim intensity and duration. Comp Biochem Physiol B Biochem Mol Biol. 2004; 137:187–196.


9. Lira FS, Koyama CH, Yamashita AS, Rosa JC, Zanchi NE, Batista ML Jr, Seelaender MC. Chronic exercise decreases cytokine production in healthy rat skeletal muscle. Cell Biochem Funct. 2009; 27:458–461.


10. Campbell PT, Gross MD, Potter JD, Schmitz KH, Duggan C, McTiernan A, Ulrich CM. Effect of exercise on oxidative stress: a 12-month randomized, controlled trial. Med Sci Sports Exerc. 2010; 42:1448–1453.
11. Choi EY, Cho YO. The influence of different durations of aerobic exercise on fuel utilization, lactate level and antioxidant defense system in trained rats. Nutr Res Pract. 2014; 8:27–32.


12. Losa R, Sierra MI, Fernández A, Blanco D, Buesa JM. Determination of thiamine and its phosphorylated forms in human plasma, erythrocytes and urine by HPLC and fluorescence detection: a preliminary study on cancer patients. J Pharm Biomed Anal. 2005; 37:1025–1029.


13. Gatautis VJ, Naito HK. Liquid-chromatographic determination of urinary riboflavin. Clin Chem. 1981; 27:1672–1675.


14. Zempleni J, Galloway JR, McCormick DB. Pharmacokinetics of orally and intravenously administered riboflavin in healthy humans. Am J Clin Nutr. 1996; 63:54–66.


15. Horwitt MK. Interpretations of requirements for thiamin, riboflavin, niacin-tryptophan, and vitamin E plus comments on balance studies and vitamin B-6. Am J Clin Nutr. 1986; 44:973–985.


16. Powers HJ. Current knowledge concerning optimum nutritional status of riboflavin, niacin and pyridoxine. Proc Nutr Soc. 1999; 58:435–440.


17. Tasevska N, Runswick SA, McTaggart A, Bingham SA. Twenty-four-hour urinary thiamine as a biomarker for the assessment of thiamine intake. Eur J Clin Nutr. 2008; 62:1139–1147.


18. Fukuwatari T, Shibata K. Urinary water-soluble vitamins and their metabolite contents as nutritional markers for evaluating vitamin intakes in young Japanese women. J Nutr Sci Vitaminol (Tokyo). 2008; 54:223–229.


19. Tsuji T, Fukuwatari T, Sasaki S, Shibata K. Urinary excretion of vitamin B1, B2, B6, niacin, pantothenic acid, folate, and vitamin C correlates with dietary intakes of free-living elderly, female Japanese. Nutr Res. 2010; 30:171–178.


20. Fogelholm M. Micronutrient status in females during a 24-week fitness-type exercise program. Ann Nutr Metab. 1992; 36:209–218.


21. Choi SK, Baek SH, Choi SW. The effects of endurance training and thiamine supplementation on anti-fatigue during exercise. J Exerc Nutrition Biochem. 2013; 17:189–198.


22. Choi EY, Cho YO. Moderate physical training can increase muscle glycogen levels but does not alter protein levels with exercise in rats. Nutr Sci. 2006; 9:112–116.
23. Choi EY, Cho YO. The effects of physical training on antioxidative status under exercise-induced oxidative stress. Nutr Res Pract. 2007; 1:14–18.


24. Masuda H, Matsumae H, Masuda T, Hatta H. A thiamin derivative inhibits oxidation of exogenous glucose at rest, but not during exercise. J Nutr Sci Vitaminol (Tokyo). 2010; 56:9–12.


25. Shibata K, Fukuwatari T, Watanabe T, Nishimuta M. Intra- and inter-individual variations of blood and urinary water-soluble vitamins in Japanese young adults consuming a semi-purified diet for 7 days. J Nutr Sci Vitaminol (Tokyo). 2009; 55:459–470.


26. Vallerand AL, Cuerrier JP, Shapcott D, Vallerand RJ, Gardiner PF. Influence of exercise training on tissue chromium concentrations in the rat. Am J Clin Nutr. 1984; 39:402–409.


27. Hunter KE, Turkki PR. Effect of exercise on riboflavin status of rats. J Nutr. 1987; 117:298–304.


28. Gollnick PD, Armstrong RB, Saltin B, Saubert CW 4th, Sembrowich WL, Shepherd RE. Effect of training on enzyme activity and fiber composition of human skeletal muscle. J Appl Physiol. 1973; 34:107–111.


29. Molé PA, Oscai LB, Holloszy JO. Adaptation of muscle to exercise. Increase in levels of palmityl CoA synthetase, carnitine palmityltransferase, and palmityl CoA dehydrogenase, and in the capacity to oxidize fatty acids. J Clin Invest. 1971; 50:2323–2330.
30. Belko AZ, Obarzanek E, Roach R, Rotter M, Urban G, Weinberg S, Roe DA. Effects of aerobic exercise and weight loss on riboflavin requirements of moderately obese, marginally deficient young women. Am J Clin Nutr. 1984; 40:553–561.

