Abstract
BACKGROUND/OBJECTIVES
The traditional Korean diet is plant-based and rich in antioxidants. Previous studies have investigated the potential health benefits of individual nutrients of Korean foods. However, the cumulative effects of a Korean diet on inflammation remain poorly understood. Therefore, the aim of this study was to investigate the anti-inflammatory effects of a plant-based Korean diet.
MATERIALS/METHODS
Using data from the Fifth Korean National Health and Nutrition Examination Survey, 75 individual plant food items were selected which represent over 1% of the total diet intake of the Korean diet. These items were classified into ten different food groups, and the vegetable (Veg) and fruit (Fruit) groups were studied based on their high antioxidant capacity. For comparison, a mixture of all ten groups (Mix) was prepared. To produce a model of inflammation with which to test these Veg, Fruit, and Mix plant-based Korean food extracts (PKE), RAW264.7 macrophages were treated with lipopolysaccharide (LPS).
RESULTS
Levels of nitric oxide (NO) and prostaglandin E2 (PGE2), as well as protein expression of inducible nitric oxide synthase (iNOS) and cyclooxygenase-2 (COX-2) were found to be lower following PKE treatment. Furthermore, PKE treatment was found to suppress tumor necrosis factor-α (TNF-α) and interleukin-6 (IL-6) via the nuclear transcription factor kappa-B (NF-κB) signaling pathway. Overall, the Mix group exhibited the greatest anti-inflammatory effects compared with Veg and Fruit PKE group.
Inflammation is a major response to infection and is induced by several stimuli, including physical damage, microorganisms, and the immune response [1]. Accumulating evidence also indicates that chronic inflammatory processes can contribute to the development of various diseases, including cancer, type 2 diabetes, atherosclerosis, rheumatoid arthritis, and inflammatory bowel disease (IBD) [2,3]. Macrophages are innate immune cells which play a critical role in regulating various immunopathological conditions of the inflammatory process by releasing factors that mediate inflammation [4]. In particular, macrophages are activated by pro-inflammatory substances such as the endoxocin, lipopolysaccharide (LPS), which is a structural component of the outer wall of gram-negative bacteria. Following stimulation by LPS, macrophages produce inflammatory mediators via the activation of several intracellular signaling pathway, including nuclear factor-κB (NF-κB) pathway [5]. This reaction initiates amplification of the inflammatory responses and is essential for the defense system of a host [6].
NF-κB is a transcription factor that plays a critical role in the overproduction of inflammatory mediators by macrophages in response to LPS [7]. For example, in resting cells, NF-κB is retained in the cytosol by inhibitory κB (IκB), which blocks the nuclear localization signal of NF-κB [8]. However, when IκB is phosphorylated in response to various stimuli and is subsequently degraded by the proteosome, NF-κB (p50-p65) heterodimers can translocate to the nucleus and activate gene expression. In particular, cyclooxygenase-2 (COX-2) and inducible nitric oxide synthase (iNOS) are activated with the latter associated with cell damage, organ damage, enhance blood vessel expansion, and the inflammatory response by macrophages [9]. In addition, interferon-gamma (IFN-γ) is sequentially induced by iNOS, resulting in the modulation of macrophage activation and differentiation in combination with LPS [10]. Other pro-inflammatory mediators are also triggered by NF-κB activation, and these include interleukin-6 (IL-6), tumor necrosis factor α (TNF-α), and prostaglandin E2 (PGE2) [11]. Synthesis of PGE2 is caused by up-regulation of COX-2, which also mediates various inflammatory processes [12]. Therefore, treatments that provide targeted inhibition of NF-κB signaling may be therapeutic for various inflammatory diseases [13].
There are several natural antioxidant polyphenol compounds and carotenoids that have been shown to directly inhibit the expression of NF-κB-dependent pro-inflammatory mediators, including iNOS, COX-2, IL-6, and TNF-α [10,14]. In particular, both in vitro and in vivo studies have demonstrated the anti-inflammatory effects of plant-based bioactive components such as quercetin, kaempferol, and epigallocatechin-gallate (EGCG) from flavonols, and naringenin from flavones [15,16]. In addition, it has been reported that carotenoids (β-carotene and lycopene) are able to inhibit the activation of NF-κB signaling [17]. Thus, dietary plant extracts possess anti-oxidative and radical scavenging activities, and these can play an important role in the absorption and neutralization of free radicals [18]. Correspondingly, the suppressive effects of these antioxidant compounds on the production of inflammatory mediators are associated with their antioxidant activities, including the inhibitory capacity of eicosanoid generating enzymes [19].
The traditional Korean diet contains few animal-derived foods and many types of vegetables [20]. This vegetable-rich culture remains dominant in Korea, and this is hypothesized to positively affect many chronic diseases [21]. Correspondingly, several epidemiological studies have reported that a traditional Korean dietary pattern is associated with a lower prevalence of chronic diseases, infectious diseases, metabolic syndromes, obesity, coronary heart disease, and cancer compared to Western dietary pattern [22,23]. Furthermore, it has been suggested that the anti-oxidant and/or anti-inflammatory potential of the Korean diet is due to the abundance of phytochemicals, vitamins, and carotenoids that are present in plant-derived foods. Although previous anti-inflammatory studies of the Korean diet have focused on a single nutrient or food, including Kim-chi, soybean paste, and capsicum paste [24,25], the anti-inflammatory effects of a Korean diet overall remains poorly understood.
Therefore, in the present study, extracts of the plant foods that are commonly consumed in plant-based Korean diet were prepared in order to evaluate the anti-inflammatory effects of these foods, as well as the effects of these foods on the NF-κB pathway and related inflammatory mediators that are produced in response to LPS in mouse macrophage cell line, RAW264.7.
The plant food intakes of the Korean diet were estimated using raw data from the Korea National Health and Nutrition Examination Survey (KNHANES V, 2009-2011) [26]. The KNHANES (2009) was a nationwide representative study of a total of 8,027 subjects that were older than 1 year of age. Seventy-five individual plant-based food items were identified that represented over 1% of the total food intake of the typical Korean diet. These food items represent the dietary intakes those were estimated per person, per day of each food item examined from the average Korean diet. The individual foods were purchased at different local supermarkets and were grouped into ten food groups as follows: cereals, potatoes, legumes, vegetables, kimchies, mushrooms, fruits, nuts, seaweeds, and oils. To represent the total per capita daily intake of foods in the Korean diet, the following categories with their total weights were established: cereals (total: 238.4 g), vegetables (total: 175.0 g), legumes (total: 34.5 g), potatoes (total: 33.0 g), kimchies (total: 100.9 g), mushrooms (total: 4.9 g), nuts (total: 2.7 g), fruits (total: 165.6 g), and seaweeds (total: 11.7 g). From these categories, samples were freeze-dried, ground, and stored in duplicate prior to analysis. Vegetable oils (total: 5.5 g) were also analyzed individually and included soybean oil, sesame seed oil, wild sesame seed oil, rape seed oil, and corn oil.
Among the groups, a total mixture of ten groups (Mix), a vegetable group (Veg), and a fruits group (Fruit) were high in diphenylpicrylhydrazyl (DPPH) radical scavenging activity (unpublished data). Therefore, we used these three groups for the experiments in the present study. Eighteen types of vegetables, including onions, red peppers, tomatoes, cucumbers, green onions, Chinese cabbage, soybean sprouts, squash, radish leaves, radishes, lettuce, spinach, carrots, sesame leaf, garlic, bracken, cabbage, and leeks were included in the Veg PKE. The Fruit PKE contained ten fruits, including apples, citrus fruits, pears, water melons, grapes, persimmons, muskmelons, strawberries, peaches, and Japanese apricot. The food items that were included in the Mix were reported by Lee at al. [27]. Samples were prepared according to the total daily per capita intake of solid plant food that has been reported for the Korean diet.
To prepare extracts of each group, one gram of each ground plant food sample was placed in a test tube with 40 ml methanol/water (50:50, v/v), and the pH was adjusted to 2.0 using 2 N HCl. The tubes were then thoroughly shaken at room temperature for 1 h and centrifuged at 2500 × g for 10 min. Supernatants were subsequently collected and mixed with 40 ml acetone/water (70:30, v/v), and shaken for another hour. After centrifugation at 2500 × g for 10 min, both methanol and acetone extracts were combined. The mixed solution for each sample was stored at -80℃ until they needed.
The RAW264.7 macrophage cell line was purchased from the Korean Cell Line Bank (Seoul, Korea) and cultured with Dulbecco's Modified Eagle's Medium (DMEM) (Welgene, Daegu, Korea). The medium used for subculturing was supplemented with 10% fetal bovine serum (FBS) (v/v, Hyclone, Logan, UT) and 1% penicillin-streptomycin (100 U/ml and 100 µg/ml, respectively; Invitrogen, Carlsbad, CA). Cells were maintained 5% CO2 and 37℃. Cells were stimulated with 1 µg/ml LPS (Sigma, St. Louis, MO) and treated with various doses of PKE.
The cell viability of RAW264.7 cells treated with PKE was assessed using trypan blue exclusion assays. Briefly, cells were seeded in 12-well plates (4.5 × 105 cells/well) and incubated at 37℃ and 5% CO2 for 24 h. Cells were then treated with various doses of PKE for 24 h, then cells were trypsinized and stained with 0.4% trypan blue solution (Invitrogen, Carlsbad, CA) at a 1:1 dilution. Stained and unstained cells were counted in three independent experiments for each treatment using a hemocytometer (Marienfeld, Germany), and the proportion of surviving cells was calculated.
NO production was detected by measuring the concentration of nitrite present in culture medium was described previously [28]. Briefly, RAW264.7 cells in 10% FBS-DMEM without phenol red were plated in 24-well plates (3×105 cells/ml). Cells were pretreated with 0, 2, 4, or 8 mg/ml PKE for 30 min, then were treated with LPS (1 µg/ml) or LPS (100 ng/ml) + IFN-γ (10 ng/ml) for 24 h. Nitrate levels were measured in cell culture supernatants using the Greiss reagent (1% sulfanilamide, 0.1% N-1-naphthylethylenediamine dihydrochloride, 5% H3PO4 solution), and these levels were compared with a nitrate standard curve. An equal volume (100 µl) of cultured supernatant and Griess reagent was gently mixed and incubated in the dark for 15 min. Absorbance values at 560 nm were recorded using a microplate reader (Molecular Devices, Sunnyvale, CA).
RAW264.7 cells were pretreated with PKE (0, 2, 4, or 8 mg/ml) for 30 min, then were incubated with or without LPS (1 µg/ml) for 24 h. PGE2 production was measured using a Parameter assay kit (R&D Systems, Minneapolis, MN), and the production of IL-6 or TNF-α were measured using ELISA kits (R&D Systems, Minneapolis, MN) according to the manufacturer's instructions. Results from three independent experiments were used for statistical analyses.
Cytosolic and nuclear extracts were prepared as described previously [29,30]. Briefly, for cytosolic extracts, cells were pretreated with 0, 2, 4, or 8 mg/ml PKE for 30 min, then were treated with or without LPS (1 µg/ml) for 24 h. Protein lysates were obtained using lysis buffer [50 mM Tris-Cl (pH 7.5), 1% Nonidet P-40, 150 mM NaCl, 0.5% sodium deoxycholate, 0.1% SDS, 1 mM PMSF, 1 mM Na3VO4, 1 mM NaF]. Lysates were centrifuged at 13,000 rpm for 15 min, then supernatants were collected and stored at -80℃.
For nuclear extracts, cells were washed twice with cold PBS, were scraped from plates using cold PBS, and were pelleted by centrifugation. The cell pellets were resuspended gently in hypotonic buffer [buffer A: 4 M NaCl, 10 mM HEPES (pH 7.9), 10 mM NaF, 0.1 mM Na3VO4, 500 mM EDTA, 1 mM PMSF, 0.05 mM dithiothreitol (DTT), 10 µg/ml aprotinin, pepstatin 1 mg/ml, leupeptin 1 mg/ml], then were incubated on ice for 10 min. The cells were subsequently lysed with the addition of 0.05% NP-40 and vortexing for 1 min. After being centrifuged at 13,000 rpm for 10 min at 4℃, resulting cell pellets were resuspended in hypertonic buffer [buffer C : 20 mM HEPES (pH 7.9), 400 mM NaCl, 1.5 mM MgCl2, 0.2 mM EDTA, 0.5 mM DTT, 0.5 mM PMSF, 25% (v/v) glycerol, 1 M benzamidine], then were incubated on ice for 20 min. Cells were centrifuged again at 13,000 rpm for 3 min at 4℃, and the supernatants were stored at -80℃.
Western blotting assays were performed as previously described [28]. Briefly, nuclear or cytosolic protein concentrations were determined using a Bradford protein assay (BioRad, Hercules, CA). For detection of iNOS (Santa Cruz, Santa Cruz, CA), COX-2 (Becton Dickinson, Bedford, MA), NF-κB (Cell Signaling, Danvers, MA), p-IκBα (Cell Signaling, Danvers, MA), Lamin A/C (Cell Signaling, Danvers, MA), and α-tubulin (Sigma, St. Louis, MO), total cellular protein (100 µg) was separated using 8-12% acrylamide sodium dodecyl sulfatepolyacrylamide gel electrophoresis (SDS-PAGE). Proteins were transferred to polyvinylidene difluoride (PVDF) membranes (Millipore, Billerica, MA). The membranes were washed three times with Tris-buffered saline containing Tween-20 (TBST) and then were incubated with blocking solution (5% skim milk or 5% BSA) for 1 h. The membranes were incubated overnight at 4℃ with primary antibodies and exposed to horseradish peroxidase-labeled secondary antibodies (Santa Cruz, Santa Cruz, CA) for 1 h at room temperature. Bound antibodies were visualized using enhanced chemiluminescence reagents (Animal Genetics Inc, Suwon, Gyeonggi-do, South Korea). Lamin A/C and α-tubulin were used as loading controls for nuclear and cytosolic proteins, respectively.
Results are presented as the mean ± standard error of the mean (SEM) for at least three independent experiments and were analyzed using GraphPad PRISM 3.0 software (GraphPad Software, Inc., SanDiego, CA). The significance of the results were assessed using one-way analysis of variance (ANOVA) followed by Tukey's post-hoc test. A P-value less than 0.05 was considered statistically significant.
Many foods that exhibit antioxidant properties also mediate anti-inflammatory effects when they are consumed together. For example, Veg and Fruit groups exhibited higher levels of antioxidant activity than the other food groups assayed, which included cereal, potatoes, legumes, kimchies, mushrooms, nuts, and oils (unpublished data). Consequently, Veg and Fruit groups were further compared with Mix group that included a combination of all ten food groups. For these three groups (Mix, Veg, Fruit), PKE was generated and was used to treat RAW264.7 cells. In these assays, the cell viability of RAW264.7 cells treated with each of the three PKEs was not affected (Supplementary Fig. 1).
It is widely known that iNOS produced by macrophages is responsible for the overproduction of NO during the inflammatory process [31]. To investigate whether PKE affect induction of NO in RAW264.7 cells in response to LPS, production of NO was detected following the treatment of RAW264.7 cells with various concentrations of Mix, Veg, and Fruit PKE. As shown in Fig. 1, the treatment of cells with LPS (1 µg/ml) resulted in a 5-fold increase in NO levels in culture medium. In contrast, pretreatment of cells with Mix, Veg, and Fruit PKE significantly decreased LPS-induced NO production in a dose-dependent manner. A comparison of all three PKE at a concentration of 8 mg/ml also showed that inhibition of NO production was the highest for the Mix group (Fig. 1D).
To further elucidate the mechanism by which PKE inhibits NO production by LPS-induced macrophages, iNOS expression was also assayed for RAW264.7 cells treated with Mix, Veg, and Fruit PKE (Fig. 2). Under normal conditions, macrophage express undetectable levels of iNOS protein expression, and these levels markedly increase following LPS treatment. However, following the treatment of RAW264.7 cells with Mix, Veg, and Fruit PKE, down-regulation of iNOS expression was observed after each treatment, and the greatest inhibition was achieved with application of Mix PKE. These results indicate that the inhibition of NO production by PKE tested involves down-regulation of iNOS.
IFN-γ is a cytokine that also plays an important role in macrophage activation and differentiation, and is induced by iNOS in response to LPS [10]. Therefore, RAW264.7 macrophages were individually activated with LPS+IFN-γ, and then were treated with Mix, Veg, and Fruit PKE. Although a significant increase in NO production was observed following LPS+IFN-γ treatment, NO levels were significantly suppressed following treatment with Mix and Veg PKE in a dose-dependent manner (Fig. 3A, B). Furthermore, the pretreatment of cells with 8 mg/ml Mix PKE was found to significantly inhibit the induction of NO by LPS+IFN-γ by 78%. The inhibitory effect of Fruit was slightly less than that of either the Mix or Veg PKE, yet was still statistically significant at all three concentrations assayed (Fig. 3C). In addition, when all three PKE groups were used at 8 mg/ml, cells were treated with Mix PKE exhibited the highest efficacy for NO inhibition (Fig. 3D), while cells treated with Veg PKE were second.
It is well-known that PGE2 is produced by activated macrophages via induction of COX-2 [19]. To identify the potential anti-inflammatory properties of the Mix, Veg, and Fruit PKE investigated in this study, levels of the pro-inflammatory mediators, PGE2 and COX-2 were analyzed following the treatment of activated macrophages with each PKE. While PGE2 levels were undetectable in un-stimulated macrophages, PGE2 levels increased dramatically following treatment with LPS (Fig. 4). When RAW264.7 cells were treated with Mix, Veg, or Fruit PKE in the presence of LPS, the levels of PGE2 decreased for each PKE, although only the 4 mg/ml and 8 mg/mL doses resulted in a significantly decrease in Veg and Fruit group (Fig. 4A). In addition, treatment with 8 mg/ml Mix PKE decreased PGE2 production by 55.7%. When all three PKE groups were used at 8 mg/ml, they all suppressed LPS-induced PGE2 production substantially (Fig. 4D).
To determine the mechanism by which PKE reduces LPS-induced PGE2 production, COX-2 expression was analyzed. While LPS treatment was found to markedly up-regulate expression of COX-2, levels of COX-2 were suppressed following treatment with all three groups PKE groups (Fig. 5). These results suggest that the PKE tested are able to suppress COX-2 expression, and this corresponds with the dramatic decrease in levels of PGE2 that were also observed.
TNF-α is one of the most important pro-inflammatory cytokines and induces the secretion of IL-6 [32]. For IL-6, elevated levels induced by LPS were suppressed following treatment with all three PKE groups (Fig. 6). In particular, Mix PKE reduced IL-6 production in a dose-dependent manner. However, only the 4 mg/ml and 8 mg/ml concentrations of Veg significantly suppressed IL-6 production (Fig. 6B), while the Fruit exhibited a slight, yet significant, inhibition of IL-6 production at all three concentrations applied (2, 4, and 8 mg/ml) (Fig. 6C). When all three PKE groups were compared, attenuation of IL-6 production was most evident for the Mix PKE group (Fig. 6D).
As shown in Fig. 7A, the LPS-induced release of TNF-α decreased significantly following treatment with all three concentrations of Mix PKE. In particular, application of 8 mg/ml Mix resulted in an 81% decrease in TNF-α production. Similarly, the Fruit was associated with an inhibition of TNF-α production at all three doses (Fig. 7C). In contrast, the Veg only inhibited TNF-α production at a concentration of 8 mg/ml (Fig. 7B). A comparison of all three PKE groups showed that Mix induced the greatest inhibition of TNF-α production, while the Veg and Fruit PKE exhibited similar levels of inhibition (Fig. 7D). Taken together, these data suggest that the PKE tested were able to inhibit the release of the pro-inflammatory mediators, IL-6 and TNF-α.
NF-κB is a key transcription factor which regulates the production of pro-inflammatory mediators in LPS-induced macrophages. Activation of NF-κB is also required for the expression of iNOS, COX-2, and TNF-α [7]. To clarify the mechanism by which PKE could inhibit LPS-induced production of NO and TNF-α, the effects of PKE on LPS-induced NF-κB was analyzed by detecting p65 subunit of NF-κB. Degradation of phosphorylated IkBα and translocation of p65 from the cytoplasm to the nucleus were also analyzed (Fig. 8).
Upon LPS induction, levels of phosphorylated IkBα and nuclear p65 markedly increased. However, both of these levels decreased following treatment with Mix, Veg, and Fruit PKE. Although the treatment of Fruit and Veg groups had inhibitory effect, Mix treatment showed the most significant suppression of phosphorylated IkBα and nuclear p65. These data indicate that the PKE tested were able to inhibit the expression of pro-inflammatory mediators by regulating the NF-κB pathway in LPS-induced RAW264.7 macrophages.
The Korean diet is typically low in fat and rich in whole grains, fruits, and vegetables. As such, this dietary pattern would be predicted to be beneficial for inflammatory chronic diseases [33]. To determine whether plant-based foods in the Korean diet mediate anti-inflammatory effects, a macrophage model was used to evaluate the production of various inflammatory mediators and cytokines in response to treatment with prepared food extracts. To identify the PKE to be tested, the KNHANES V (2009-2011) data were used to identify individual food items that represented more than 1% of the total food intake of a Korean diet. As a result, the anti-inflammatory effects of Mix, Veg, and Fruit PKE on LPS-induced RAW264.7 macrophage were examined.
It is widely known that LPS, a component of the cell envelope of gram-negative bacteria, is recognized by toll-like receptor 4 (TLR4) on cell membranes and triggers an acute and early release of pro-inflammatory mediators and responses [34]. Moreover, the excessive production of various cytokines has been found to be responsible for many of the clinical disorders associated with immune diseases such as rheumatoid arthritis, inflammatory bowel disease, autoimmune disease, and septic shock [35,36]. Thus, inhibitors of the production of these cytokines and inflammatory mediators have been regarded as targets for the development of anti-inflammatory drugs. In the present study, pre-treatment with PKE was found to reduce production of NO, PGE2, COX-2, iNOS, IL-6, and TNF-α via NF-κB. The pro-inflammatory mediators, NO and PGE2 have been shown to play critical roles in inflammatory diseases. For example, NO is produced by iNOS and can generate intracellular signals that affect the function of both immune cells and tumor cells [37]. In the present study, all three of the PKE tested were found to significantly suppress NO production by LPS-induced macrophages. Similarly, PKE treatment was associated with a significant decrease in levels of PGE2, a factor which mediates inflammatory responses and is generated by the sequential enzymatic reaction of arachidonic acid by cyclooxygenases, COX-1 and COX-2 [38]. Moreover, the promoter region of COX-2 contains binding sites for various transcription factors, including NF-κB [39]. Thus, it is possible that COX-2 is modulated by various cytokines that regulate the immune response.
Pro-inflammatory cytokines such as TNF-α, IL-1β, and IL-6 are known to be modulated by numerous inflammatory cytokines. In addition, these cytokines can mediate tissue damage and organ failure in humans [40]. The results of a previous study suggest that TNF-α play a key role in some types of autoimmune pulmonary inflammation in lupus-prone mice [41]. Moreover, TNF-α has been found to elicit physiological effects such as septic shock, inflammation, cachexia, and cytotoxicity [42]. IL-6 is also a pivotal pro-inflammatory cytokine that is regarded as an endogeneous mediator of LPS-induced fever [43]. In the present study, pre-treatment of RAW264.7 macrophage with the Mix, Veg, and Fruit PEKs tested led to an inhibition of TNF-α and IL-6 production. While the Veg and Fruit only exhibited a slightly inhibitory effect, the Mix PKE showed significant suppression of IL-6 production. Similarly, the Mix was associated with the strongest inhibition of TNF-α production. In combination, these results suggest that the anti-inflammatory activity of the PKE tested involve reducing secretion of IL-6 and TNF-α, and this could help regulate inflammatory diseases.
NF-κB is a critical regulator of numerous genes which are involved in immune and inflammation responses [44]. Generally, when cells are activated by inflammatory stimuli, the inhibitory protein of NF-κB is degraded and NF-κB translocates into nucleus to promote transcription of pro-inflammatory mediators [45]. It has been reported that several natural antioxidants, including carotenoids and polyphenol compounds such as β-carotene, lycopene, lutein, astaxanthin, curcumin, and the aflavin mediate anti-inflammatory activities by directly inhibiting the expression of the NF-κB dependent cytokines, iNOS and COX-2 [45,46]. In the present study, the effects of the PKE generated on NF-κB activation were investigated in order to confirm the role of PKE in NF-κB signaling pathway. It was observed that accumulation of NF-κB in the nucleus was reduced followed PKE pre-treatment, despite activation by LPS. Therefore, we hypothesize that the antioxidant activity of the PKE tested inhibited the release of pro-inflammatory mediators by preventing the phsophorylation and degradation of IκBα in RAW264.7 macrophages. Consequently, activation of NF-κB p65 subunit was suppressed.
In the present study, the ability of the Mix, Veg, and Fruit PKE to inhibit the production of inflammatory mediators by LPS-induced RAW264.7 macrophages was compared. Overall, Mix PKE was consistently associated with the greatest reduction in LPS-induced, pro-inflammatory cytokines production. This observation is consistent with previous studies which demonstrated the synergistic effect of combined nutrients. For example, the combined antioxidant properties (e.g., scavenging capacity) of lycopene, vitamin E, vitamin C, and β-carotene were found to be substantially superior to that of each nutrient [47]. Correspondingly, the potent antioxidant and anticancer activities observed for the PKE tested are attributed to the additive and synergistic effects of phytochemicals present in plant-based foods [48]. Similarly, the antioxidant capacity of a Mediterranean diet is attributed to the favorable effect of mixing different foods together [49]. Therefore, a Korean diet, which includes the combination of several plant-based and antioxidant-rich side dishes within a single meal, has the potential to provide additive, anti-inflammation effects.
In conclusion, the PKE examined were found to exhibit anti-inflammatory activities, that were related to the suppression of NO, PGE2, IL-6, and TNF-α, as well as expression of COX-2 and iNOS via suppression of the NF-κB signaling pathway, in LPS-induced RAW264.7 macrophages. In particular, the anti-inflammatory efficacy of the PKE tested were maximized when macrophage were pre-treated with Mix PKE, an extract containing a mixture often plant-based food groups. Taken together, these results suggest that PKE can help attenuate inflammation mediated by activated macrophage, thereby potentially improving a host's response to inflammation-mediated diseases.
Figures and Tables
Fig. 1
PKE inhibits production of NO in LPS-induced RAW264.7 macrophages. Cells were pretreated with each PKE for 30 min, then were incubated with LPS. After 24 h, the culture medium was collected and analyzed for NO using Griess reagent. Three independent experiments were performed and the data shown represent the mean ± SEM for absrobances recorded at 560 nm. One-way ANOVA was implemented using Tukey's post-hoc test (α = 0.05). Data for Mix PKE (A), Veg PKE (B), Fruit PKE (C), and all three PKE at 8 mg/ml (D) are shown. Mix, an extract containing all ten food groups; Veg, vegetable PKE; Fruit, fruit PKE.
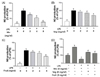
Fig. 2
PKE down-regulates iNOS expression in LPS-induced RAW264.7 macrophages. Cells were treated with various doses of each PKE for 24 h and were incubated with LPS for 30 min. Expression of iNOS in RAW264.7 cells was assayed using western blotting with α-tubulin used as a loading control. Data for Mix PKE (A), Veg PKE (B), Fruit PKE (C) are shown. Mix, an extract containing all ten food groups; Veg, vegetable PKE; Fruit, fruit PKE.
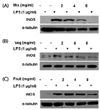
Fig. 3
PKE inhibits NO production in LPS/IFN-γ-induced RAW264.7 macrophages. Cells were pretreated with each PKE for 30 min and were incubated with LPS + IFN-γ. After 24 h, the culture medium was collected and assayed for NO production using Griess reagent. Three independent experiments were performed and the data shown represent the mean ± SEM for absorbances recorded at 560 nm. One-way ANOVA was implemented using Tukey's post-hoc test (α = 0.05). Data for Mix PKE (A), Veg PKE (B), Fruit PKE (C), and all three PKE at 8 mg/ml (D) are shown. Mix, an extract containing all ten food groups; Veg, vegetable PKE; Fruit, fruit PKE.
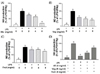
Fig. 4
PKE inhibits PGE2 production in LPS-induced RAW264.7 macrophages. Cells were treated with each PKE (2, 4, or 8 mg/ml) in 24-well plates for 30 min, then were stimulated with LPS. After 24 h, cell-free culture medium was collected and analyzed for levels of PGE2. Absorbance values were measured at 450 nm and three independent experiments were performed. Data represent the mean ± SEM, and different letters indicate significant differences (n = 3). One-way ANOVA was implemented using Tukey's post-hoc test (α = 0.05). Mix PKE (A), Veg PKE (B), Fruit PKE (C), and all three PKEs at 8 mg/ml (D) are shown. Mix, an extract containing all ten food groups; Veg, vegetable PKE; Fruit, fruit PKE.
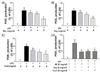
Fig. 5
PKE down-regulates expression of COX-2 in LPS-induced RAW264.7 macrophages. Cells were pretreated with various doses of each PKE for 30 min and were incubated with LPS. After 24 h, levels of COX-2 were analyzed using western blotting with α-tubulin used as a loading control. Mix PKE (A), Veg PKE (B), and Fruit PKE (C) are shown. Mix, an extract containing all ten food groups; Veg, vegetable PKE; Fruit, fruit PKE.
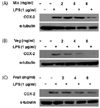
Fig. 6
PKE inhibits IL-6 production in LPS-induced RAW264.7 macrophages. Cells were pretreated with each PKE (2, 4, or 8 mg/ml) in 24-well plates for 30 min, then were stimulated with LPS. After 24 h, cell-free culture medium was collected and analyzed using an IL-6 ELISA kit. Absorbances were measured at 450 nm, and three independent experiments were performed. Data represent the mean ± SEM. One-way ANOVA was implemented using Tukey's post-hoc test (α = 0.05). Mix PKE (A), Veg PKE (B), Fruit PKE (C), and all three PKEs at 8 mg/ml (D) are shown. Mix, an extract containing all ten food groups; Veg, vegetable PKE; Fruit, fruit PKE.
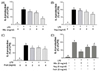
Fig. 7
PKE inhibits TNF-α production in LPS-induced RAW264.7 macrophages. Cells were pretreated with each PKE (2, 4, or 8 mg/ml) in 24-well plates for 30 min, then stimulated with LPS. After 24 h, cell-free culture medium was collected and analyzed using a TNF-α ELISA kit. Absorbance values were at 450 nm recorded for three independent experiments. Data represent the mean ± SEM. One-way ANOVA was implemented using Tukey's post-hoc test (α = 0.05). Mix PKE (A), Veg PKE (B), Fruit PKE (C), and all three PKE at 8 mg/ml (D) are shown. Mix, an extract containing all ten food groups; Veg, vegetable PKE; Fruit, fruit PKE.
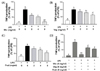
Fig. 8
PKE inhibits IκBα phosphorylation and translocation of the NF-κB p65 subunit in LPS-induced RAW264.7 macrophages. Cells were treated with various doses of each PKE for 30 min and were incubated with LPS for 24 h. Levels of cytosolic p-IκBα and nuclear NF-κB p65 were analyzed using western blotting. Lamin A/C and α-tubulin were used as loading controls for nuclear and cytosolic proteins, respectively. Mix PKE (A), Veg PKE (B), Fruit PKE (C), and all three PKE at 8 mg/ml (D) are shown. Mix, an extract containing all ten food groups; Veg, vegetable PKE; Fruit, fruit PKE.
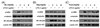
References
1. Rahman I, Biswas SK, Kirkham PA. Regulation of inflammation and redox signaling by dietary polyphenols. Biochem Pharmacol. 2006; 72:1439–1452.


2. Chiodoni C, Colombo MP, Sangaletti S. Matricellular proteins: from homeostasis to inflammation, cancer, and metastasis. Cancer Metastasis Rev. 2010; 29:295–307.


3. Hotamisligil GS, Erbay E. Nutrient sensing and inflammation in metabolic diseases. Nat Rev Immunol. 2008; 8:923–934.


4. Mollace V, Colasanti M, Muscoli C, Lauro GM, Iannone M, Rotiroti D, Nistico G. The effect of nitric oxide on cytokine-induced release of PGE2 by human cultured astroglial cells. Br J Pharmacol. 1998; 124:742–746.


5. Chen CH, Sheu MT, Chen TF, Wang YC, Hou WC, Liu DZ, Chung TC, Liang YC. Suppression of endotoxin-induced proinflammatory responses by citrus pectin through blocking LPS signaling pathways. Biochem Pharmacol. 2006; 72:1001–1009.


6. Pison U, Max M, Neuendank A, Weissbach S, Pietschmann S. Host defence capacities of pulmonary surfactant: evidence for 'non-surfactant' functions of the surfactant system. Eur J Clin Invest. 1994; 24:586–599.


7. Shapira L, Soskolne WA, Houri Y, Barak V, Halabi A, Stabholz A. Protection against endotoxic shock and lipopolysaccharide-induced local inflammation by tetracycline: correlation with inhibition of cytokine secretion. Infect Immun. 1996; 64:825–828.


8. Zhang T, Sun L, Liu R, Zhang D, Lan X, Huang C, Xin W, Wang C, Zhang D, Du G. A novel naturally occurring salicylic acid analogue acts as an anti-inflammatory agent by inhibiting nuclear factor-kappaB activity in RAW264.7 macrophages. Mol Pharm. 2012; 9:671–677.


9. Verma IM. Nuclear factor (NF)-kappaB proteins: therapeutic targets. Ann Rheum Dis. 2004; 63:Suppl 2. ii57–ii61.
10. Chan ED, Riches DW. IFN-gamma + LPS induction of iNOS is modulated by ERK, JNK/SAPK, and p38 (mapk) in a mouse macrophage cell line. Am J Physiol Cell Physiol. 2001; 280:C441–C450.
11. Brodsky M, Halpert G, Albeck M, Sredni B. The anti-inflammatory effects of the tellurium redox modulating compound, AS101, are associated with regulation of NFkappaB signaling pathway and nitric oxide induction in macrophages. J Inflamm (Lond). 2010; 7:3.
12. Pettus BJ, Bielawski J, Porcelli AM, Reames DL, Johnson KR, Morrow J, Chalfant CE, Obeid LM, Hannun YA. The sphingosine kinase 1/sphingosine-1-phosphate pathway mediates COX-2 induction and PGE2 production in response to TNF-alpha. FASEB J. 2003; 17:1411–1421.


13. Bacher S, Schmitz ML. The NF-kappaB pathway as a potential target for autoimmune disease therapy. Curr Pharm Des. 2004; 10:2827–2837.
14. Brodsky M, Duran F, Sanhueza J, Giaccone J II. Hemodynamic study of pulmonary arterial hypertension. Rev Med Chil. 1956; 84:7–16.
15. Chanput W, Mes J, Vreeburg RA, Savelkoul HF, Wichers HJ. Transcription profiles of LPS-stimulated THP-1 monocytes and macrophages: a tool to study inflammation modulating effects of food-derived compounds. Food Funct. 2010; 1:254–261.


16. Bravo L. Polyphenols: chemistry, dietary sources, metabolism, and nutritional significance. Nutr Rev. 1998; 56:317–333.


17. Di Tomo P, Canali R, Ciavardelli D, Di Silvestre S, De Marco A, Giardinelli A, Pipino C, Di Pietro N, Virgili F, Pandolfi A. beta-Carotene and lycopene affect endothelial response to TNF-alpha reducing nitro-oxidative stress and interaction with monocytes. Mol Nutr Food Res. 2012; 56:217–227.


18. Middleton E Jr, Kandaswami C, Theoharides TC. The effects of plant flavonoids on mammalian cells: implications for inflammation, heart disease, and cancer. Pharmacol Rev. 2000; 52:673–751.
19. Choi EM, Hwang JK. Effects of Morus alba leaf extract on the production of nitric oxide, prostaglandin E2 and cytokines in RAW264.7 macrophages. Fitoterapia. 2005; 76:608–613.


20. Kim SH, Oh SY. Cultural and nutritional aspects of traditional Korean diet. World Rev Nutr Diet. 1996; 79:109–132.


21. Kesteloot H, Zhang J. Salt consumption during the nutrition transition in South Korea. Am J Clin Nutr. 2000; 72:199–201.


22. Lee J, Demissie K, Lu SE, Rhoads GG. Cancer incidence among Korean-American immigrants in the United States and native Koreans in South Korea. Cancer Control. 2007; 14:78–85.


23. Gordon BH, Kang MS, Cho P, Sucher KP. Dietary habits and health beliefs of Korean-Americans in the San Francisco Bay Area. J Am Diet Assoc. 2000; 100:1198–1201.


24. Chon SU, Heo BG, Park YS, Kim DK, Gorinstein S. Total phenolics level, antioxidant activities and cytotoxicity of young sprouts of some traditional Korean salad plants. Plant Foods Hum Nutr. 2009; 64:25–31.


25. Kim NY, Song EJ, Kwon DY, Kim HP, Heo MY. Antioxidant and antigenotoxic activities of Korean fermented soybean. Food Chem Toxicol. 2008; 46:1184–1189.


26. Ministry of Health and Welfare, Korea Centers for Disease Control and Prevention. Korea Health Statistics 2010: Korea National Health and Nutrition Examination Survey (KNHANES V-1). Cheongwon: Korea Centers for Disease Control and Prevention;2011.
27. Lee H, Cho MR, Chang N, Kim Y, Oh S, Kang M. Total antioxidant capacity of the Korean diet. Nutr Res Pract. 2013; Forthcoming.


28. Hayashi H, Tatebe S, Osaki M, Goto A, Sato K, Ito H. Anti-Fas antibody-induced apoptosis in human colorectal carcinoma cell lines: role of the p53 gene. Apoptosis. 1998; 3:431–437.
29. Guesdon F, Ikebe T, Stylianou E, Warwick-Davies J, Haskill S, Saklatvala J. Interleukin 1-induced phosphorylation of MAD3, the major inhibitor of nuclear factor kappa B of HeLa cells. Interference in signalling by the proteinase inhibitors 3,4-dichloroisocoumarin and tosylphenylalanyl chloromethylketone. Biochem J. 1995; 307:287–295.


30. Park S, Kim J, Kim Y. Mulberry leaf extract inhibits cancer cell stemness in neuroblastoma. Nutr Cancer. 2012; 64:889–898.


31. Wilson KT, Ramanujam KS, Mobley HL, Musselman RF, James SP, Meltzer SJ. Helicobacter pylori stimulates inducible nitric oxide synthase expression and activity in a murine macrophage cell line. Gastroenterology. 1996; 111:1524–1533.


32. Tracey KJ, Cerami A. Tumor necrosis factor: a pleiotropic cytokine and therapeutic target. Annu Rev Med. 1994; 45:491–503.
33. Song Y, Joung H. A traditional Korean dietary pattern and metabolic syndrome abnormalities. Nutr Metab Cardiovasc Dis. 2012; 22:456–462.


34. Berthet J, Damien P, Hamzeh-Cognasse H, Arthaud CA, Eyraud MA, Zéni F, Pozzetto B, McNicol A, Garraud O, Cognasse F. Human platelets can discriminate between various bacterial LPS isoforms via TLR4 signaling and differential cytokine secretion. Clin Immunol. 2012; 145:189–200.


35. Mihara M, Hashizume M, Yoshida H, Suzuki M, Shiina M. IL-6/IL-6 receptor system and its role in physiological and pathological conditions. Clin Sci (Lond). 2012; 122:143–159.


36. Stokkers PC, Camoglio L, van Deventer SJ. Tumor necrosis factor (TNF) in inflammatory bowel disease: gene polymorphisms, animal models, and potential for anti-TNF therapy. J Inflamm. 1995; 47:97–103.
37. Wang Z, Jiang W, Zhang Z, Qian M, Du B. Nitidine chloride inhibits LPS-induced inflammatory cytokines production via MAPK and NF-kappaB pathway in RAW 264.7 cells. J Ethnopharmacol. 2012; 144:145–150.


38. Cruz JR, Monterroso MA, Zeissig OA, Hazendonk AG, Van Wezel AL. Paralytic poliomyelitis in Guatemala. Bol Oficina Sanit Panam. 1987; 103:123–129.
39. Appleby SB, Ristimäki A, Neilson K, Narko K, Hla T. Structure of the human cyclo-oxygenase-2 gene. Biochem J. 1994; 302:723–727.


40. Chen GY, Tang J, Zheng P, Liu Y. CD24 and Siglec-10 selectively repress tissue damage-induced immune responses. Science. 2009; 323:1722–1725.


41. Deguchi Y, Kishimoto S. Tumour necrosis factor/cachectin plays a key role in autoimmune pulmonary inflammation in lupus-prone mice. Clin Exp Immunol. 1991; 85:392–395.


42. Aggarwal BB, Natarajan K. Tumor necrosis factors: developments during the last decade. Eur Cytokine Netw. 1996; 7:93–124.
43. Harré EM, Roth J, Pehl U, Kueth M, Gerstberger R, Hübschle T. Selected contribution: role of IL-6 in LPS-induced nuclear STAT3 translocation in sensory circumventricular organs during fever in rats. J Appl Physiol (1985). 2002; 92:2657–2666.


44. Beg AA, Finco TS, Nantermet PV, Baldwin AS Jr. Tumor necrosis factor and interleukin-1 lead to phosphorylation and loss of I kappa B alpha: a mechanism for NF-kappa B activation. Mol Cell Biol. 1993; 13:3301–3310.


45. Park OJ, Surh YJ. Chemopreventive potential of epigallocatechin gallate and genistein: evidence from epidemiological and laboratory studies. Toxicol Lett. 2004; 150:43–56.


46. Bai SK, Lee SJ, Na HJ, Ha KS, Han JA, Lee H, Kwon YG, Chung CK, Kim YM. beta-Carotene inhibits inflammatory gene expression in lipopolysaccharide-stimulated macrophages by suppressing redox-based NF-kappaB activation. Exp Mol Med. 2005; 37:323–334.


47. Liu D, Shi J, Colina Ibarra A, Kakuda Y, Jun Xue S. The scavenging capacity and synergistic effects of lycopene, vitamin E, vitamin C, and β-carotene mixtures on the DPPH free radical. Lebenson Wiss Technol. 2008; 41:1344–1349.


SUPPLEMENTARY MATERIALS
Fig. S1
PEKs do not affect cell viability in RAW264.7 macrophages. Cells were treated with various doses of each PEK (2, 4, or 8 mg/ml) for 24 h. The cell numbers were counted using a hemocytometer after staining with trypan blue for enumerating viable cells. Stained and unstained cells were counted and the proportion of surviving cells was calculated for cell viability. One-way ANOVA was implemented using Tukey's post-hoc test (α = 0.05). Mix PKE (A), Veg PKE (B), and Fruit PKE (C) are shown. Mix, an extract containing all ten food groups; Veg, vegetable PKE; Fruit, fruit PKE.