Abstract
BACKGROUND
Acanthopanax divaricatus var. albeofructus (ADA) extract has been reported to have anti-oxidant, immunomodulatory, and anti-mutagenic activity.
MATERIALS/METHODS
We investigated the effects of ADA extract on two mouse models of Alzheimer's disease (AD); intracerebroventricular injection of β-amyloid peptide (Aβ) and amyloid precursor protein/presenilin 1 (APP/PS1)-transgenic mice.
RESULTS
Intra-gastric administration of ADA stem extract (0.25 g/kg, every 12 hrs started from one day prior to injection of Aβ1-42 until evaluation) effectively blocked Aβ1-42-induced impairment in passive avoidance performance, and Aβ1-42-induced increase in immunoreactivities of glial fibrillary acidic protein and interleukin (IL)-1α in the hippocampus. In addition, it alleviated the Aβ1-42-induced decrease in acetylcholine and increase in malondialdehyde levels in the cortex. In APP/PS1-transgenic mice, chronic oral administration of ADA stem extract (0.1 or 0.5 g/kg/day for six months from the age of six to 12 months) resulted in significantly enhanced performance of the novel-object recognition task, and reduced amyloid deposition and IL-1β in the brain.
An immense health burden has been imposed by Alzheimer's disease (AD), for which there is no prevention, and no disease-modifying agents have been approved so far. AD is a progressive neurodegenerative disorder characterized, at least in part, by abnormal accumulation of β-amyloid peptide (Aβ) in the brain [1]. The accumulated Aβ is believed to play an important role in the pathogenesis of AD [2]. Thus, Aβ continues to be an important target for prevention and treatment of AD [3].
For in vivo evaluation of beneficial effects of substances targeting Aβ, some mouse models, including intracerebroventricular (i.c.v.) injection of Aβ1-42 [4], and amyloid precursor protein (APP) transgenic [5] or APP/presenilin 1 (PS1) double transgenic [6] mice, have been used. In the i.c.v. Aβ1-42 injection model, direct toxic effects of i.c.v. administered Aβ1-42 are shown as long-lasting defects in behavioral tests accompanied by pathologic changes in brain tissues [4]. On the other hand, in the APP transgenic mice model, the deleterious effects of progressive overproduction of endogenous Aβ peptides from APP genes are induced [5,6].
Acanthopanax, which has been regarded as having ginseng like activity, has been known as an adaptogen [7], and has been used in the Far East in treatment of various disorders, including stress-induced physiological changes, inflammation, hypertension, and cancer [8]. Among the various species of Acanthopanax genus, Acanthopanax divaricatus var. albeofructus (ADA) extract has been reported to have anti-oxidant [9], immunomodulatory [10], and anti-mutagenic activity [11]. In this study, we investigated the effect of ADA extract in two mouse models of AD: i.c.v. injection of Aβ1-42 [4] and APP/PS1 transgenic mice [6].
Water extracts of ADA stem were provided from Susin Ogapy Co. (Seoul, Korea); 200 grams of ADA were steeped and stirred in 1.5 l of hot distilled water for 16 hrs. The extract was filtered with Whatman filter paper and concentrated with a spray dryer. Sixteen grams was obtained from ADA stem. Procedures for animal experiments were approved by the Animal Experimentation Committee at Hallym University (Hallym 2010-6). For the short-term experiment (Fig. 1A), male ICR mice (4-5 weeks old) were used. An i.c.v. injection of Aβ1-42 (410 pmol/mouse) was done, as described previously [4,12]. For the long-term experiment (Fig. 1B), female APP/PS1 (APPswe/PS1dE9) transgenic mice obtained from Jackson Laboratory (Bar Harbor, ME) were used.
Passive avoidance test was performed as described previously [4]. In brief, one day after injection of Aβ1-42, mice were trained on one-trial step-through passive avoidance task. The passive avoidance box was divided into two compartments, one illuminated and the other dark, equipped with a grid floor. During the training trial, each mouse was placed in the lighted compartment; as soon as it entered the dark compartment, the door was closed and the mouse received an inescapable shock (0.25 mA, 1 s). In the testing trial, given one day after the training trial, the mouse was again placed in the lighted compartment and the time until it entered the dark compartment was measured (the step-through latency maximum testing limit was 300 s). Y-maze task was performed as described previously [4,13]. In brief, the maze was made of black-painted wood and each arm was 25 cm long, 14 cm high, 5 cm wide, and positioned at an equal angle. Mice were placed at the end of one arm and allowed to move freely through the maze during an 8-min session. The series of arm entries was recorded visually, and an arm entry was considered complete when the hind paws of the mouse were completely placed in the arm. Alternation was defined as successive entries into the three arms on overlapping triplet sets. The percentage alternation was calculated as the ratio of actual to possible alternations (defined as the total number of arm entries minus two), multiplied by 100. Novel-object recognition task was performed as described previously [14]. Briefly, mice were exposed to the empty cage for 5 min for two consecutive days in order to accommodate them to the experimental cage. On the third and fourth day, mice were exposed to a cage containing two objects. On the fifth day, one of the objects was replaced with a new one. The percentage of time spent exploring one of the two objects (training session) or the new object (retention session) over the total time spent exploring both objects (exploratory preference) was calculated.
ACh was measured as described previously [4,15]. MDA levels as an index of lipid peroxidation were determined spectrophotometrically using a commercially available kit (Bioxytech MDA-586 kit, Oxis International Inc). ELISA kits for measurement of Aβ and IL-1β were purchased from Bioscience. Assays were performed according to the manufacturer's instructions.
An i.c.v. injection of Aβ1-42 into mice effectively induces Aβ toxicity in vivo, as demonstrated by impairment in passive avoidance performance, increases in immunoreactivities of the astrocyte marker GFAP and IL-1 in the hippocampus, and reduction in ACh levels in the cortex [4,17]. In the current study, we evaluated the effects of ADA extract on various Aβ-induced changes after i.c.v. injection of Aβ1-42 (410 pmol) into male ICR mice. Two different doses of ADA stem extract (0.25 or 0.5 g/kg) were administered intra-gastrically every 12 hrs, five times (two times prior to Aβ1-42 treatment, and three times post-Aβ 1-42 treatment), and the passive avoidance test was performed one day after treatment with Aβ1-42 (Fig. 1A). As shown in Fig. 2A, treatment with ADA stem extract at the dose of 0.25 g/kg effectively blocked the Aβ1-42-induced impairment of performance of the passive avoidance task, while the dose of 0.5 g/kg was less effective. Next, we attempted to determine whether other parts of ADA are also effective, but found that ADA root and leaf were totally ineffective at the dose of 0.25 g/kg (Fig. 2B).
Next, we attempted to determine whether administration of ADA stem extract has protective effects against Aβ1-42-induced changes in cortical ACh and MDA levels, and hippocampal GFAP and IL-1α-immunoreactivities (Fig. 1A). ADA stem extract (0.25 g/kg) was administered intra-gastrically every 12 hrs for six days (from one day before Aβ1-42 treatment to five days post-Aβ 1-42 treatment), and evaluation was performed five days after Aβ1-42 treatment (Fig. 1A). As shown in Fig. 3A,B, i.c.v. injection of Aβ1-42 resulted in significantly decreased levels of ACh and increased levels of MDA, as reported previously [4,18], however, treatment of mice with ADA stem extract (0.25 g/kg) resulted in alleviation of these Aβ1-42-induced changes. As shown in Fig. 3C, i.c.v. injection of Aβ1-42 resulted in increased GFAP- and IL-1α-immunoreactivities in the hippocampus, as reported previously [4,17], however, treatment with ADA stem extract effectively blocked these Aβ1-42-induced changes.
Next, we evaluated the effect of chronic oral administration of ADA stem extract on APP/PS1 transgenic mice. Because AD is more prevalent in women [19], we used female APP/PS1 transgenic mice. ADA stem extract was administered from six months of age for six months at two different doses (0.1 or 0.5 g/kg/day). Because the dose of 0.25 g/kg/12 h of ADA stem extract was effective in the previous Aβ1-42 i.c.v. injection model (Fig. 2A), we used the doses of 0.1 or 0.5 g/kg/day. Each dose of ADA stem extract added to chow was calculated for each mouse to take the dose. After administration of ADA stem extract for six months, i.e. at 12 months of age, behavioral tests (Y-maze and novel-object recognition task) were conducted and measurement of Aβ and IL-1β levels in the brain was performed (Fig. 1B).
Of particular interest, ADA stem extract caused a dose-dependent decrease in body weight (Fig. 4A). The body weight-reducing effect of ADA stem extract does not appear to result from the toxic response, as administration of ADA stem extract did not adversely affect the survival (0 and two months, n = 4, 5, 5 for control, 0.1 g/kg/day, 0.5 g/kg/day, respectively; six months, n = 3, 5, 4 for control, 0.1 g/kg/day, 0.5 g/kg/day, respectively).
At the age of 12 months, APP/PS1-transgenic mice showed no significant changes in spontaneous alternation behavior on the Y-maze test (data not shown). In the novel-object recognition task, treatment with ADA stem extract at the dose of 0.5 g/kg/day resulted in significantly enhanced performance in APP/PS1-transgenic mice (Fig. 4B). Next we examined the effect of treatment with ADA stem extract on levels of Aβ1-40 and Aβ1-42 in APP/PS1-transgenic mice at 12 months of age. As shown in Fig. 4C,D, treatment with ADA stem extract induced a marked decrease in Aβ levels, which was significant at the dose of 0.1 g/kg/day for cortical and hippocampal Aβ1-40 levels, and cortical Aβ1-42 levels, and at the dose of 0.5 g/kg/day for cortical Aβ1-40 and Aβ1-42 levels. In addition, levels of cortical IL-1β were significantly decreased at the dose of 0.1 g/kg/day (Fig. 4E).
In the current study, we observed for the first time the protective effect of ADA stem extract on two different mouse models of Alzheimer's disease based on the Aβ hypothesis of AD: one (an i.c.v. Aβ1-42 injection model) for short-term treatment of ADA, and the other (APP/PS1-transgenic mice model) for long-term treatment of ADA. Previously we reported on the protective effect of long-term (i.e. 4 wks) administration of ferulic acid on i.c.v. Aβ1-42 injection-induced changes, including impairment in performance of the passive avoidance task [4,17,20]. Compared with ferulic acid, which requires pretreatment for four weeks for maximal protection [4], ADA stem extract appears to be much more rapid in imparting protection against i.c.v. Aβ1-42 injection insult, as just one day of pretreatment was effective in this experiment (Fig. 1A). These results suggest that the mechanisms of protection against i.c.v. Aβ1-42 injection insult are different between ferulic acid and ADA stem extract.
In addition to the protective effects of ADA extract against the direct i.c.v.-injected Aβ1-42 toxicities (Figs. 2, 3), ADA extract also effectively inhibited Aβ accumulation in APP/PS1-transgenic mice at a similar dosage range (Fig. 4), indicating that ADA extract has multiple protective mechanisms regarding Aβ toxocity. Although the mechanism of the ADA stem extract-induced beneficial effects in mouse models of Alzheimer's disease was not addressed in the current study, it could be due to, at least in part, the anti-oxidant activity of ADA extract [9]. Anti-oxidants have been shown to be beneficial in animal models of AD [21]. It was remarkable that only the extract of stem but not of root nor of leaf was effective (Fig. 2B). The responsible molecules in the ADA stem extract for these beneficial effects remain to be identified. As there are at least 18 species in the Acanthopanax genus [8], the effects of other species of Acanthopanax genus on these AD animal models need be examined. The unexpected finding of the weight-reducing effect of ADA (Fig. 4A) also warrants further study.
The limitations of the current study are as follows: although the passive avoidance test and novel-object recognition task are the established behavioral tests for evaluation of learning and memory performance, the Morris water maze test would be helpful for evaluation of the protective effects of ADA on spacial memory in both the i.c.v. Aβ injection model and APP/PS1-transgenic mice model. In addition, although the current study showed that ADA treatment has anti-inflammatory activity, as demonstrated by its inhibition of the Aβ 1-42-induced increase in hippocampal IL-1α immunoreactivity, and in its reduction of brain IL-1β in APP/PS1-transgenic mice, more extensive study on the effects of ADA on other inflammatory parameters, including TNF-α and nitric oxide, would be needed for more definitive evaluation of its anti-inflammatory activity in these AD models. An additional limitation of this study is that the active ingredient(s) of ADA for the beneficial effects observed in the current study were not identified. Our findings indicate that ADA extract of stem, but not leaf and root, would be a good starting point for purification of the active principles of ADA.
In conclusion, administration of ADA stem extract showed protective activity against Aβ-related toxicity, which was elicited by either i.c.v. injection of Aβ or over-expression of APP/PS1 mutant genes, in both behavioral and neuropathological aspects. These results suggest that ADA stem extract may be useful for prevention and treatment of AD. In light of increasing burden of AD in modern society in the absence of approved preventive measures, development of ADA stem extract, which has been widely used as a tonic in Korea, demonstrating no toxicity, as a neutraceutical for prevention of AD could be useful. A clinical study on the preventive effect of ADA stem extract in pre-AD subjects (i.e. patients with mild cognitive impairment) is warranted.
References
1. Huang Y, Mucke L. Alzheimer mechanisms and therapeutic strategies. Cell. 2012; 148:1204–1222. PMID: 22424230.


2. Maltsev AV, Bystryak S, Galzitskaya OV. The role of β-amyloid peptide in neurodegenerative diseases. Ageing Res Rev. 2011; 10:440–452. PMID: 21406255.


3. Lemere CA, Masliah E. Can Alzheimer disease be prevented by amyloid-beta immunotherapy? Nat Rev Neurol. 2010; 6:108–119. PMID: 20140000.
4. Yan JJ, Cho JY, Kim HS, Kim KL, Jung JS, Huh SO, Suh HW, Kim YH, Song DK. Protection against beta-amyloid peptide toxicity in vivo with long-term administration of ferulic acid. Br J Pharmacol. 2001; 133:89–96. PMID: 11325798.
5. Lim GP, Chu T, Yang F, Beech W, Frautschy SA, Cole GM. The curry spice curcumin reduces oxidative damage and amyloid pathology in an Alzheimer transgenic mouse. J Neurosci. 2001; 21:8370–8377. PMID: 11606625.


6. Jantzen PT, Connor KE, DiCarlo G, Wenk GL, Wallace JL, Rojiani AM, Coppola D, Morgan D, Gordon MN. Microglial activation and beta-amyloid deposit reduction caused by a nitric oxide-releasing nonsteroidal anti-inflammatory drug in amyloid precursor protein plus presenilin-1 transgenic mice. J Neurosci. 2002; 22:2246–2254. PMID: 11896164.
7. Brekhman II, Dardymov IV. New substances of plant origin which increase nonspecific resistance. Annu Rev Pharmacol. 1969; 9:419–430. PMID: 4892434.


8. Yook CS. Medicinal Herbs of Acanthopanax in Asia. Seoul: Kyeongwon Media;2001.
9. Kim JY, Yang KS. Screening of antioxidant activity of Acanthopanax species in vitro. Yakhak Hoeji. 2003; 47:361–364.
10. Lyu SY, Park WB. Th1/Th2 cytokine modulation in human PBMC by Acanthopanax divaricatus var. albeofructus. Food Sci Biotechnol. 2008; 17:631–636.
11. Hong CE, Cho MC, Jang HA, Lyu SY. Mutagenicity and antimutagenicity of Acanthopanax divaricatus var. albeofructus. J Toxicol Sci. 2011; 36:661–668. PMID: 22008541.


12. Laursen SE, Belknap JK. Intracerebroventricular injections in mice. Some methodological refinements. J Pharmacol Methods. 1986; 16:355–357. PMID: 3784576.
13. Sarter M, Bodewitz G, Stephens DN. Attenuation of scopolamine-induced impairment of spontaneous alteration behaviour by antagonist but not inverse agonist and agonist beta-carbolines. Psychopharmacology (Berl). 1988; 94:491–495. PMID: 2836875.
14. Tang YP, Shimizu E, Dube GR, Rampon C, Kerchner GA, Zhuo M, Liu G, Tsien JZ. Genetic enhancement of learning and memory in mice. Nature. 1999; 401:63–69. PMID: 10485705.


15. Israël M, Lesbats B. Application to mammalian tissues of the chemiluminescent method for detecting acetylcholine. J Neurochem. 1982; 39:248–250. PMID: 7045285.


16. Baker H, Farbman AI. Olfactory afferent regulation of the dopamine phenotype in the fetal rat olfactory system. Neuroscience. 1993; 52:115–134. PMID: 8094544.


17. Cho JY, Kim HS, Kim DH, Yan JJ, Suh HW, Song DK. Inhibitory effects of long-term administration of ferulic acid on astrocyte activation induced by intracerebroventricular injection of beta-amyloid peptide (1-42) in mice. Prog Neuropsychopharmacol Biol Psychiatry. 2005; 29:901–907. PMID: 15970368.
18. Fu AL, Dong ZH, Sun MJ. Protective effect of N-acetyl-L-cysteine on amyloid beta-peptide-induced learning and memory deficits in mice. Brain Res. 2006; 1109:201–206. PMID: 16872586.
19. Webber KM, Casadesus G, Perry G, Atwood CS, Bowen R, Smith MA. Gender differences in Alzheimer disease: the role of luteinizing hormone in disease pathogenesis. Alzheimer Dis Assoc Disord. 2005; 19:95–99. PMID: 15942328.
20. Kim HS, Cho JY, Kim DH, Yan JJ, Lee HK, Suh HW, Song DK. Inhibitory effects of long-term administration of ferulic acid on microglial activation induced by intracerebroventricular injection of beta-amyloid peptide (1-42) in mice. Biol Pharm Bull. 2004; 27:120–121. PMID: 14709913.


21. Dumont M, Beal MF. Neuroprotective strategies involving ROS in Alzheimer disease. Free Radic Biol Med. 2011; 51:1014–1026. PMID: 21130159.
Fig. 1
Experimental schedules for evaluation of the effects of ADA extract on mouse models of i.c.v. injection of Aβ1-42 (A) and APP/PS1-transgenic mice (B).
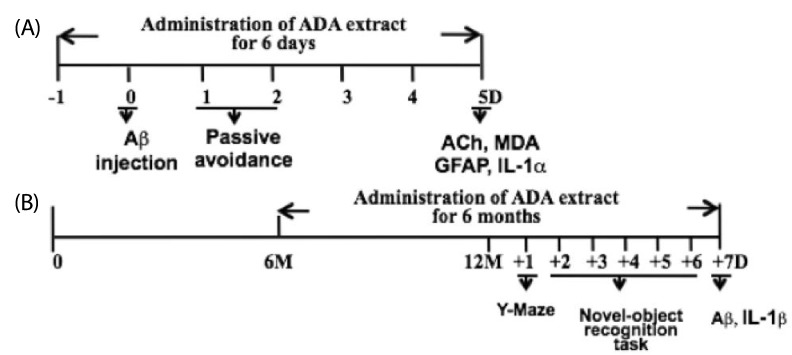
Fig. 2
Protective effect of ADA stem extract on Aβ1-42-induced impairment of passive avoidance performance. (A). ADA stem extract (0.25 g/kg or 0.5 g/kg) was administered intra-gastrically every 12 hrs, five times (two times prior to Aβ1-42 treatment, and three times post-Aβ1-42 treatment). Passive avoidance test was performed one day after Aβ1-42 treatment. (B). Effects of different parts of ADA extract (0.25 g/kg) given with the same protocol on the Aβ1-42-induced impairment of passive avoidance performance. *P < 0.05, **P < 0.01 vs control. #P < 0.05, ##P < 0.01 vs Aβ1-42 alone. Data are expressed as mean ± SEM (n = 10-12).

Fig. 3
Protective effects of ADA stem extract on Aβ1-42-induced changes in cortical acetylcholine (ACh) and malondialdehyde (MDA) as well as hippocampal GFAP- and IL-1α-immunoreactivities. ADA stem extract (0.25 g/kg) was administered intra-gastrically every 12 hrs for six days (from one day before Aβ1-42 treatment to five days post-Aβ1-42 treatment), and evaluation was performed five days after Aβ1-42 treatment. *P < 0.05, **P < 0.01 vs control. #P < 0.05 vs Aβ 1-42 alone. Data are expressed as mean ± SEM (n = 10).

Fig. 4
Effect of ADA stem extract treatment for six months on body weight (A), novel-object recognition task (B), brain Aβ (C,D) and IL-1β (E) levels in APP/PS1-transgenic mice at 12 months of age.
*P < 0.05, **P < 0.01, ***P < 0.001 vs control. #P < 0.05 vs ADA (0.1 g/kg/day). Data are expressed as mean ± SEM (a: 0 and two months, n = 4, 5, 5 for control, 0.1 g/kg/day, 0.5 g/kg/day, respectively; six months, n = 3, 5, 4 for control, 0.1 g/kg/day, 0.5 g/kg/day, respectively; b-e: n = 3, 5, 4 for control, 0.1 g/kg/day, 0.5 g/kg/day, respectively).
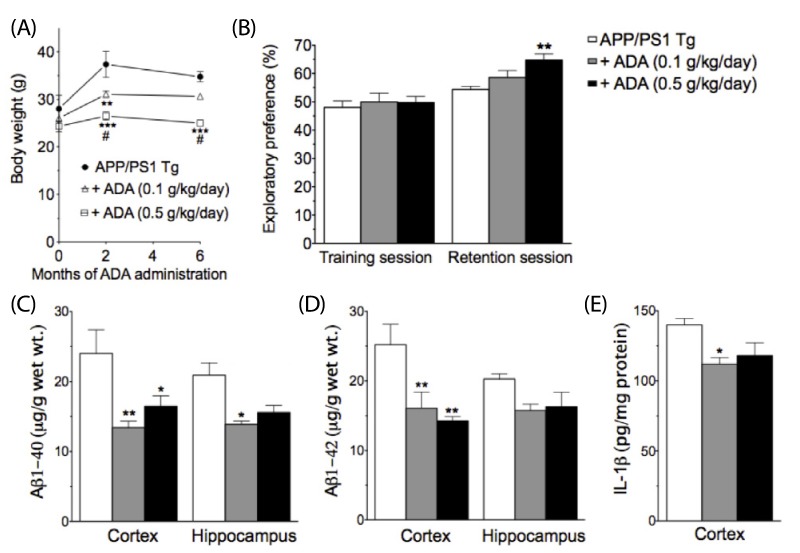