Abstract
BACKGROUND/OBJECTIVES
Overproduction of nitric oxide (NO) by the inducible nitric oxide synthase (iNOS) enzyme can cause inflammation. Cyclooxygenase-2 (COX-2) is also involved in the inflammatory response through regulation of nuclear factor-kappa B (NF-κB). Areca catechu is one of the known fruit plants of the Palmaceae family. It has been used for a long time as a source of herbal medicine in Indonesia. In this study, we explored the effect of Indonesian Areca catechu leaf ethanol extract (ACE) in lipopolysaccharide (LPS)-induced inflammation and carrageenan-induced paw edema models. Recently, this natural extract has been in the spotlight because of its efficacy and limited or no toxic side effects. However, the mechanism underlying its anti-inflammatory effect remains to be elucidated.
MATERIALS/METHODS
We measured NO production by using the Griess reagent, and determined the expression levels of inflammation-related proteins, such as iNOS, COX2, and NF-κB, by western blot. To confirm the effect of ACE in vivo, we used the carrageenan-induced paw edema model.
Health is highly influenced by diet because obesity increases the risks for immune system disorders [1]. The incidence of obesity has greatly increased in recent years owing to the consumption of processed foods and a high-fat diet, and this pattern is expected to increase over time. Hence, the use of Western medicine commonly used to treat obesity-related diseases is also increasing, as are the health risks among patients [2]. Alternative therapies such as Korean traditional medicine, which makes use of potent secondary metabolites from plants, is one of the oldest forms of treatment known to mankind [3]. Bioactive components isolated from diverse plants have provided a significant source of medicinal agents and abundant nutrients since ancient times [4]. Furthermore, the type of plant is important for the investigation of pharmacological properties because plants have different abilities to accumulate secondary metabolites [5].
Indonesia has an abundance of plant species because of its tropical climate that supports plant growth [6]. Areca catechu is one of the known fruit plants of the Palmaceae family [7]. It has been used for a long time as a source of herbal medicine to treat dysentery or dysuria in Indonesia. While the Areca catechu nut has been actively studied for its pharmacological properties, including anti-inflammatory effects, there is a lack of studies on the effect of Areca catechu leaves and the underlying molecular mechanism.
The immune system attempts to prevent inflammatory responses resulting from damaged cellular functions or is activated at the onset of microorganism infections [8]. Therefore, most chronic diseases, including vascular diseases, arthritis, cancer, and type II diabetes, involve inflammatory reactions [9]. Claria et al. reported that macrophages contributed to adipose tissue inflammation and phenotypic changes caused by obesity [10]. Inflammation is a complicated process, which is regulated by transcription factors, pro-inflammatory cytokines, adhesion enzymes, and other mediators [11].
Lipopolysaccharide (LPS), which is a major component of bacterial cell walls, activates immune cells and induces the production of inflammatory mediators such as nitric oxide (NO) [10]. The activation of inducible nitric oxide synthase (iNOS) and cyclooxygenase-2 (COX-2) can result in excessive NO production [13]. These enzymes are regulated by the nuclear factor-kappa B (NF-κB) transcription factor, which is activated and translocates to the nucleus in response to inflammatory stimuli [14]. LPS-stimulated RAW 264.7 cells and carrageenan-induced inflammation are widely used as chronic and acute inflammation models [15]. There is evidence that the immune response underlies the induction of iNOS and COX-2 [16]. Therefore, herbal medicines may act as anti-inflammatory agents by suppressing mediators such as NF-κB, iNOS, and COX-2.
In this study, we attempted to elucidate the anti-inflammatory effect of Areca catechu ethanol extract (ACE) on LPS-induced RAW 264.7 macrophages by measuring nitrate, NF-κB, iNOS, and COX-2 levels.We also investigated its anti-inflammatory effect in a carrageenan-mediated rat inflammation model to determine whether this herb has a potential medical benefit as an anti-inflammation agent.
The materials for cell culture were purchased from Gibco BRL (Gaithersburg, MD), and the EZ-cytox Cell Viability Assay Kit was obtained from Daeil Lab Service (Seoul, Korea). LPS (Escherichia coli O11:B4) and carrageenan were purchased from Sigma (St. Luis, MO). The COX-2 antibody was obtained from Cell Signaling Technology Inc. (Beverly, MA), and iNOS, NF-κB, IκB-α, and GAPDH antibodies were obtained from Santa Cruz (Santa Cruz, MA). All other reagents were purchased from Sigma.
Areca catechu was collected from Merauke, Indonesia. A voucher specimen was deposited at the Department of Pharmacology and Phytochemistry, Airlangga University, Indonesia. A total of 100 g of the leaves were ground using liquid nitrogen in a blender, and the crude powder was precipitated with 500 mL of 70% ethanol at room temperature for 3 days. The aqueous extracts were concentrated and evaporated at 60℃ under vacuum. The extract was dissolved in 50 mL of sterile deionized water and lyophilized by freezer drying at -60℃. The final yield was 0.12 g of powder from the plant leaves; the powder was subsequently used in experiments.
Murine RAW 264.7 macrophages (Korean Cell Line Bank, Seoul, Korea) were cultured in Dulbecco's Modified Eagle's Medium (DMEM) supplemented with 10% fetal bovine serum (FBS) and 1% penicillin-streptomycin in a humidified 5% CO2 atmosphere at 37℃. A total of 5 × 104 cells were seeded in a 96-well micro-plate and incubated with different concentrations of ACE (dissolved and diluted in DMEM) for 24 h. Cell viability was measured by enzyme-linked immunosorbent assay (ELISA) using the EZ-cytox Cell Viability Assay Kit according to the manufacturer's instructions.
A total of 5 × 104 cells seeded in a 96-well micro-plate were incubated with different concentrations of ACE (0.3-3 µg/ml) for 1 h. The medium was replaced with LPS-containing DMEM (1 µg/ml) with 10% FBS, and the cells were incubated for 24 h. To determine the total concentration of NO in the culture media, Griess reagent was added to 100 µl of supernatant at each treatment condition and the absorbance at 520 nm was measured with an ELISA reader.
Cell lysates from each sample (30 µg) were separated on 12% acrylamide gels. The proteins were transferred onto a polyvinylidene difluoride (PVDF) membrane (Amersham Pharmacia Biotech) at 4℃. To block non-specific protein binding, PVDF membranes were incubated in 5% skim milk overnight at 4℃. Subsequently, the membranes were washed three times with Tris-buffered saline containing 0.1% Tween 20 (TBS/T) for 10 min. Membranes were incubated with COX2, iNOS, NF-κB, IκB-α, or GAPDH antibodies at 1:1000 dilution. After incubation with the corresponding secondary antibodies, the membranes were analyzed with a chemiluminesce reaction kit (ECL Plus, Amersham Pharmacia Biotech). The relative intensities of the bands were visualized and analyzed with Image J Software.
Twelve male Sprague-Dawley rats weighing 200-250 g were purchased from Veterinaria Pharma, Indonesia. Prior to experiments, all animals were adapted to the new environment for one week. The rats were individually housed in stainless steel cages with ad libitum access to water and feed (AIN 93G formula). All the rats were kept in a controlled environment (room temperature, 24 ± 2℃; humidity, 40 ± 2%; and 12-h light/dark cycle). All experiments and animal care were conducted in conformity with the institutional guidelines of Airlangga University, Indonesia (100/TDC/ETIC/2013). To test the anti-inflammatory activity of ACE, the animals were divided into a control group and three experimental groups. Different concentrations of ACE (2.5, 5, and 10 mg/kg/day in normal saline) were administered orally for 7 days at a regular schedule. For the acute inflammatory assay, tests were carried out after 1% carrageenan (in normal saline) was injected into the right hind paw. Paw edema volume was measured with a plethysmometer (Ugo Basile, Italy) [17] 0, 1, 2, 3, 4, and 5 h after carrageenan injection. The increase in paw volume (% of control) was calculated based on the difference between the control and test groups.
We used the XTT (2,3-Bis-(2-Methoxy-4-Nitro-5-Sulfophenyl)-2H-Tetrazolium-5-Carboxanilide)assay to determine the effect of ACE on RAW 264.7 murine macrophage viability. The results indicated that ACE was not cytotoxic at concentrations between 0.3-3 µg/ml (Fig. 1A). Next, to test the effect of ACE on NO production in LPS-stimulated RAW 264.7 cells, we measured nitrate levels by using the Griess reagent assay. Cells were incubated with ACE (0.3-3 µg/ml) in LPS-containing DMEM (1 µg/ml) for 24 h. As shown in Fig. 1B, ACE attenuated NO production at non-cytotoxic concentrations. The inhibition of LPS-induced NO levels was approximately 45%, and 50% in response to 1, and 3 µg/ml ACE, respectively.
To investigate whether ACE can regulate LPS-induced NO production signaling in RAW 264.7 cells, we measured iNOS and COX-2 protein expression by immunoblot analysis. RAW 264.7 cells were cultured in the absence or presence of ACE (0.3-3 µg/ml) in DMEM containing 10% FBS for 1 h, followed by stimulation with LPS (1 µg/ml) for the next 24 h. As shown in Fig. 2, iNOS and COX-2 expression were induced by LPS. However, ACE treatment inhibited LPS-induced COX-2 protein expression in a dose-dependent manner (Fig. 2 B). Further, treatment with 3 µg/ml ACE significantly decreased iNOS expression by approximately 20% (Fig. 2 C). Therefore, the strongest inhibitory effect of ACE on iNOS and COX-2 expression occurred at a concentration of 3 µg/m1 ACE. Next, we determined the effect of ACE on NF-κB signaling in response to LPS stimulation. ACE (0.3-3 µg/ml) significantly reduced NF-κB expression while increasing IκB levels in a dose-dependent manner (Fig. 2 D, E).
To examine the effect of ACE on acute inflammation, paw edema volumes of the control and ACE-treated rats were compared after injection of 1% carrageenan in the right paw. Paw edema volumes were measured 1, 2, 3, 4, and 5 h after injection. Paw volumes increased over time by 22.3 ± 2%, 30 ± 7%, 43.6 ± 11%, 54 ± 20%, and 57 ± 20% at 1, 2, 3, 4, and 5 h, respectively, in rats treated with 2.5 mg/kg ACE. The paw volumes in the group treated with 5 mg/kg ACE were 22.6 ± 0.05%, 28 ± 4.3%, 35.3 ± 7.7%, 34 ± 5.7%, and 36.3 ± 3% at 1, 2, 3, 4, and 5 h, respectively, after carrageenan injection. However, treatment with a high concentration of ACE (10 mg/kg) significantly reduced paw volume in this group compared to the paw volumes in the other groups. Paw volume was 22.6 ± 5% at 1 h and increased to 27.3 ± 1.1% at 5 h. ACE treatment significantly inhibited carrageenan-induced paw edema in a dose-dependent manner (Fig. 3).
Areca catechu is a well-known herbal medicine. It has been used to treat gastrointestinal infections in Indonesia, and East and North Asian countries [18]. In this study, we demonstrated the inhibitory effects of ACE against inflammation in vitro and in vivo. We examined the effect of ACE on the major inflammation signaling pathways in LPS-activated RAW 264.7 macrophages. Moreover, we examined the effect of ACE in an acute inflammation model of carrageenan-induced paw edema in rats. We found that inhibition of iNOS and NF-κB signaling was involved in the anti-inflammatory effect of ACE. Our results clearly demonstrate that ACE has a powerful activity against chronic and acute inflammation.
Inflammation is a complicated process, which is regulated by pro-inflammatory cytokines and by the translocation of cytosolic NF-κB to the nucleus [11, 14, 19]. Macrophages are the early antagonists of pathogens in the body and play an important role during inflammation [20]. Inflammatory responses in macrophages induced by LPS or carrageenan include production of pro-inflammatory cytokines and increased expression of iNOS and COX-2 [13]. Therefore, we first focused on the LPS-induced NO production in the in vitro study. We found that ACE was not toxic at concentrations between 0.3 and 3 µg/ml, based on the post-treatment viability of RAW 264.7 cells; however, higher dosages, such as 10 µg/ml, were cytotoxic. In addition, we demonstrated that ACE significantly decreased NO production in LPS-induced RAW 264.7 cells. Therefore, ACE is a promising therapeutic agent against acute and chronic inflammation.
Overproduction of NO by iNOS can induce inflammatory diseases and COX-2 can also induce the expression of pro-inflammatory cytokines [16,21]. The activation of iNOS in macrophages and the resulting overproduction of NO can lead to inflammation, cancer, and autoimmune diseases. Therefore, we also investigated the changes in the levels of key proteins involved in inflammation by performing immunoblot analysis. Our results indicated that ACE treatment inhibited iNOS and COX-2 expression in LPS-stimulated RAW 264.7 cells. These results suggest that ACE inhibits the transcription of iNOS, leading to decrease NO production.
Notably, ACE inhibited inflammation by regulating NF-κB expression at low concentrations. The NF-κB transcription factor is a key regulator of immune and inflammatory responses [22]. In its inactive state, NF-κB is present in the cytoplasm in a complex with IκB [23]. Upon cell stimulation, IκB is phosphorylated and dissociates from NF-κB, which translocates to the nucleus and activates the transcription of inflammation-associated genes [24,25,26]. Our results showed that treatment with 1 and 3 µg/ml ACE significantly increased IκB expression, suggesting that ACE inhibited LPS-induced NF-κB expression by suppressing IκB phosphorylation.
Similarly, Huo et al. previously showed an anti-inflammatory effect of the natural compound linalool in LPS-stimulated RAW 264.7 cells [24]. Contrary to our results, Lin et al. (2005) reported that Areca nut extract, which is rich in polyphenols, induced COX-2 expression through the activation of the NF-κB signal transduction pathway [27]. However, Areca nut extract has also been shown to inhibit COX-2 expression [28]. This difference is probably due to an active component present in Areca nuts, and not leaves, which we used in our study.
Furthermore, we found the anti-inflammatory activity of ACE after carrageenan treatment caused irritation of sub-pleural tissues. The contents of exudates provide useful information on the anti-inflammatory effects of chemicals [29]. Sustained administration of ACE at 2.5-10 mg/kg/day for several days prevented carrageenan-induced inflammatory exudate formation. Our results indicated that treatment with different concentrations (2.5-10 mg/kg) of ACE decreased paw volume in the acute inflammation animal model. Treatment with a high dose (10 mg/kg) of ACE greatly decreased paw volumes and prevented further inflammation, which was consistent with the results of Barman et al. showing that treatment with Areca catechu leaves inhibited nociceptive activity in the acetic acid-induced gastric pain mouse model [30]. Therefore, Areca catechu leaves might contain phytochemicals similar to those found in the nuts, which might be the reason for the anti-inflammatory activity observed in this study.
In conclusion, we clearly demonstrated the anti-inflammatory effect of ACE in vitro and in vivo. Our results suggest that anti-inflammatory capacity of ACE is attributable not only to the suppression of iNOS and COX-2 but also to the phosphorylation of IκB. Although we confirmed the effect of ACE in the LPS-induced RAW 264.7 cell inflammatory response, the activation of other mechanisms and signaling pathways such as pro-inflammatory signals and the secretion of cytokines and related enzyme activity were not evaluated in this study. Hence, further studies are crucial for a broader molecular understanding of the anti-inflammatory effect of ACE.
Figures and Tables
Fig. 1
Effect of Areca catechu leaf ethanol extract (ACE) on cell viability and production of nitric oxide (NO) in lipopolysaccharide (LPS)-stimulated RAW 264.7 cells. (A) RAW 264.7 cells were treated with 0.3-10 µg/ml ACE for 24 h and cell viability was determined with the XTT assay. Values are expressed as a percentage of control. Each value represents the mean from three independent experiments ± SD. *indicates a significant difference compared with untreated controls (P < 0.05). (B) RAW 264.7 cells were treated with 0.3-3 µg/ml ACE and 1 µg/ml LPS for 24 h. NO production was determined with the Griess reagent assay. The values were determined and quantified from the sodium nitrate standard curve. The nitrate concentrations (µM) were determined in three independent experiments. Data were expressed as mean ± SD. *indicates a significant difference compared with LPS treatment only (P < 0.05).
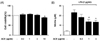
Fig. 2
Effect of Areca catechu leaf ethanol extract (ACE) on inducible nitric oxide synthase (iNOS), cyclooxygenase (COX)-2, inhibitor of kappa B (IκB), and nuclear factor-kappa B (NF-κB) expression in lipopolysaccharide (LPS)-stimulated RAW 264.7 cells. (A) RAW 264.7 cells were treated with 0.3-3 µg/ml ACE and 1 µg/ml LPS for 24 h. The levels of iNOS, COX-2, IκB, and NF-κB proteins were examined by immunoblot analysis. (B-E) The graphs show the band intensity from each immunoblot with specific antibodies. Value changes from three independent experiments are expressed relative to those in LPS-treated controls. *indicates a significant difference compared with LPS only treatment (P < 0.05).
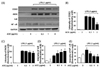
Fig. 3
Effect of Areca catechu leaf ethanol extract (ACE) on carrageenan (CR)-induced paw edema in rats. To test the effect of ACE (2.5, 5, or 10 mg/kg) on CR-induced paw edema, rats were divided into 4 groups. Water (control) and ACE (dissolved in 500 µl of normal saline) were administered orally during a 7-day regular schedule. To explore the anti-inflammatory effect of ACE in each group, 50 µl of 1% carrageenan was injected into the sub-plantar side of the hind paw of all animals. The paw edema volumes were recorded at 0, 1, 2, 3, 4, and 5 h post-injection. Values are expressed as the increase in paw edema volume (%).
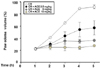
References
1. Bastard JP, Maachi M, Lagathu C, Kim MJ, Caron M, Vidal H, Capeau J, Feve B. Recent advances in the relationship between obesity, inflammation, and insulin resistance. Eur Cytokine Netw. 2006; 17:4–12.
3. Leem KH, Park HK. Traditional Korean medicine: now and the future. Neurol Res. 2007; 29:Suppl 1. S3–S4.


4. Wang X, Zhang A, Sun H. Future perspectives of Chinese medical formulae: chinmedomics as an effector. OMICS. 2012; 16:414–421.


5. Salatino A, Fernandes-Silva CC, Righi AA, Salatino ML. Propolis research and the chemistry of plant products. Nat Prod Rep. 2011; 28:925–936.


6. Trusheva B, Popova M, Koendhori EB, Tsvetkova I, Naydenski C, Bankova V. Indonesian propolis: chemical composition, biological activity and botanical origin. Nat Prod Res. 2011; 25:606–613.


7. Khan S, Mehmood MH, Ali AN, Ahmed FS, Dar A, Gilani AH. Studies on anti-inflammatory and analgesic activities of betel nut in rodents. J Ethnopharmacol. 2011; 135:654–661.


8. Broz P, Monack DM. Molecular mechanisms of inflammasome activation during microbial infections. Immunol Rev. 2011; 243:174–190.


9. Libby P. Inflammatory mechanisms: the molecular basis of inflammation and disease. Nutr Rev. 2007; 65:S140–S146.


10. Clària J, González-Périz A, López-Vicario C, Rius B, Titos E. New insights into the role of macrophages in adipose tissue inflammation and Fatty liver disease: modulation by endogenous omega-3 Fatty Acid-derived lipid mediators. Front Immunol. 2011; 2:49.


12. Li C, Wang MH. Anti-inflammatory effect of the water fraction from hawthorn fruit on LPS-stimulated RAW 264.7 cells. Nutr Res Pract. 2011; 5:101–106.


13. Ahn KS, Aggarwal BB. Transcription factor NF-kappaB: a sensor for smoke and stress signals. Ann N Y Acad Sci. 2005; 1056:218–233.
14. Park CM, Song YS. Luteolin and luteolin-7-O-glucoside inhibit lipopolysaccharide-induced inflammatory responses through modulation of NF-κB/AP-1/PI3K-Akt signaling cascades in RAW 264.7 cells. Nutr Res Pract. 2013; 7:423–429.


15. Halici Z, Dengiz GO, Odabasoglu F, Suleyman H, Cadirci E, Halici M. Amiodarone has anti-inflammatory and anti-oxidative properties: an experimental study in rats with carrageenan-induced paw edema. Eur J Pharmacol. 2007; 566:215–221.


16. Zamora R, Vodovotz Y, Billiar TR. Inducible nitric oxide synthase and inflammatory diseases. Mol Med. 2000; 6:347–373.


17. Fereidoni M, Ahmadiani A, Semnanian S, Javan M. An accurate and simple method for measurement of paw edema. J Pharmacol Toxicol Methods. 2000; 43:11–14.


18. Elliott S, Brimacombe J. The medicinal plants of Gunung Leuser National Park, Indonesia. J Ethnopharmacol. 1987; 19:285–317.


19. O'Neill LA, Kaltschmidt C. NF-kappa B: a crucial transcription factor for glial and neuronal cell function. Trends Neurosci. 1997; 20:252–258.
20. Gilroy DW, Lawrence T, Perretti M, Rossi AG. Inflammatory resolution: new opportunities for drug discovery. Nat Rev Drug Discov. 2004; 3:401–416.


21. Lee SH, Soyoola E, Chanmugam P, Hart S, Sun W, Zhong H, Liou S, Simmons D, Hwang D. Selective expression of mitogen-inducible cyclooxygenase in macrophages stimulated with lipopolysaccharide. J Biol Chem. 1992; 267:25934–25938.


22. Ghosh S, May MJ, Kopp EB. NF-kappa B and Rel proteins: evolutionarily conserved mediators of immune responses. Annu Rev Immunol. 1998; 16:225–260.


23. Gao Y, Jiang W, Dong C, Li C, Fu X, Min L, Tian J, Jin H, Shen J. Anti-inflammatory effects of sophocarpine in LPS-induced RAW 264.7 cells via NF-κB and MAPKs signaling pathways. Toxicol In Vitro. 2012; 26:1–6.


24. Huo M, Cui X, Xue J, Chi G, Gao R, Deng X, Guan S, Wei J, Soromou LW, Feng H, Wang D. Anti-inflammatory effects of linalool in RAW 264.7 macrophages and lipopolysaccharide-induced lung injury model. J Surg Res. 2013; 180:e47–e54.


25. Baeuerle PA, Baltimore D. NF-kappa B: ten years after. Cell. 1996; 87:13–20.
26. Baldwin AS Jr. The NF-kappa B and I kappa B proteins: new discoveries and insights. Annu Rev Immunol. 1996; 14:649–683.
27. Lin SC, Lu SY, Lee SY, Lin CY, Chen CH, Chang KW. Areca (betel) nut extract activates mitogen-activated protein kinases and NF-kappaB in oral keratinocytes. Int J Cancer. 2005; 116:526–535.


28. Huang PL, Chi CW, Liu TY. Effects of Areca catechu L. containing procyanidins on cyclooxygenase-2 expression in vitro and in vivo. Food Chem Toxicol. 2010; 48:306–313.


29. Vinegar R, Truax JF, Selph JL, Voelker FA. Pathway of onset, development, and decay of carrageenan pleurisy in the rat. Fed Proc. 1982; 41:2588–2595.
30. Barman MR, Uddin MS, Akhter S, Ahmed MN, Haque Z, Rahman S, Mostafa F, Zaman M, Noor FA, Rahmatullah M. Antinociceptive activity of methanol extract of Areca catechu L.(Arecaceae) stems and leaves in mice. Adv Nat Appl Sci. 2011; 5:223–226.