Abstract
BACKGROUND/OBJECTIVES
The purpose of this study was to examine the effects and associated mechanisms of arctiin, a lignan compound found in burdock, on adipogenesis in 3T3-L1 cells. Also, the effects of arctiin supplementation in obese mice fed a high-fat diet on adiposity were examined.
MATERIALS/METHODS
3T3-L1 cells were treated with arctiin (12.5 to 100 µM) during differentiation for 8 days. The accumulation of lipid droplets was determined by Oil Red O staining and intracellular triglyceride contents. The expressions of genes related to adipogenesis were measured by real-time RT-PCR and Western blot analyses. For in vivo study, C57BL/6J mice were first fed either a control diet (CON) or high-fat diet (HF) to induce obesity, and then fed CON, HF, or HF with 500 mg/kg BW arctiin (HF + AC) for four weeks.
RESULTS
Arctiin treatment to 3T3-L1 pre-adipocytes markedly decreased adipogenesis in a dose-dependent manner. The arctiin treatment significantly decreased the protein levels of the key adipogenic regulators PPARγ and C/EBPα, and also significantly inhibited the expression of SREBP-1c, fatty acid synthase, fatty acid-binding protein and lipoprotein lipase. Also, arctiin greatly increased the phosphorylation of AMP-activated protein kinase (AMPK) and its downstream target phosphorylated-acetyl CoA carboxylase. Furthermore, administration of arctiin significantly decreased the body weight in obese mice fed with the high-fat diet. The epididymal, perirenal or total visceral adipose tissue weights of mice were all significantly lower in the HF + AC than in the HF. Arctiin administration also decreased the sizes of lipid droplets in the epididymal adipose tissue.
Obesity is one of the major public health problems. The prevalence of obesity has dramatically increased worldwide, and over 200 million men and nearly 300 million women aged 20 and older are obese [1]. Obesity is characterized by characterized by an excess in the number or size of adipocytes. As the normal functions of adipocytes are crucial in maintaining energy and metabolic homeostasis, excess adipocytes often result in dysregulated secretion of adipocytokines and systemic insulin insensitivity, as well as perturbation in energy metabolism [2]. Consequently, obesity is closely associated with increased risks for various metabolic diseases including type 2 diabetes, cardiovascular disease, hypertension, musculoskeletal disorders and some cancers [3,4,5,6].
Adipogenesis involves the differentiation of pre-adipocytes into mature adipocytes and plays a key role in the expansion of adipose tissue mass and subsequent obesity. Adipogenesis is controlled by a coordinated gene expression, which is mediated by a number of transcription factors. In particular, proliferator-activated receptor gamma (PPARγ) and CCAAT/enhancer-binding protein alpha (C/EBPα) are considered as the two primary transcription factors that mediate adipogenesis [7]. PPARγ has been shown to be necessary for adipogenesis as evidenced by the observations that the deletion of PPARγ in mice resulted in placental dysfunction and embryonic lethality [8] and transgenic mice lacking PPARγ specifically in adipose tissue exhibited greatly reduced sized fat pads [9]. Similarly, transgenic mice lacking C/EBPα had defective adipogenesis [10] and ectopic expression of C/EBPα was sufficient to initiate adipogenesis [11]. Both PPARγ and C/EBPα are greatly induced during adipogenesis, and they are necessary for the expression of many adipogenic genes such as fatty acid synthase (FAS), adipocyte fatty acid-binding protein (aP2) [12,13,14], and lipoprotein lipase (LPL) [15]. Therefore, the dietary or natural compounds that suppress PPARγ and C/EBPα and the adipogenic process would have significant effects on the prevention and treatment of obesity.
Arctiin is a major lignan constituent of burdock (Arctium lappa L.). Also known as Woo-ung in Korean, burdock is commonly used in many foods as well as in traditional medicine. Many studies have shown that arctiin has a variety of biological activities such as anti-viral [16], anti-proliferative [17], and anti-inflammatory [18,19] activities; however, its anti-adipogenic effect has not yet been investigated. Therefore, in the present study, we investigated the effect of arctiin on adipogenesis and related molecular mechanisms using 3T3-L1 pre-adipocytes. Further, we examined the effects of arctiin supplementation on body weight and adiposity in obese mice fed a high-fat diet.
Reflux extraction of the Arctium lappa L. seeds (5.4 kg) was done by applying 5 L of n-hexane followed by 50 L of 80% ethanol. The 80% ethanol extract was evaporated to dryness, yielding 533 g of dry powder. The 80% ethanol extract was suspended in distilled water (1 L) and further extracted with 5 L of ethyl acetate. The ethyl acetate extract was then applied to a column of silica gel column chromatography (7 × 40 cm) and eluted with chloroform: methanol (10:1) to yield five sub-fractions. Among these, arctiin was obtained from fraction III (9.62 g) by recrystallization with methanol, which were verified by using the 1H-NMR and 14C-NMR data [20].
3T3-L1 fibroblast cell lines (Korea cell line bank, Seoul, Korea) were maintained in Dulbecco's modified eagle's medium (DMEM)/high glucose (Hyclone, UT, USA) with 10% newborn calf serum (Gibco™, Life Technologies, NY, USA), 100 units/ml penicillin, and 100 µg/ml streptomycin at 37℃ in 5% CO2 incubator. To induce differentiation, 100% confluent 3T3-L1 pre-adipocytes (day 0) were stimulated for 2 days with MDI media [0.5 mM 3-isobutyl-1-methylxanthine (Sigma Aldrich, MO, USA), 1 µM dexamethasone (Sigma Aldrich), and 5 µg/ml insulin (Gibco™) in DMEM/10% fetal bovine serum (FBS, Hyclone)] [21]. On day 3, the MDI media was replaced with differentiation media (5 µg/ml insulin in DMEM/10% FBS). On day 6, the differentiation media was replaced with growth media (DMEM/10% FBS). The cell culture media was changed every 2 day.
The 3T3-L1 pre-adipocytes were seeded in 24 well plates at a density of 2 × 104 cells/ml/well. Various concentrations of arctiin were added to the confluent 3T3-L1 pre-adipocytes during the differentiation period. At the end of the treatment, the culture medium was removed and replaced with 50 µl of 5 mg/ml sterile-filtered 3-(4,5-dimethylthiazol-2-thiazolyl)-2,5 diphenyl-2H-tetrazolium bromide (MTT, Sigma Aldrich) solution. Then, cells were incubated for 90 min at 37℃ and dissolved with 500 µl dimethyl sulfoxide (DMSO, Sigma Aldrich). The absorbance of each sample was measured at 540 nm.
Cells were first washed with phosphate-buffered saline, fixed with 10% formalin for 1 hour and washed with distilled water. Cells were then stained with 0.6% Oil Red O solution for 30 min at room temperature, washed 3 times with distilled water, and photographed. For quantitative analyses, stained Oil Red O was eluted with 100% isopropanol and quantified by measuring absorbance at 520 nm.
At the end of the treatment, 3T3-L1 mature adipocytes were collected in 200 µl of PBS-10 mM EDTA (pH 7.4) and sonicated. Total lipids were extracted with the mixture of 2 ml isopropanol: hexane (4:1), 0.5 ml of hexane: diethyl ether (1:1), and 1 ml of distilled water. The organic phase was collected, dried under N2 gas, and dissolved in isopropanol. Triglyceride contents were enzymatically determined by using a commercial kit according to the manufacturer's instructions (Bio-Clinical System, Gyeonggi-do, Korea).
Total RNA was extracted using Trizol® Reagent (Life Technologies) according to the manufacturer's instructions. cDNA was generated using the PrimeScript™ RT reagent kit (Takara, Otsu, Japan). The sequences of forward and reverse primers are listed in Table 1. All PCRs were carried out using SYBR® Premix Ex Taq™ II (Takara) and Mini Opticon instrument (BioRad, Hercules, CA, USA). The real-time cycling conditions were as follows: initial enzyme activation at 50℃ for 2 min and denaturation at 95℃ for 10 min followed by 40 cycles of denaturation at 95℃ for 15 sec and annealing/extension at 60℃ for 1 min. The product purity was confirmed by a dissociation curve analysis. The mRNA levels of the target genes were normalized to the values of β-actin, and presented as fold changes relative to controls (no arctiin treatment).
3T3-L1 cells were collected and suspended in lysis buffer containing 25 mM Tris-HCl (pH 7.6), 1% NP-40, 1% sodium deoxycholate, 150 mM NaCl, 1% SDS, 1 mM phenylmethanesulfonylfluoride, protease inhibitor tablet (Roche, IN, USA) and phosphatase inhibitor tablet (Roche). Total protein concentrations in lysates were measured by using BCA protein assay kit (Pierce, IL, USA). 20 µg protein homogenates were mixed with 2 × loading buffer [25 mM Tris-HCl (pH 6.8), 1% SDS, 30% glycerol, 10% 2-mercaptoethanol (Sigma Aldrich), protease inhibitor tablet (Roche) and phosphatase inhibitor tablet (Roche), 0.7% bromophenol blue (Sigma Aldrich)], heated at 95℃ for 5 min, and separated on 10% SDS polyacrylamide gel. Proteins were then transferred onto PVDF membrane (Millipore, Billerica, MA, USA). After blocking for 2 hours at room temperature with 5% non-fat skim milk (DIFCO, Paris, France) in TBS-T [Tris-buffered saline containing 0.01% Tween 20 (Sigma Aldrich)], the membranes were incubated overnight at 4℃ with the following primary antibodies: PPARγ, C/EBPα, phospho-AMPK, AMPK, phospho-acetyl CoA carboxylase (p-ACC) (Cell Signaling, Boston, MA, USA) and β-actin (Santa Cruz Biotechnology, CA, USA). After washing 5 times with TBS-T, the membranes were further reacted with goat anti-rabbit IgG (H + L)-HRP conjugate (BioRad) or goat anti-mouse IgG (H + L)-HRP conjugate (BioRad) at room temperature for 90 min. Bands were visualized by enhanced chemiluminescence (Clarity™ western ECL substrate, BioRad) and chemiluminescence imager instrument (CLINX Science Instrument, China). For quantification, the densities of each band were determined by a gel analysis software (CLINX Science Instrument).
Male C57BL/6J mice (SLC Inc., Hamamatsu, Japan) were housed in a room with controlled temperature (21~23℃), humidity (55~60%) and lighting (12-h light/dark cycle). After acclimation for 1 week, animals were divided, by weight-matching, into three groups (HF, HF + AC, and CON). HF and HF + AC groups were first fed a high-fat diet (60% kcal from fat) (Research Diets, New Brunswick, NJ, USA) for eleven weeks to induce obesity [22], then HF or HF + AC group were continued to be fed a high-fat diet with 0 or 500 mg/kg body weight (BW) arctiin for four weeks. CON group was fed a control diet (10% kcal from fat) (Research Diets) for the entire study period. Arctiin or vehicle (distilled water) was given five times weekly via oral gavage. At the end of the experimental period, the mice were terminally exsanguinated under isoflurane anesthesia (Aerrane, Fort Dodge Animal Health, Fort Dodge, IA, USA). All animal protocols were approved by the Institutional Animal Care and Use Committee at Kyung Hee University (KHUASP (SE)-12-049).
Epididymal adipose tissues were collected and portions of each tissue were fixed in 10% buffered formalin for further embedding in paraffin wax. The formalin-fixed and paraffin-embedded tissue blocks were further processed by a routine procedure for hematoxylin and eosin (H&E) staining. The sections were photographed under 100 × magnification and examined by investigators blinded to the treatment groups.
To investigate the effects of arctiin on adipocyte differentiation, 3T3-L1 cells were induced to differentiate into adipocytes for 8 days in the presence of various concentrations of arctiin (0-100 µM). Oil red O staining showed that the number of lipid droplets in the differentiated cells was significantly increased as compared with that in the undifferentiated cells (Fig. 1A). Arctiin clearly decreased lipid accumulation in a dose-dependent manner (Fig. 1A and 1B). In addition, arctiin at a dose of 25, 50, and 100 µM markedly decreased the intracellular TG levels by 24.8%, 63.8%, and 73.4%, respectively (Fig. 1C). The treatment with arctiin at concentrations of 12.5 to 100 µM for 8 days did not significantly affect the viability of 3T3-L1 cells, as evaluated by an MTT assay (Fig. 2).
To determine whether arctiin inhibits adipocyte differentiation through down-regulating adipogenic transcription factors, we measured the protein levels of C/EBPα and PPARγ by Western blot analyses. As shown in Fig. 3, arctiin treatment greatly decreased the protein levels of C/EBPα and PPARγ in a dose-dependent manner.
We further examined the effects of arctiin on the mRNA levels of C/EBPα and PPARγ and their target genes such as aP2, FAS, LPL and SREBP-1c by quantitative RT-PCR. Consistent with changes in the protein levels, arctiin treatment at doses of 25, 50 and 100 µM significantly suppressed the induction of C/EBPα and PPARγ gene expression as compared with those in untreated controls. The arctiin treatment also significantly decreased the mRNA levels of aP2, FAS, LPL and SREBP-1c in a dose-dependent manner (Fig. 4).
To examine whether arctiin affects the activity of AMPK, the levels of phosphorylated-AMPK were determined by Western blot analyses. The basal level of phosphorylated-AMPK in untreated 3T3-L1 adipocytes was low. On the other hand, arctiin treatment significantly increased the levels of phosphorylated-AMPK but did not change the levels of AMPK. Arctiin treatment also significantly increased the levels of phosphorylated-ACC, which is the downstream target of AMPK (Fig. 5).
We further investigated the effects of arctiin on body weight and adiposity in vivo using a high-fat diet fed mice model. The final body weights of mice fed a high-fat diet (HF) were significantly higher than those of mice fed the control diet (CON), indicating that the high-fat diet induced obesity. The mice fed the high-fat diet with arctiin (HF + AC) showed significantly lower body weight, as compared to mice in the HF group (Table 2). In addition, the weights of epididymal adipose tissue in the HF + AC group were significantly lower by 26%, compared to the HF group (P < 0.05). The weights of perirenal and total visceral adipose tissue in the HF + AC group were also significantly lowered, as compared to the HF group (P < 0.05). Arctiin administration did not significantly change the daily food intake during the experimental period.
Histological analysis of epididymal adipose tissue confirmed that adipocyte size was markedly enlarged in the HF group compared to the CON group; whereas the adipocyte size was much smaller in the HF + AC group, as compared to the HF group (Fig. 6).
Adipogenesis and increased lipid accumulation are key features in obesity. In the present study, we demonstrated that arctiin, a lignan compound found in burdock (Woo-ung in Korean), significantly inhibited adipogenesis in 3T3-L1 cells and greatly decreased the body weight and the amount of adipose tissue in mice fed a high-fat diet. Previous studies have shown that arctiin and its aglycon arctigenin have a variety of biological activities including anti-tumor, anti-mutagenic, and anti-inflammatory actions [23,24]. However, this is the first report to show that arctiin inhibited adipogenesis in 3T3-L1 cells.
In this study, we first evaluated the anti-obesity effect of arctiin using 3T3-L1 cells. The 3T3-L1 cell line is one of the most well-characterized and reliable models of studying adipogenesis [25]. Adipogenesisis composed of two major phases - adipocyte determination and terminal differentiation, a process during which fibroblast-like pre-adipocytes developed into mature lipid-loaded, insulin-responsive adipocytes [26]. It has been well documented that some natural compounds such as epigallocatechin gallates, resveratrol, and curcumin inhibit adipogenesis [27]. We found that arctiin decreased lipid accumulation, as measured by Oil Red O staining, and reduced triglyceride levels in the cytoplasm of treated cells in a dose-dependent manner. Furthermore, arctiin significantly down-regulated both the mRNA and protein levels of PPARγ and C/EBPα. PPARγ and C/EBPα have been suggested as master regulators of adipogenesis [7,14], and the induction of these transcription factors was shown to increase adipogenic gene expression such as FAS and aP2 by 10 to 100 fold. In our study, when adipogenesis was stimulated in 3T3-L1 pre-adipocytes by treatment with a mixture of isobutylmethylxanthine, dexamethasone, and insulin (MDI), the expression of PPARγ and C/EBPα was highly induced, indicating an essential role for these transcription factors in the regulation of adipogenesis. However, when 3T3-L1 pre-adipocytes were treated with MDI in the presence of various concentrations of arctiin, the expression of PPARγ and C/EBPα was dose-dependently down-regulated. Consistent with the suppression of PPARγ and C/EBPα expression by arctiin, the expressions of FAS, aP2 and LPL were all significantly decreased by arctiin in MDI-treated 3T3-L1 cells. These results demonstrate that arctiin inhibits adipogenesis through the down-regulation of adipogenic transcriptional factors and their target genes.
We also showed that SREBP-1c gene expression was significantly decreased after arctiin treatment during adipocyte differentiation. SREBP-1c is a predominant SREBP-1 isoform in adipose tissue and has been shown to have significant roles in adipogenesis. For example, ectopic expression of a dominant-negative SREBP-1c was shown to attenuate adipocyte differentiation [28]. In addition, overexpression of SREBP-1c enhanced the adipogenic activity of PPARγ [29]. Thus, it is possible that the reduction of SREBP-1c by arctiin could also contribute to the suppression of adipogenesis observed in our study.
To further elucidate the molecular mechanism underlying arctiin-mediated suppression of adipogenesis, we examined the activation of AMPK. AMPK plays a major role in the maintenance of energy homeostasis, and the activation of AMPK in the adipose tissue can induce changes in adiposity which may be implicated in the prevention of obesity [30]. AMPK is involved in the various aspects of metabolism in the adipose tissue including glucose uptake, fatty acid β-oxidation, lipolysis, and adipokine secretion [31]. Moreover, previous studies have reported that the activation of AMPK is associated with the inhibition of adipogenesis [32]. For example, treatment of 3T3-L1 cells with AICAR (5-aminoimidazole-4-carboxamide-1-β-D-ribofuranoside), an analog of AMP, completely inhibited the adipogenesis and lipid accumulation in these cells [33]. In the present study, we demonstrated that arctiin significantly increased the protein levels of phosphorylated-AMPK, the active form of AMPK, suggesting arctiin can act as a potent activator for the AMPK. Further, the activation of AMPK by arctiin was accompanied by a significant increase in the phosphorylation of ACC, one of the major downstream targets of AMPK. ACC catalyzes ATP-dependent carboxylation of acetyl CoA to produce malonyl CoA, which is a rate-limiting step in de novo fatty acid synthesis. Since the phosphorylation of ACC inhibits the enzyme's activity, increased levels of phosphorylated-ACC by arctiin would lead to a decrease in fatty acid biosynthesis. Similar to our results, a recent study has shown that AMPK activation with resveratrol-derived small molecules resulted in a significant inhibition of adipogenesis [34]. Taken together, our findings suggest that arctiin is a potent inhibitor of adipogenesis, whose molecular mechanism involves the AMPK signaling pathways.
Consistent with our in vitro results, the administration of arctiin to mice fed HF diet significantly decreased the final body weights and visceral adipose tissue weights (Table 2). Furthermore, the arctiin administration markedly decreased the size of adipocytes (Fig. 6). There was no difference in daily food intake among the groups. Supporting our data, a previous study by Kuo et al. [35] have reported that burdock has a capacity to lower body weights in rats. However, the Kuo's study [35] did not examine the changes in adipose tissue nor identify the active component of burdock that is responsible for the observed weight reduction. The findings of our study indicate that the arctiin found in burdock has a beneficial effect on body weight management in high-fat diet induced obesity.
In the present study, however, molecular markers associated with decreased adiposity in obese mice have not been examined. As adipogenesis plays a key role in obesity, the marked inhibition of adipogenesis by arctiin treatment in 3T3-L1 adipocytes provides a significant clue as to the potential mechanisms by which arctiin supplementation decreased adiposity and body weight gain in obese mice induced by high-fat diet. We have an ongoing study with a similar approach and will address the question.
In conclusion, we demonstrated that arctiin exerted antiadipogeneic effects through the inhibition of PPARγ and C/EBPα and the activation of AMPK signaling pathways in 3T3-L1 adipocytes, and that arctiin decreased body weight and adiposity in high-fat diet induced obese mice. Our findings warrants further study to develop arctiin as a natural and effective agent for the prevention or treatment of obesity.
Figures and Tables
Fig. 1
Effects of arctiin on the differentiation and adipogenesis of 3T3-L1 cells. 3T3-L1 pre-adipocytes were incubated with MDI (DMEM with 3-isobutyl-1-methylxanthine, dexamethasone, and insulin) for 2 days and then replaced with DMEM containing insulin with or without arctiin (0, 12.5, 25, 50, and 100 µM) for 8 days. (A) Intracellular lipid droplets were stained with Oil Red O and observed at magnification 200 ×. (B) Intensities of Oil Red O staining measured by spectrophotometric analysis at 520 nm. (C) Intracellular triglyceride concentrations. Data are presented as the mean ± SE from three independent experiments. Different letters indicate significant difference (P < 0.05).
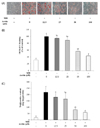
Fig. 2
Effects of arctiin treatment on cell viability in 3T3-L1 cells. 3T3-L1 pre-adipocytes were incubated with MDI (DMEM with 3-isobutyl-1-methylxanthine, dexamethasone, and insulin) for 2 days and then replaced with DMEM containing insulin with or without arctiin (0, 12.5, 25, 50, and 100 µM) for 8 days. Cell viability was determined by MTT assay. Data are presented as the mean ± SE from three independent experiments. Different letters indicate significant difference (P < 0.05).
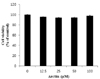
Fig. 3
Effects of arctiin on the protein levels of adipogenic transcription factors in 3T3-L1 cells. The protein levels of PPARγ and C/EBPα in 3T3-L1 cells were determined by Western blot analyses. (A) Representative Western blot. (B) Densitometric analyses. Data are presented as the mean ± SE from three independent experiments. Different letters indicate significant difference (P < 0.05).
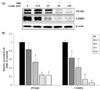
Fig. 4
Effects of arctiin on the expression of adipogenic genes in 3T3-L1 cells. The mRNA levels of adipogenic genes were examined by real-time RT-PCR. Data are presented as the mean ± SE from three independent experiments. Different letters indicate significant difference (P < 0.05).
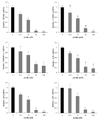
Fig. 5
Effects of arctiin on AMPK phosphorylation in 3T3-L1 cells. The phosphorylation of AMPK and ACC in 3T3-L1 cells were determined by Western blot analyses. (A) Representative Western blot. Densitometric analyses for AMPK phosphorylation (B) and ACC phosphorylation (C) Data are presented as the mean ± SE from three independent experiments. Different letters indicate significant difference (P < 0.05).
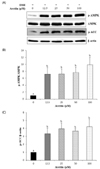
Fig. 6
Effects of arctiin on the cell sizes of the epididymal adipose tissues in mice fed a high-fat diet. Hematoxylin and eosin-stained sections of epididymal adipose tissues are shown at 100 × magnification.
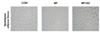
References
1. World Health Organization. Obesity and overweight [Internet]. [place unknown]: World Health Organization;2014. cited 2014 May. Available from: http://www.who.int/mediacentre/factsheets/fs311/en/.
2. Konige M, Wang H, Sztalryd C. Role of adipose specific lipid droplet proteins in maintaining whole body energy homeostasis. Biochim Biophys Acta. 2014; 1842:393–401.


5. Grundy SM, Brewer HB Jr, Cleeman JI, Smith SC Jr, Lenfant C. American Heart Association. National Heart, Lung, and Blood Institute. Definition of metabolic syndrome: Report of the National Heart, Lung, and Blood Institute/American Heart Association conference on scientific issues related to definition. Circulation. 2004; 109:433–438.
7. Siersbaek R, Nielsen R, Mandrup S. PPARgamma in adipocyte differentiation and metabolism--novel insights from genome-wide studies. FEBS Lett. 2010; 584:3242–3249.


8. Bastie C, Holst D, Gaillard D, Jehl-Pietri C, Grimaldi PA. Expression of peroxisome proliferator-activated receptor PPARdelta promotes induction of PPARgamma and adipocyte differentiation in 3T3C2 fibroblasts. J Biol Chem. 1999; 274:21920–21925.


9. He W, Barak Y, Hevener A, Olson P, Liao D, Le J, Nelson M, Ong E, Olefsky JM, Evans RM. Adipose-specific peroxisome proliferator-activated receptor gamma knockout causes insulin resistance in fat and liver but not in muscle. Proc Natl Acad Sci U S A. 2003; 100:15712–15717.
10. Wang ND, Finegold MJ, Bradley A, Ou CN, Abdelsayed SV, Wilde MD, Taylor LR, Wilson DR, Darlington GJ. Impaired energy homeostasis in C/EBP alpha knockout mice. Science. 1995; 269:1108–1112.
11. Lin FT, Lane MD. CCAAT/enhancer binding protein alpha is sufficient to initiate the 3T3-L1 adipocyte differentiation program. Proc Natl Acad Sci U S A. 1994; 91:8757–8761.


12. Wu Z, Rosen ED, Brun R, Hauser S, Adelmant G, Troy AE, McKeon C, Darlington GJ, Spiegelman BM. Cross-regulation of C/EBP alpha and PPAR gamma controls the transcriptional pathway of adipogenesis and insulin sensitivity. Mol Cell. 1999; 3:151–158.


13. Farmer SR. Regulation of PPARgamma activity during adipogenesis. Int J Obes (Lond). 2005; 29:Suppl 1. S13–S16.
14. White UA, Stephens JM. Transcriptional factors that promote formation of white adipose tissue. Mol Cell Endocrinol. 2010; 318:10–14.


15. Bae CR, Park YK, Cha YS. Quercetin-rich onion peel extract suppresses adipogenesis by down-regulating adipogenic transcription factors and gene expression in 3T3-L1 adipocytes. J Sci Food Agric. 2014; Forthcoming.


16. Hayashi K, Narutaki K, Nagaoka Y, Hayashi T, Uesato S. Therapeutic effect of arctiin and arctigenin in immunocompetent and immunocompromised mice infected with influenza A virus. Biol Pharm Bull. 2010; 33:1199–1205.


17. Matsuzaki Y, Koyama M, Hitomi T, Yokota T, Kawanaka M, Nishikawa A, Germain D, Sakai T. Arctiin induces cell growth inhibition through the down-regulation of cyclin D1 expression. Oncol Rep. 2008; 19:721–727.


18. Wu JG, Wu JZ, Sun LN, Han T, Du J, Ye Q, Zhang H, Zhang YG. Ameliorative effects of arctiin from Arctium lappa on experimental glomerulonephritis in rats. Phytomedicine. 2009; 16:1033–1041.


19. Lee S, Shin S, Kim H, Han S, Kim K, Kwon J, Kwak JH, Lee CK, Ha NJ, Yim D, Kim K. Anti-inflammatory function of arctiin by inhibiting COX-2 expression via NF-kappaB pathways. J Inflamm (Lond). 2011; 8:16.
20. Tice A, Ruckle JE, Sultan OS, Kemble S. Access to care: the physician's perspective. Hawaii Med J. 2011; 70:33–38.
21. Kim WK, Kang NE, Kim MH, Ha AW. Peanut sprout ethanol extract inhibits the adipocyte proliferation, differentiation, and matrix metalloproteinases activities in mouse fibroblast 3T3-L1 preadipocytes. Nutr Res Pract. 2013; 7:160–165.


22. Yamasaki M, Ogawa T, Wang L, Katsube T, Yamasaki Y, Sun X, Shiwaku K. Anti-obesity effects of hot water extract from Wasabi (Wasabia japonica Matsum.) leaves in mice fed high-fat diets. Nutr Res Pract. 2013; 7:267–272.


23. Yao X, Zhu F, Zhao Z, Liu C, Luo L, Yin Z. Arctigenin enhances chemosensitivity of cancer cells to cisplatin through inhibition of the STAT3 signaling pathway. J Cell Biochem. 2011; 112:2837–2849.


24. Awale S, Lu J, Kalauni SK, Kurashima Y, Tezuka Y, Kadota S, Esumi H. Identification of arctigenin as an antitumor agent having the ability to eliminate the tolerance of cancer cells to nutrient starvation. Cancer Res. 2006; 66:1751–1757.


25. Ntambi JM, Young-Cheul K. Adipocyte differentiation and gene expression. J Nutr. 2000; 130:3122S–3126S.


26. Ali AT, Hochfeld WE, Myburgh R, Pepper MS. Adipocyte and adipogenesis. Eur J Cell Biol. 2013; 92:229–236.


27. Wang S, Moustaid-Moussa N, Chen L, Mo H, Shastri A, Su R, Bapat P, Kwun I, Shen CL. Novel insights of dietary polyphenols and obesity. J Nutr Biochem. 2014; 25:1–18.


28. Kim JB, Spiegelman BM. ADD1/SREBP1 promotes adipocyte differentiation and gene expression linked to fatty acid metabolism. Genes Dev. 1996; 10:1096–1107.


29. Kim JB, Wright HM, Wright M, Spiegelman BM. ADD1/SREBP1 activates PPARgamma through the production of endogenous ligand. Proc Natl Acad Sci U S A. 1998; 95:4333–4337.
30. Ceddia RB. The role of AMP-activated protein kinase in regulating white adipose tissue metabolism. Mol Cell Endocrinol. 2013; 366:194–203.


31. Daval M, Foufelle F, Ferré P. Functions of AMP-activated protein kinase in adipose tissue. J Physiol. 2006; 574:55–62.


32. Habinowski SA, Witters LA. The effects of AICAR on adipocyte differentiation of 3T3-L1 cells. Biochem Biophys Res Commun. 2001; 286:852–856.


33. Lee H, Kang R, Bae S, Yoon Y. AICAR, an activator of AMPK, inhibits adipogenesis via the WNT/beta-catenin pathway in 3T3-L1 adipocytes. Int J Mol Med. 2011; 28:65–71.