Abstract
BACKGROUND/OBJECTIVES
This study investigated the antioxidant activities and hepatoprotective effects of Schisandra chinensis Baillon extract (SCE) against tert-butyl hydroperoxide (t-BHP)-induced oxidative hepatic damage in rats.
MATERIALS/METHODS
Sprague-Dawley (SD) rats were pretreated with SCE (300, 600, and 1,200 mg/kg BW) or saline once daily for 14 consecutive days. On day 14, each animal, except those belonging to the normal control group, were injected with t-BHP (0.8 mmol/kg BW/i.p.), and all of the rats were sacrificed 16 h after t-BHP injection.
RESULTS
Although no significant differences in AST and ALT levels were observed among the TC and SCE groups, the high-dose SCE group showed a decreasing tendency compared to the TC group. However, erythrocyte SOD activity showed a significant increase in the low-dose SCE group compared with the TC group. On the other hand, no significant differences in hepatic total glutathione (GSH) level, glutathione reductase (GR), and glutathione peroxidase (GSH-Px) activities were observed among the TC and SCE groups. Hepatic histopathological evaluation revealed that pretreatment with SCE resulted in reduced t-BHP-induced incidence of lesions, such as neutrophil infiltration, swelling of liver cells, and necrosis. In particular, treatment with a high dose of SCE resulted in induction of phase II antioxidant/detoxifying enzyme expression, such as glutathione S-transferase (GST) and glutamate-cysteine ligase catalytic subunit (GCLC).
CONCLUSIONS
Based on these results, we conclude that SCE exerts protective effects against t-BHP induced oxidative hepatic damage through the reduction of neutrophil infiltration, swelling of liver cells, and necrosis. In addition, SCE regulates the gene expression of phase II antioxidant/detoxifying enzymes independent of hepatic antioxidant enzyme activity.
Reactive oxygen species (ROS)-derived oxidative stress plays an important role in the pathogenesis of various diseases, including cancer, cardiovascular diseases, and inflammation [1]. Endogenous antioxidants have evolved to meet the very basic requirement of overcoming the oxidative stresses that occur during normal metabolic processes. However, when the endogenous antioxidant defense systems are overwhelmed by ROS, oxidative stress occurs and ultimately leads to development of various pathological conditions [2,3]. Antioxidant systems, such as antioxidant vitamins, superoxide dismutase (SOD), catalase, glutathione (GSH), and glutathione peroxidase (GSH-Px) protect cells against lipid peroxidation, which is the basis of many pathologic processes [4]. Erythrocytes and liver contain enzymes that contribute to the anti-oxidant defense mechanism. Erythrocytes are constantly exposed to both extracellular and intracellular sources of reactive oxygen species (ROS). In addition, there is a high probability that the liver will be subjected to oxidative stress-induced toxicity [5] because it is an important organ for the biotransformation and detoxification of toxic substances and oxidative agents [6]. Phase I and II biotransformation enzymes in the liver are involved in metabolic activation and detoxification of various toxic xenobiotics. Phase I enzymes convert xenobiotics to active intermediates, and phase II enzymes catalyze the conjugation of these active intermediates with endogenous cofactors and facilitate their excretion [7]. In particular, the induction of phase II detoxifying/antioxidant enzymes, such as glutathione S-transferase (GST), glutamate-cysteine ligase (GCL), which includes a catalytic subunit (GCLC) and a modifier subunit (GCLM), and heme oxygenase-1 (HO-1), results in detoxification of xenobiotics and inactivation of ROS [8].
Tert-butylhydroperoxide (t-BHP), a cytotoxin and oxidative agent, has often been used as a model in investigation of the mechanism of hepatocyte injury initiated by acute oxidative stress. T-BHP can be metabolized to free radical intermediates, such as peroxyl and alkoxyl radicals, by cytochrome P 450 in hepatocytes [9]. In addition, the detoxification of t-BHP can be achieved by GSH-mediated GSH-Px catalyzed reactions to yield t-butanol and glutathione disulfide (GSSG) [10]. GSSG is then reduced to GSH by glutathione reductase (GR). Free radical intermediates induced by t-BHP can subsequently initiate lipid peroxidation and GSH depletion and can lead to oxidative-induced hepatocyte damage [11]. Under oxidative stress, the intake of exogenous antioxidant compounds from a dietary source that enhances the biological antioxidant defense systems can prevent oxidative-induced liver damage [12].
Schisandra chinensis Baillon, a member of the Magnoliaceae family, grows wild in Korea, Russia, China, and Japan [13]. In a previous study, in vitro antioxidant activity of Schisandra chinensis Baillon extracts (SCE) was demonstrated through chemical assays [14]. In addition, its various dibenzocyclooctadiene lignans, including schisandrin, schisandrin B, and gomisin A, have been shown to exert antioxidant activities and hepatoprotective effects in vitro [15] and in vivo [16,17]. This study investigated the endogenous antioxidant capacity of SCE as an exogenous antioxidant dietary source against t-BHP-induced oxidative hepatic damage in rats.
Schisandra chinensis Baillon (Mungyeong, Gyeongbuk, Korea) was provided by the Rural Development Administration (Suwon, Gyeonggi, Korea). The fruits of Schisandra chinensis Baillon (100 g) were extracted with 1 L of 60% ethanol at 60℃ for 6 h. After removal of the cake by filtration, the extract was freeze-dried under vacuum for five days. The manufacturing yield and the water contents were 32.4% and 10.75%, respectively. SCE was analyzed for schisandrin, Gomisin N, Gomisin A, and Deoxyschisandrin, and such. The content of schisandrin (8.3 mg per 100 g SCE) as a marker compound of the Schisandra chinensis Baillon extract was analyzed by HPLC (Waters Alliance HPLC Systems, USA) using a Luna C18 column (4.5 × 250 mm, 5 µm, Phenomenex Inc., Torrance, CA, USA).
Seven-week-old male Sprague-Dawley rats (Orientbio Inc., Seongnam, Gyeonggi, Korea) were individually housed in stainless steel wire-mesh cages under laboratory conditions of 23 ± 1℃ with a 12 h light / 12 h dark cycle and 45 ± 5% humidity. Rats (200-220 g, n = 60) were fed a commercial pellet diet (Samyang, Seoul, Korea) for seven days for acclimatization and randomized into six groups: NC, normal control; TC, t-BHP control; SCE-L, 300 mg/kg BW SCE; SCE-M, 600 mg/kg BW SCE; and SCE-H, 1,200 mg/kg BW SCE. Prior to t-BHP injection, the animals received daily administration of SCE by gavage for 14 days. On day 14, t-BHP was injected intraperitoneally (i.p., 0.8 mmol/kg B.W.) into each animal, except for animals in the NC group. After 16 hours, the animals were anesthetized, and their blood was collected by cardiac puncture. The blood samples were centrifuged (3000 × g, 4℃, 30 min) in order to separate the serum. Erythrocytes were separated from the pellet after separation of the plasma layer and stored at -80℃. The liver, kidney, and spleen were removed, weighed, snap-frozen in liquid nitrogen, and stored at -80℃. The experimental protocol was approved by the Institutional Animal Care and Use Committee (IACUC) of the Ewha Womans University of Korea (Approval No. 2012-01-033).
Serum aspartate aminotransferase (AST) and alanine aminotransferase (ALT) activities were measured using commercial kits (Asan Pharmaceutical, Seoul, Korea) based on the method developed by Reitman-Frankel. Absorbance was determined using a microplate reader (Eon Microplate Spectrophotometer, BioTek Instruments, Inc, Winooski, Vermont, USA) at 505 nm.
The formalin-fixed liver tissue samples were dehydrated through a gradient series (70-100%) of ethyl alcohol, dealcoholized in xylene, embedded in paraffin, and sectioned (5 µm thickness). For histological analysis, the slide-mounted embedded tissues were deparaffinized in xylene, rehydrated in a reverse-gradient series of ethyl alcohol, and stained with hematoxylin and eosin (H&E). The dehydrated and dealcoholized formalin-fixed tissue sections were mounted on slides with Canada balsam, and tissue damage was observed using an Olympus 51 microscope (Olympus Optical Co., Tokyo, Japan).
Total RNA was extracted from the liver samples using TRIzol (Invitrogen Co., San Diego, CA, USA). The RNA concentration and quality were determined using a BioSpec-nano spectrophotometer (Shimadzu Corp., Kyoto, Japan). cDNA was synthesized using a High-Capacity RNA-to-cDNA kit (Applied Biosystems, Foster City, CA, USA). Quantitative RT-PCR was performed using the TaqMan method with the Step-One-Plus RT-PCR System (Applied Biosystems). The primer sets for the target genes were Heme Oxygenase-1 (HO-1, Rn01536933_m1), glutathione S-transferase (GST, Rn01446656_m1), glutamate-cysteine ligase catalytic subunit (GCLC, Rn00689046_m1), glutamate-cysteine ligase modifier subunit (GCLM, Rn00568900_m1), and β-actin (Rn0066 7869_m1). Amplifications were performed starting with a 10-min template denaturation step at 95℃, which was followed by 40 cycles at 95℃ for 15 s and 60℃ for 1 min. The relative amounts of these mRNAs were normalized to the amount of β-actin, and the relative amounts of the RNAs were calculated using the comparative CT method.
The superoxide dismutase (SOD) activities of the erythrocytes were determined using the method developed by Miquel [18]. One unit of SOD was defined as the amount of enzyme that inhibits 50% of cytochrome c in a xanthine-xanthine oxidase system. Erythrocyte catalase (CAT) activity was measured by production of formaldehyde from the decomposition of H2O2, as described by Johnson et al. [19].
Total glutathione (GSH) concentrations were quantified using the glutathione reductase recycling assay as described by Akerboom et al. [20]. Glutathione Reductase (GR) activity was assayed as described by Foyer et al. with some modifications [21]. GR activity was expressed as nmoles of NADPH per minute per milligram of protein using the following equation, with a molar extinction coefficient for NADPH at 340 nm. Glutathione peroxidase (GSH-Px) activity was determined using the modified method of Mohandas et al. [22]. GSH-Px activity was expressed as nmoles of NADPH oxidized to NADP per minute per milligram of protein using the following equation, with a molar extinction coefficient for NADPH at 340 nm.
Plasma malondialdehyde (MDA) was estimated using the method of thiobarbituric acid reactive substances (TBARS), which primarily reflects malondialdehyde (MDA), as previously described [23]. The absorbance of the organic layer was measured at 535 and 572 nm using a microplate reader (Eon Microplate Spectrophotometer, BioTek® Instruments, Inc, Winooski, Vermont, USA).
All statistical analyses were performed using the SAS 9.2 program (SAS Institute, Cary, NY, USA). The values are presented as the mean and standard error (S.E.). The significance of the differences between the NC and TC groups was analyzed using the Student's t-test (**P ≤ 0.01). The significance of the differences between the TC and SCEs groups was analyzed using one-way analysis of variance (ANOVA) with post-hoc Dunnett's multiple-comparison test (#P ≤ 0.05 and ##P ≤ 0.01).
Significantly higher serum ALT activity was observed in the TC group compared with that in the NC group (68.94 ± 12.71 vs. 10.44 ± 0.57, P = 0.0013, Fig. 1A). No significant differences in the levels of ALT were observed in the SCE groups compared with the TC group, however, that of the SCE-H group was lowered compared to the TC group. A significant increase in serum AST activity was observed in the TC group compared to the NC group (206.15 ± 41.03 vs. 29.86 ± 1.83, P = 0.002, Fig. 1B). Although no significant differences were observed among the TC and SCE groups, that of the SCE-H group was lowered compared to the TC group.
Livers of the NC group showed a regular arrangement of hepatocytes with clearly visible nuclei (Fig. 2A). However, those of the TC group showed moderate neutrophil infiltration (sharp arrow), swelling (arrow), and necrosis (arrowhead, Fig. 2B). Swelling and necrosis of hepatocytes in the SCE-L group were decreased compared with the TC group (Fig. 2C). The SCE-M and SCE-H groups showed only swelling and almost normal morphology (Fig. 2D and 2E). Pretreatment with SCE resulted in reduced incidence of lesions, such as neutrophil infiltration, swelling of hepatocytes, and necrosis, induced by injection of t-BHP.
Hepatic HO-1, GST, GCLC, and GCLM mRNA levels were measured using quantitative RT-PCR in order to determine the effects of SCE on phase II detoxification and antioxidant enzymes (Fig. 3). HO-1 expression in the TC group tended to be higher than that observed in the NC group. However, a marked decrease was observed in the SCE-L and SCE-M groups compared with the TC group, although no significant differences were observed among the groups (Fig. 3A). The mRNA levels of GST, GCLC, and GCLM were lowered significantly by t-BHP injection compared to the NC group (P = 0.0006, P < 0.0001, and P = 0.046, respectively).
Conversely, the GST, GCLC, and GCLM mRNA levels in the SCE-pretreated groups showed a dose-dependent increase with SCE (Fig. 3B, 3C and 3D). In particular, significantly higher mRNA levels of GST and GCLC were observed in the SCE-H group than in the TC group (P = 0.0177 and P = 0.0097, respectively). No significant difference in the GCLM mRNA level was observed between the TC and SCEs groups, although the SCE-H group showed an increasing tendency compared to the levels observed in the TC group.
In erythrocytes, the SOD activity was lowered significantly in the TC group compared with the NC group (15.60 ± 2.40 vs. 9.97 ± 0.83, P = 0.0483, Table 1). The SCE-M group tended to exhibit higher SOD activity than the TC group, and the SCE-L group showed a significant increase in SOD activity compared with the TC group (9.97 ± 0.83 vs. 16.82 ± 1.77, P < 0.0001). Nonetheless, no significant difference in catalase activity was observed among the groups. In the liver, the total GSH and GR activities did not differ significantly among the groups. In addition, no significant difference in plasma TBARS level and hepatic GSH-Px activity was observed among the groups.
We evaluated the endogenous antioxidant capacity and hepatoprotective potential of SCE against t-BHP-induced oxidative liver damage in rats. T-BHP induces lipid peroxidation, damages the membrane of liver cells and organelles, causes swelling and necrosis of hepatocytes, and results in release of cytosolic enzymes, such as ALT and AST, into the circulating blood [24]. In the current study, the serum ALT and AST activities tended to decrease after pretreatment of rats with a high dose of SCE. In addition, SCE caused attenuation of liver lesions in rats with t-BHP-induced oxidative damage. The hepatic histopathological evaluation revealed that pretreatment with SCE resulted in reduced t-BHP-induced incidence of lesions, such as neutrophil infiltration, hepatocyte swelling, and necrosis.
The body has an effective mechanism for prevention and neutralization of the damage induced by free radicals. This mechanism is accomplished by a set of endogenous antioxidant enzymes, including SOD, catalase, GSH, and GSH-Px. SOD neutralizes the superoxide anion that plays a pivotal role in oxidative stress-induced cellular damage by transforming it into hydrogen peroxide and thereby preventing formation of highly aggressive compounds, such as peroxynitrite and hydroxyl radical [26]. Erythrocytes are extremely vulnerable to these oxidative challenges [25]. SOD and CAT are considered to be a primary defense that protects biological macromolecules from oxidative damage. Intake of exogenous antioxidant compounds from a dietary source that enhances the biological antioxidant systems can prevent oxidative damage [12]. In this study, pretreatment with a low dose of SCE prevented the decrease in the erythrocyte SOD activity induced by t-BHP. However, catalase activities did not differ significantly among the groups. Next, we focused on hepatic phase II antioxidant/detoxifying enzymes because we used a t-BHP-induced oxidative hepatic damage model. Liver is known to undergo free radical-mediated injury, and increased ROS is related to damage of hepatic anti-oxidant defense. Antioxidants are of direct and indirect types. Direct antioxidants, such as GSH and ascorbic acid, are substances that can participate in physiological, biochemical, and cellular processes involved in inactivation of free radicals and/or that prevent free radical-initiated chemical reactions. In contrast, indirect antioxidants boost the antioxidant capacity of cells and thereby afford protection against oxidative stress. There is substantial evidence indicating that phase II enzymes, such as GSTs, NAD(P)H, and heme oxygenase, play important roles in detoxification of electrophiles and that their induction protects animals and their cells against carcinogenesis and mutagenesis [27,28]. Induction of phase II detoxifying/antioxidant enzymes, such as GST, GCLC, GCLM, and HO-1, results in detoxification of xenobiotics and inactivation of ROS [8]. In particular, GST catalyzes the conjugation of electrophilic molecules with GSH and thereby protects cellular macromolecules against toxic foreign chemicals and oxidative stress [7]. In addition, GCL is the rate-limiting enzyme in the overall glutathione synthesis pathway. In this study, no significant differences in hepatic total glutathione (GSH) level, glutathione reductase (GR), and glutathione peroxidase (GSH-Px) activities were observed among the TC and SCE groups. On the other hand, treatment with a high dose of SCE resulted in induction of phase II antioxidant/detoxifying enzyme expression, such as glutathione S-transferase (GST) and glutamate-cysteine ligase catalytic subunit (GCLC). The major bioactive components of SCE include schisandrin, Gomisin N, Gomisin A, and Deoxyschisandrin, and such. Among them, schisandrin is the highest, at 8.3 mg per 100 g SCE. Previous studies have demonstrated that schisandrin exhibits anti-oxidative effects in vivo [16]. In particular, the methylenedioxy group of the schisandrin molecule is an important structural determinant for enhancing the hepatic mitochondrial glutathione and hence protecting against CCl4 hepatotoxicity in mice [29]. LD50 value of ethanol extract of Schisandra chinensis was estimated to be 35.63 ± 6.46 g/kg [30]. In addition, Ryu et al. investigated acute toxicity of the fruit figment of SCE in mice following oral administration at the doses of 1, 2, and 4 g/kg, without abnormal behaviors, body weight, or other toxic symptoms for 14 days [31]. Furthermore, the activities of AST and ALT were decreased by 92% and 87%, respectively, by administration of 5 g SCE/kg BW in rats with CCl4-induced liver injury [32]. In addition, 1.2 g/kg as an animal dose is applicable to 0.72 g/day as a human dose, depending on the safety factor. Therefore, in this study, medium or high-doses of SCE were free from undesirable side effects.
In conclusion, we suggest that SCE is a potent inducer of phase II detoxification enzymes and exerts protective effects against t-BHP-induced oxidative hepatic damage. In particular, treatment with SCE resulted in reduced neutrophil infiltration, swelling of liver cells, and necrosis in liver. Induction of phase II antioxidant and detoxifying enzymes such as GST, GCLC, and GCLM mRNA expression might contribute to antioxidant potential of SCE via detoxification of xenobiotics and inactivation of ROS. However, SCE regulates gene expression of phase II antioxidant/detoxifying enzymes independent of hepatic antioxidant enzyme activity. Conduct of further studies will be needed in order to provide a logical explanation for interaction between these parameters.
Figures and Tables
Fig. 1
Effects of Schisandra chinensis Baillon on serum (A) ALT and (B) AST activities in SD rats injected with t-BHP. Each bar represents the mean ± S.E. The significance of the differences between the NC and TC groups was analyzed by Student's t-test (**P ≤ 0.01). ALT, alanine aminotransferase; AST, aspartate aminotransferase
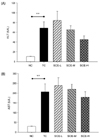
Fig. 2
Effect of Schisandra chinensis Baillon on histopathologic changes in liver of SD rats injected with t-BHP. Hematoxylin-eosin staining; original magnification, 400X; sharp arrow, neutrophil infiltration; arrow, swelling; arrowhead, necrosis. (A) NC, normal control, saline; (B) TC, t-BHP control, t-BHP; (C) SCE-L, low-dose SCE, t-BHP + 300 mg/kg B.W. SCE; (D) SCE-M, medium-dose SCE, t-BHP + 600 mg/kg B.W. SCE; and (E) SCE-H, high-dose SCE, t-BHP + 1200 mg/kg B.W. SCE.
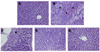
Fig. 3
Effects of Schisandra chinensis Baillon administration on hepatic mRNA expression of (A) HO-1, (B) GST, (C) GCLC, and (D) GCLM in SD rats injected with t-BHP. Each bar represents the mean ± S.E. The significance of the differences between the NC and TC groups was analyzed by Student's t-test (**P ≤ 0.01). The significance of the differences between the TC and SCEs groups was analyzed by one-way analysis of variance (ANOVA) with post-hoc Dunnett's multiple-comparison test (#P ≤ 0.05 and ##P ≤ 0.01). HO-1, heme oxygenase-1; GST, glutathione S-transferase; GCLC, glutamate-cysteine ligase catalytic subunit; GCLM, glutamate-cysteine ligase modifier subunit.
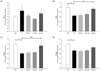
Table 1
Effects of Schizandra chinensis Baillon extract administration on antioxidant activities and lipid peroxidation in SD rats injected with t-BHP
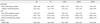
Mean ± S.E. (n = 10)
The significance of the differences between the NC and TC groups was analyzed by Student's t-test (*P ≤ 0.05). The significance of the differences between the TC and SCEs groups was analyzed by one-way analysis of variance (ANOVA) with post-hoc Dunnett's multiple-comparison test (##P ≤ 0.01).
SOD, superoxide dismutase; GSH, glutathione; GR, glutathione reductase; GSH-Px, glutathione peroxidase
ACKNOWLEDGMENTS
We would like to thank the Rural Development Administration (Suwon, Gyeonggi, Korea) for the gift of the Schisandra chinensis Baillon (Mungyeong, Gyeongbuk, Korea).
References
1. Kris-Etherton PM, Lefevre M, Beecher GR, Gross MD, Keen CL, Etherton TD. Bioactive compounds in nutrition and health-research methodologies for establishing biological function: the antioxidant and anti-inflammatory effects of flavonoids on atherosclerosis. Annu Rev Nutr. 2004; 24:511–538.


2. Bandyopadhyay U, Das D, Banerjee RK. Reactive oxygen species: oxidative damage and pathogenesis. Curr Sci. 1999; 77:658–666.
3. Chandra J, Samali A, Orrenius S. Triggering and modulation of apoptosis by oxidative stress. Free Radic Biol Med. 2000; 29:323–333.


4. Kanter M, Coskun O, Budancamanak M. Hepatoprotective effects of Nigella sativa L and Urtica dioica L on lipid peroxidation, antioxidant enzyme systems and liver enzymes in carbon tetrachloride-treated rats. World J Gastroenterol. 2005; 11:6684–6688.


6. Yang J, Li Y, Wang F, Wu C. Hepatoprotective effects of apple polyphenols on CCl4-induced acute liver damage in mice. J Agric Food Chem. 2010; 58:6525–6531.


7. Hayes JD, Flanagan JU, Jowsey IR. Glutathione transferases. Annu Rev Pharmacol Toxicol. 2005; 45:51–88.


8. Giudice A, Montella M. Activation of the Nrf2-ARE signaling pathway: a promising strategy in cancer prevention. Bioessays. 2006; 28:169–181.


9. Minotti G, Borrello S, Palombini G, Galeotti T. Cytochrome P-450 deficiency and resistance to t-butyl hydroperoxide of hepatoma microsomal lipid peroxidation. Biochim Biophys Acta. 1986; 876:220–225.


10. Sies H, Summer KH. Hydroperoxide-metabolizing systems in rat liver. Eur J Biochem. 1975; 57:503–512.


11. Martín C, Martínez R, Navarro R, Ruiz-Sanz JI, Lacort M, Ruiz-Larrea MB. tert-Butyl hydroperoxide-induced lipid signaling in hepatocytes: involvement of glutathione and free radicals. Biochem Pharmacol. 2001; 62:705–712.
12. Havsteen BH. The biochemistry and medical significance of the flavonoids. Pharmacol Ther. 2002; 96:67–202.


13. Hancke JL, Burgos RA, Ahumada F. Schisandra chinensis (Turcz.) baill. Fitoterapia. 1999; 70:451–471.


14. Jung CH, Hong MH, Kim JH, Lee JY, Ko SG, Cho K, Seog HM. Protective effect of a phenolic-rich fraction from Schisandra chinensis against H2O2-induced apoptosis in SH-SY5Y cells. J Pharm Pharmacol. 2007; 59:455–462.


15. Smejkal K, Slapetová T, Krmencík P, Kubínová R, Suchý P, Dall'Acqua S, Innocenti G, Vanco J, Kalvarová K, Dvorská M, Slanina J, Kramárová E, Muselík J, Zemlicka M. Evaluation of the antiradical activity of Schisandra chinensis lignans using different experimental models. Molecules. 2010; 15:1223–1231.


16. Ip SP, Ko KM. The crucial antioxidant action of schisandrin B in protecting against carbon tetrachloride hepatotoxicity in mice: a comparative study with butylated hydroxytoluene. Biochem Pharmacol. 1996; 52:1687–1693.


17. Teraoka R, Shimada T, Aburada M. The molecular mechanisms of the hepatoprotective effect of gomisin A against oxidative stress and inflammatory response in rats with carbon tetrachloride-induced acute liver injury. Biol Pharm Bull. 2012; 35:171–177.


18. Miquel J, Quintanilha AT, Weber H. CRC Handbook of Free Radicals and Antioxidants in Biomedicine. Volume II. Boca Raton (FL): CRC Press;1989.
19. Johansson LH, Borg LA. A spectrophotometric method for determination of catalase activity in small tissue samples. Anal Biochem. 1988; 174:331–336.


20. Akerboom TP, Sies H. Assay of glutathione, glutathione disulfide, and glutathione mixed disulfides in biological samples. Methods Enzymol. 1981; 77:373–382.
21. Bellomo G, Mirabelli F, DiMonte D, Richelmi P, Thor H, Orrenius C, Orrenius S. Formation and reduction of glutathione-protein mixed disulfides during oxidative stress. A study with isolated hepatocytes and menadione (2-methyl-1,4-naphthoquinone). Biochem Pharmacol. 1987; 36:1313–1320.


22. Mohandas J, Marshall JJ, Duggin GG, Horvath JS, Tiller DJ. Differential distribution of glutathione and glutathione-related enzymes in rabbit kidney. Possible implications in analgesic nephropathy. Biochem Pharmacol. 1984; 33:1801–1807.


23. Jentzsch AM, Bachmann H, Fürst P, Biesalski HK. Improved analysis of malondialdehyde in human body fluids. Free Radic Biol Med. 1996; 20:251–256.


24. Xiong Q, Hase K, Tezuka Y, Tani T, Namba T, Kadota S. Hepatoprotective activity of phenylethanoids from Cistanche deserticola. Planta Med. 1998; 64:120–125.


25. Vijayakumar RS, Nalini N. Efficacy of piperine, an alkaloidal constituent from Piper nigrum on erythrocyte antioxidant status in high fat diet and antithyroid drug induced hyperlipidemic rats. Cell Biochem Funct. 2006; 24:491–498.


26. Afonso V, Champy R, Mitrovic D, Collin P, Lomri A. Reactive oxygen species and superoxide dismutases: role in joint diseases. Joint Bone Spine. 2007; 74:324–329.


27. Kensler TW. Chemoprevention by inducers of carcinogen detoxication enzymes. Environ Health Perspect. 1997; 105:Suppl 4. 965–970.


28. Prestera T, Talalay P, Alam J, Ahn YI, Lee PJ, Choi AM. Parallel induction of heme oxygenase-1 and chemoprotective phase 2 enzymes by electrophiles and antioxidants: regulation by upstream antioxidant-responsive elements (ARE). Mol Med. 1995; 1:827–837.


29. Ip SP, Ma CY, Che CT, Ko KM. Methylenedioxy group as determinant of schisandrin in enhancing hepatic mitochondrial glutathione in carbon tetrachloride-intoxicated mice. Biochem Pharmacol. 1997; 54:317–319.


30. Pan SY, Yu ZL, Dong H, Xiang CJ, Fong WF, Ko KM. Ethanol extract of fructus schisandrae decreases hepatic triglyceride level in mice fed with a high fat/cholesterol diet, with attention to acute toxicity. Evid Based Complement Alternat Med. 2011; 729412.


31. Ryu SN, Kim KS, Lee EB, Kang SS, Kim JS, Cheon SA, Lee BH. Acute toxicity of fruit pigment and seed oil of Schizandra chinensis in mice. J Korean Soc Int Agric. 1998; 10:37–41.
32. Lee JS, Lee SW. Effect of water extract in fruits of Omija (Schizandra chinensis Baillon) on CCl4 toxicity. Korean J Diet Cult. 1990; 5:253–257.