Abstract
Many aging male suffer various andropause symptoms including loss of physical and mental activities. This study evaluated the putative alleviative effects of CRS-10 dandelion and rooibos extract complex (CRS-10) on the symptoms of andropause. The survival rate of TM3 Leydig cells (TM3 cells) treated with CRS-10 was measured based on typical physiological stress. After daily intake of CRS-10 for 4 weeks, the level of testosterone, physical activity and both the number and activity of sperm in older rats (18 weeks) were measured. Furthermore, thirty males were surveyed with AMS (Aging Males' Symptoms) questionnaire after intake of 400 mg of CRS-10. Overall, CRS-10 protected TM3 cells from serum restriction and oxidative stress via activation of ERK and Akt pathways. The level of testosterone and activation of spermatogenesis in rats were significantly enhanced. In addition, physical locomotion was markedly improved. Daily intake of 400 mg of CRS-10 improved the quality of life among agingmale respondents, according to a clinical survey using the AMS. The results indicate the potential of CRS-10 as a safe and efficacious natural substance for reducing or alleviating andropause symptoms.
Leydig cells in the interstitium of testis are the primary source of testosterone, which stimulates the differentiation of the male phenotype and spermatogenesis in the testes [1,2]. Loss of Leydig cells significantly contributes to the age-related decline of serum testosterone [3-5]. Substantial losses in serum testosterone may result in the inactivation of spermatogenesis, loss of muscle strength, as well as declines in muscle performance and physical function, which can detract from the quality of life (QoL) of aging males [6-8]. A predominant proportion of aging and older men have reduced levels of serum testosterone, which is a major reason for andropause symptoms [5].
Several recent studies have shown that the restoration of testosterone level improves andropause symptoms, such as loss of skeletal muscle, depressed mood, increased fat mass, sexual dysfunction and osteopenia [9,10]. However, injection of artificial testosterone can simultaneously induce many side effects including prostate cancer, benign prostatic hyperplasia and cardiovascular events [11,12]. Therefore, identification and refinement of natural substances, which can protect Leydig cells and support the continued production of serum testosterone, is very crucial for reducing the symptoms of andropause.
Dandelions are composed of a variety of compounds including sesquiterpene lactones and phenylpropanoids, which appear to possess a broad-spectrum of anti-bacterial, anti-fungal, anti-viral, anti-diabetic, anti-inflammatory, hepatoprotective, diuretic and tumor apoptosis-inducing properties [13-16]. Rooibos, which is produced in leaves of Aspalathus linearis, contains flavonol, quercetin, flavones (orientin and soorientin), luteolin dihydrochalcone, aspalathin, isoquercitrin and rutin [17-19]. Rooibos has been implicated as being beneficial in the reduction of inflammation, hyperglycemia oxidative stress and liver damage [20-22]. The effects, if any, of dandelion extract and rooibos on the reduction of andropause symptoms of aging male have not been studied.
In this study, we assessed the prowess of extracts from dandelion (ED) or rooibos (ER), individually and the complex of ED and ER (CRS-10) could protect TM3 Leydig cells (TM3 cells) and increase serum testosterone in aging male rats. Also, the clinical availability of CRS-10, which reduces andropause symptoms, was evaluated with aging male.
Dried dandelion was extracted twice with hot water and filtered, followed by concentration and drying processes. Fermented leaves of Rooibos (Aspalathus linearis) were extracted twice with hot water and filtered, followed by concentration and spray drying processes. The dandelion and rooibos extracts were mixed in order to generate CRS-10.
TM3 cells, an immature mouse Leydig cell line (ATCC, USA), were maintained in Dulbecco's modified Eagle's medium (DMEM)/F-12 medium (GIBCO®, Life Technologies Co., USA), supplemented with glutamine, antibiotics and 10% fetal bovine serum (FBS; GIBCO®) at 37℃. Cells (75,000 cells/1.0 ml medium/well) were seeded in 24-well cluster dishes (Corning, USA) for binding and competition assays, unless otherwise specified.
The cells were plated on 96-well plates (Corning) in 10% FBS/DMEM/F-12 and incubated for 24 h. To create oxidative cellular stress, hydrogen peroxide (H2O2) was treated for two hours. To make low serum stress, the cells were incubated in 1% FBS/DMEM/F-12 for 24 h. Subsequently, Alamar Blue (Invitrogen, USA) was aseptically added to the cells from either the oxidative stress condition or from the low serum. The cells were incubated for 3 h and the absorbance of the cells was measured at a wavelength of 570 nm using an ELISA Reader (Molecular Devices, USA). The background absorbance was measured at 600 nm and was then subtracted. The cell viability was defined as [(test sample count - blank count) ÷ (untreated control count - blank count)] × 100.
After treatment, total cellular protein was obtained by placing cell in a lysis buffer consisting of 0.1% sodium dodecyl sulfate (SDS) in phosphate buffered saline (PBS), followed by a brief sonication. Protein concentration was determined by a BCA protein assay (Pierce Chemical Co., USA). Thirty micrograms of total cellular protein were separated by 12% SDS-polyacrylamide gel electrophoresis (SDS-PAGE) and then transferred to nitrocellulose membranes. Blots were probed with an antibody specific for the following proteins: β-actin (1:5,000 dilution; Assay Designs, USA); phosphate-extracellular signal regulated kinase (p-ERK) and total ERK (1:1,000 dilution; Cell Signaling Technology, USA), and phosphate-Akt (p-Akt) and total Akt (1:500 dilution; Santa Cruz Biotechnology, USA). Detection of bound antibody on each blot was assessed with horseradish peroxidase-conjugated secondary antibody visualized by enhanced chemiluminescence (ECL; Western blot detection kit; Amersham Pharmacia Biotech, USA).
18-week-old male Sprague-Dawley rats were purchased from SAMTAKO (Gyeonggi, Korea). The animals were used in the experiments after 1 week of acclimation. The rats were housed in an air-conditioned room that was controlled for temperature (23 ± 3℃) and humidity (55%) with a 12 h dark/12 h light cycle (lights on from 8:00 until 20:00), with unrestricted access to water and food. Ten rats were orally administrated with 40 mg/kg flour daily for 4 weeks, as the control (CTL) group. Another 10 rats were orally administrated with 40 mg/kg CRS-10 daily for 4 weeks, as the experimental group.
Blood samples were collected from 18-22-week-old male rats (between 14:00 and 16:00) and then the amount of serum testosterone was measured by a standard coated-tube radioimmunoassay kit (Diagnostic Systems Laboratories, USA).
The rats were placed on a standard accelerating rotarod device (Harvard Apparatus, USA). Following a 30-day training session at a fixed speed, the rats were tested twice on the rotarod at an accelerating speed of 40 rpm over a duration of 5 min or until the animals fell off. The time on the bar and the speed attained on the rotarod prior to the experiment were recorded and the better score of the two trials was used for analysis.
A swimming retention test evaluating the physical function of the rats was performed, and the results were recorded throughout the 4 weeks. The swimming exercise was performed in a plastic barrel filled with water with the temperature controlled at 32-36℃. After 30 days, the rats were subjected to the swimming exercise daily for 4 weeks. This exercise always took place at noon. The swimming times was recorded weekly. Swimming times, definedas the time from the start of swimming until the time when the rats were exhausted and sank below the surface of the water for 10 sec, were measured. After completing the measurement of the swimming time, the rats were immediately rescued from the water to avoid drowning.
Two cauda epididymis from 18-22 weeks-old male rats were harvested at the same time each day (between 14:00 and 16:00). The sperm-containing fluid was squeezed out of the cauda, which was later cut into pieces. The sperm fluid and pieces of cauda were suspended in HEPES buffer (GIBCO®). Suspensions of spermatozoa were fixed in 10% formalin. A hemocytometer was used to determine the number of spermatozoa. To measure the activity of the sperm, the sperm were incubated in M199 medium (GIBCO®) containing 0.5% BSA for 5 min. Mobilesperm were enumerated.
Thirty men over 40 years of age and a matching placebo group were recruited at Chung-Ang University Medical Center, Seoul, Korea. Males were excluded from the study if they were presented with any of the following conditions: metabolic diseases, prostatic hyperplasia, development of clinical prostate cancer, urethral stricture, neurogenic bladder, cardiovascular events, thyroid disease during the past year or use of medications containing sex hormones.
Demographics and other clinical dates were obtained through the Aging Males' Symptoms (AMS) questionnaire. The study protocol was approved by the Institutional Review Boards at the participating hospitals (Chung-Ang University Medical Center). Volunteers were randomly distributed into one of three groups by a pharmacist who was not aware of any clinical information of the subjects. Group 1 (n = 10) was the placebo group. Group 2 (n = 10) was treated with 200 mg of CRS-10 daily and group 3 (n = 10) was treated with 400 mg of CRS-10 daily. During the study period, the investigator, rater and the study participants were double-blinded. AMS data were compiled before the CRS-10 regimen and after the 4-week regimen.
Student's t-test was used in order to compare the demographic variables between the CRS-10 and placebo groups. One-way ANOVA was used for comparisons either between CRS-10 and the control group or between the before and after administration of CRS-10.All statistical analyses were performed using SPSS 11.0 (SPSS, USA) with statistical significance defined at an alpha level < 0.05.
To evaluate the effects of ED, ER and CRS-10, TM3 cells were incubated with either 10 or 50 µg/ml of natural extracts in low serum (1%) medium. At the low serum medium, TM3 cells displayed a generally decreased proliferation rate and induction of apoptotic cell death. However, treatment of CRS-10 increased the survival rate of TM3 cells (Fig. 1A). Treatment of CRS-10 also increased cell viability of TM3 cells by upto 38%, while statistically significant increased cell viability was not detected from the treatment of either ED or ER. Moreover, the cells in CRS-10 treated media were healthy without apoptotic features, whereas some control cells displayed apoptotic morphological features, such as floating and loss of cellular shape (Fig. 1B). These results were consistent with the ability of CRS-10, which are necessary to maintain TM3 cells in a viable and healthy state when under physiological stress.
To determine whether CRS-10 could protect TM3 cells from H2O2-induced damage, the Alamar Blue assay was examined. As expected, 2 h incubation with H2O2 significantly decreased cell viabilityby 40% compared to the cultured cell without H2O2 (Fig. 2). Cell viabilities were increased to 138% and 148% by the treatment with CRS-10 concentrations of 10 µg/ml and 50 µg/ml, respectively, indicating a dose-dependent protection (Fig. 2). Even though the cell viabilities were increased by 50 µg/ml ED (approximately 120%) and by 10 and 50 µg/ml ER (both approximately 128%), the improvement was lower compared to CRS-10. A dose-dependent effect was not evident using ED (Fig. 2). Thus, CRS-10 was much more effective in protecting TM3 cells than were ED or ER, even at a lower concentration.
The next experiment assessed whether CRS-10 could protect TM3 cells from H2O2-induced injury by regulating ERK and Akt phosphorylation. Phosphorylation of both kinases is instrumental in general cellular defense against various stresses in human cells. As shown in the Western blot analyses, the phosphorylation of ERK and Akt were significantly increased by 50 µg/ml of CRS-10 (Fig. 3A). Cell viability in the CRS-10-treated group was reduced to approximately 125% and 120%, respectively, by treatment with the ERK inhibitor PD98059 or the Akt inhibitor LY294002 (Fig. 3B). These results indicated that CRS-10 was capable of effectively protecting against H2O2-induced injury through asimultaneous activation of ERK and Akt.
We further studied the effect of CRS-10 on serum testosterone level, capacity for locomotion and spermatogenesis in aging male rats (18 weeks). Ten milligrams of CRS-10 was feed each day for 4 weeks. CRS-10 significantly up-regulated serum testosterone levels from approximately 3.52 ng/ml to 5.04 ng/ml (Fig. 4A). The improved rate was about 43% compared with the baseline (Fig. 4B). However, no significant increase was detected in the control group (Fig. 4A).
Testosterone enhances physical locomotion capacity [7]. To evaluate the effect of CRS-10 on the adaptation process of rat skeletal muscles to long-term physical strength, Rotarod and swimming retention tests were performed. Control and CRS-10-treated rats were trained three times a day for 4 weeks. The latency time on the rotarod was increased from about 200 sec to 250 sec by treatment of CRS-10, representing a 25% improvement. The controls showed no increased time (Fig. 5A). Further, the CRS-10 treated rats showed a significantly increased swimming time with practice, while the control rats did not (Fig. 5B).
Testosterone has an important role during spermatogenesis in the number and activity of the sperm. To evaluate the effects of CRS-10 on spermatogenesis, the number and activity of the sperm was determined in control and CRS-10-treated rats. The sperm number per gram of epididymis was significantly increased from approximately 51.6 × 106 to approximately 60.8 × 106 by CRS-10 treatment (Fig. 6A), representing a 20% improvement rate (Fig. 6C). However, no significant change in the sperm number was detected in the control rats (Fig. 6A).
The activity of sperms was represented as a portion of active sperms 4 h after sperm acquisition. The portion of active sperm was markedly increased from 50% to 71% by the treatment of CRS-10 (Fig. 6B), representing over a 40% improvement (Fig. 6C). However, no significant variation was detected in the control (Fig. 6B). The control group displayed no differences in both the number and activity of sperms. No significant changes of body and testis were detected in the CRS-10 and control groups (Table 1).
The dose-dependent effects of CRS-10 on the QoL of aging male were assessed using the AMS clinical questionnaire. Ten normal men were treated with 400 mg of CRS-10/day and 10 other men were treated with 200 mg/day for 4 weeks. Also, 10 normal men, as the placebo group, were treated with 400 mg of dextran for 4 weeks. All groups then completed the AMS questionnaire in a double-blind manner. The men treated with 400 mg of CRS-10 reported significantly fewer negative answers to QoL-related questions. A negative answer score of about 39 prior to CRS-10 treatment was reduced to about 30 following the treatment regimen (Fig. 7A), representing a 20% improvement rate (Fig. 7B). The group receiving 200 mg of CRS-10 and the placebo group did not display significant differences before and after treatment, although the number of negative answers was slightly reduced in the 200 mg CRS-10 treated group (Fig. 7A).
Safe natural substances capable of reducing andropause symptoms of men are essential, due to the fact that an increasing proportion of men will become affected as the general life span is prolonged. The present study screened for the efficacy and safety of the combined extract of dandelions and rooibos (CRS-10).
Leydig cells of males are continuously exposed to various harmful stimulations, particularly from mid-age onward. These cells sustain gradual and progressive damage, along with age-related loss of physiological functions. As a result, their capacity to produce testosterone declines. Andropause syndrome in aging male is mainly due to testosterone reduction. Consequences include loss of skeletal muscle, decrease of locomotive capacity, depression, oligozoospermia and inactivation of sperm [6,23-26]. The present results indicate that CRS-10 may play a significant positive role in the health of TM3 cells, and thus result in the sustained production of testosterone.
Aged men suffer from accumulated physiological stresses. Through the results of this study, it has been revealed that CRS-10 increased TM3 cells viability in stressed conditions (e.g., low serum medium) and effectively protected TM3 cells against physiological oxidative stress. It has been known that testosterone exerts various positive roles including protection of the body from the stress and reduction of andropause symptoms in aging males. The testosterone is produced in Leydig cells, similar to TM3 cells in the testis. Hence, the protective effect of CRS-10 on the reproductive cells and organ might be crucial for the health and anti-aging of men. CRS-10 treatment could maintain the health of the cells by the activation of the ERK and Akt pathways. The present results are consistent with previous reports, suggesting the involvement of the pathways in the protection of cells from various insults, which is critical for maintaining a functional population of adult Leydig cells as well as for fertility [27-29].
The collective data supports the suggestion that the increase of testosterone in the serum of CRS-10 treated rats might be engendered by the protective and activating roles of CRS-10 on Leydig cells, since the cells produce testosterone in the testis [30,31].
Interestingly, the testosterone level of CRS-10 treated rats was increased, together with asignificant enhancement of physical locomotion activities. Several previous studies showed that testosterone improves skeletal muscle and strength in a dose-dependent manner, and thus improves physical performance [7,9,32]. Increased testosterone production in the presence of CRS-10 implicated in the improved physical capacity of the treated rats in the present study.
Also, CRS-10 simultaneously increased spermatogenesis and sperm activity. The serum testosterone level is linked with the production of active sperm. Testosterone improves the efficacy of germ cell differentiation and active maturation [33,34]. Recently, testosterone has been used in the clinical treatment of andropause patients with oligozoospermia and maturation arrest of sperms, despite its various side effects [10,11,35,36]. Hence, consistent with previous studies, it can be suggested that the enhanced activity and health of Leydig cells together with the enhanced serum testosterone levels by CRS-10 were the bases of improved spermatogenesis and activation of sperm. Furthermore, CRS-10 was markedly effective in the clinical study with aging male, as judged using the AMS questionnaire, which is an established means of diagnosing andropause symptoms of men [37-39]. Dose dependent effects of CRS-10 were evident, which related to the specific positive effects on andropause symptoms. The effective clinical dose was 400 mg/day.
In conclusion, CRS-10 effectively improved andropause syndromes clinically and also enhanced physical performance as well as activation of spermatogenesis. These positive effects may have occurred through protection and activation of Leydig cells, together with induced generation of endogenous testosterone.
Figures and Tables
Fig. 1
Protective effect of CRS-10 against low serum-stress. The cell viability was determined biochemically, using Alamar Blue assay, at 24 h after replacement with 1% FBS-containing medium (A). The photographs represent the optical phase contrast microscopic morphology (B). The values are mean ± SE of four separate independent experiments. The difference from the cells incubated with 10% serum was statistically significant *P < 0.05.

Fig. 2
Protective effects of CRS-10 against H2O2. TM3 cells were treated with 40 µM of H2O2 for 2 h. The cells were pretreated with either CRS-10 or dandelion or rooibos 2 h before H2O2 treatment. The cell viability was determined biochemically using the Alamar Blue assay. Open bar is the cell viability of non-treated TM3 cells in 10% FBS-medium. The difference from the cells treated with H2O2 alone (black bar) was statistically significant *P < 0.05.

Fig. 3
Enhanced activation of ERK and Akt by CRS-10 may protect TM3 cells against H2O2. After 24 h of treatment, CRS-10 treatment enhanced the activation of ERK and Akt (A). The cells were pretreated with either 10 µM PD98059 (ERK inhibitor) or 10 µM LY294002 (Akt inhibitor) 2 h before CRS-10 and H2O2 co-treatments. The cell viability was determined biochemically using the Alamar Blue assay. The difference from the cells treated with H2O2 alone was statistically significant *P < 0.05 (B). ERK, Extracellular signal-regulated kinases.

Fig. 4
Increased serum testosterone level by CRS-10. Testosterone level in the serum represents the mean ± SE. The serum testosterone levels before and after administration of CRS-10 were compared using one-way ANOVA (A). The solid bar shows the percentage of serum testosterone level of CRS-10 and placebo groups compared with the baseline (B). Significant differences are denoted by * vs. before, P < 0.05 and † vs. control, P < 0.05.

Fig. 5
Positive role of CRS-10 in the enhancement of physical activity. To evaluate the enhancement of physical function by CRS-10, Rotarod and swimming retention test were performed. The rats were trained with rotarod and swimming exercise 30 min a day for 30 days. Running time on the wheel is presented as the mean ± SE (A). Retention time, swimming time without stop for 10 sec, was determined weekly (B). Significant differences are denoted by * vs. before, P < 0.05 and † vs. control, P < 0.05.

Fig. 6
Enhanced spermatogenesis and sperm activity by CRS-10. Number of sperm before and after administration of CRS-10 was compared using one-way ANOVA (A). Activated sperms by CRS-10, which can maintain movement after 5 min of medium incubation, were counted (B). These improvements by CRS-10 were presented as the percentage compared with the baseline (C). Significant differences are denoted by * P < 0.05.
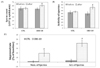
Fig. 7
Improved AMS score by 400 mg/day CRS-10. AMS questionnaire was completed at 0 and 4 weeks. Scores of the AMS answersare presented as mean ± SE (A). The scores before and after administration of CRS-10 were compared using one-way ANOVA. The solid bar shows the percentage of the AMS score of CRS-10 and placebo groups compared with the baseline (B). Significant differences are denoted by * (P < 0.05). AMS, Aging Males' Symptoms.

References
1. Zhang Y, Ge R, Hardy MP. Androgen-forming stem Leydig cells: identification, function and therapeutic potential. Dis Markers. 2008. 24:277–286.


2. Chen L, Zhao Y, Zhang Y. Progress on the research of stem Leydig cell line in the testis of rodents. Wei Sheng Yan Jiu. 2012. 41:158–162.
3. Wang C, Hikim AS, Ferrini M, Bonavera JJ, Vernet D, Leung A, Lue YH, Gonzalez-Cadavid NF, Swerdloff RS. Male reproductive ageing: using the brown Norway rat as a model for man. Novartis Found Symp. 2002. 242:82–95. discussion 95-7.


4. Luo L, Chen H, Zirkin BR. Are Leydig cell steroidogenic enzymes differentially regulated with aging? J Androl. 1996. 17:509–515.
5. Wu CY, Yu TJ, Chen MJ. Age related testosterone level changes and male andropause syndrome. Chang Gung Med J. 2000. 23:348–353.
6. Travison TG, Basaria S, Storer TW, Jette AM, Miciek R, Farwell WR, Choong K, Lakshman K, Mazer NA, Coviello AD, Knapp PE, Ulloor J, Zhang A, Brooks B, Nguyen AH, Eder R, LeBrasseur N, Elmi A, Appleman E, Hede-Brierley L, Bhasin G, Bhatia A, Lazzari A, Davis S, Ni P, Collins L, Bhasin S. Clinical meaningfulness of the changes in muscle performance and physical function associated with testosterone administration in older men with mobility limitation. J Gerontol A Biol Sci Med Sci. 2011. 66:1090–1099.


7. Sattler F, Bhasin S, He J, Chou CP, Castaneda-Sceppa C, Yarasheski K, Binder E, Schroeder ET, Kawakubo M, Zhang A, Roubenoff R, Azen S. Testosterone threshold levels and lean tissue mass targets needed to enhance skeletal muscle strength and function: the HORMA trial. J Gerontol A Biol Sci Med Sci. 2011. 66:122–129.


8. Chen RY, Ng KK. Self-referred older Asian males in a men's health clinic: the inter-relationships between androgens, metabolic parameters and quality of life measures. Aging Male. 2010. 13:233–241.


9. Ibebunjo C, Eash JK, Li C, Ma Q, Glass DJ. Voluntary running, skeletal muscle gene expression, and signaling inversely regulated by orchidectomy and testosterone replacement. Am J Physiol Endocrinol Metab. 2011. 300:E327–E340.


10. Nigro N, Christ-Crain M. Testosterone treatment in the aging male: myth or reality? Swiss Med Wkly. 2012. 142:w13539.


11. Barqawi A, Crawford ED. Testosterone replacement therapy and the risk of prostate cancer. Is there a link? Int J Impot Res. 2006. 18:323–328.


12. Tenover JL. Male hormone replacement therapy including "andropause". Endocrinol Metab Clin North Am. 1998. 27:969–987. x


13. Hu C, Kitts DD. Dandelion (Taraxacum officinale) flower extract suppresses both reactive oxygen species and nitric oxide and prevents lipid oxidation in vitro. Phytomedicine. 2005. 12:588–597.


14. He W, Han H, Wang W, Gao B. Anti-influenza virus effect of aqueous extracts from dandelion. Virol J. 2011. 8:538.


15. Huang Y, Wu T, Zeng L, Li S. Chinese medicinal herbs for sore throat. Cochrane Database Syst Rev. 2012. 3:CD004877.


16. Ovadje P, Chatterjee S, Griffin C, Tran C, Hamm C, Pandey S. Selective induction of apoptosis through activation of caspase-8 in human leukemia cells (Jurkat) by dandelion root extract. J Ethnopharmacol. 2011. 133:86–91.


17. Utter AC, Quindry JC, Emerenziani GP, Valiente JS. Effects of rooibos tea, bottled water, and a carbohydrate beverage on blood and urinary measures of hydration after acute dehydration. Res Sports Med. 2010. 18:85–96.


18. Darvesh AS, Carroll RT, Bishayee A, Geldenhuys WJ, Van der Schyf CJ. Oxidative stress and Alzheimer's disease: dietary polyphenols as potential therapeutic agents. Expert Rev Neurother. 2010. 10:729–745.


19. Bramati L, Aquilano F, Pietta P. Unfermented rooibos tea: quantitative characterization of flavonoids by HPLC-UV and determination of the total antioxidant activity. J Agric Food Chem. 2003. 51:7472–7474.


20. Awoniyi DO, Aboua YG, Marnewick J, Brooks N. The effects of rooibos (Aspalathus linearis), green tea (Camellia sinensis) and commercial rooibos and green tea supplements on epididymal sperm in oxidative stress-induced rats. Phytother Res. 2012. 26:1231–1239.


21. Marnewick JL, Rautenbach F, Venter I, Neethling H, Blackhurst DM, Wolmarans P, Macharia M. Effects of rooibos (Aspalathus linearis) on oxidative stress and biochemical parameters in adults at risk for cardiovascular disease. J Ethnopharmacol. 2011. 133:46–52.


22. Baba H, Ohtsuka Y, Haruna H, Lee T, Nagata S, Maeda M, Yamashiro Y, Shimizu T. Studies of anti-inflammatory effects of rooibos tea in rats. Pediatr Int. 2009. 51:700–704.


23. Tan RS, Pu SJ. Impact of obesity on hypogonadism in the andropause. Int J Androl. 2002. 25:195–201.


24. Vermeulen A, Kaufman JM. Ageing of the hypothalamo-pituitary-testicular axis in men. Horm Res. 1995. 43:25–28.


25. Vermeulen A. Environment, human reproduction, menopause, and andropause. Environ Health Perspect. 1993. 101:Suppl 2. 91–100.


26. Bornman MS, Reif S. Serum testosterone levels in South African men and the onset of androgen decline in ageing males. S Afr J Surg. 2007. 45:62. 64.
27. Moon C, Kim JS, Jang H, Lee HJ, Kim SH, Kang SS, Bae CS, Kim JC, Kim S, Lee Y, Shin T. Activation of Akt/protein kinase B and extracellular signal-regulated kinase in rats with acute experimental testicular torsion. J Vet Med Sci. 2008. 70:337–341.


28. Kim JY, Han EH, Kim HG, Oh KN, Kim SK, Lee KY, Jeong HG. Bisphenol A-induced aromatase activation is mediated by cyclooxygenase-2 up-regulation in rat testicular Leydig cells. Toxicol Lett. 2010. 193:200–208.


29. Yamashita S, Tai P, Charron J, Ko C, Ascoli M. The Leydig cell MEK/ERK pathway is critical for maintaining a functional population of adult Leydig cells and for fertility. Mol Endocrinol. 2011. 25:1211–1222.


30. Walsh PC, Swerdloff RS. Biphasic effect of testosterone on spermatogenesis in the rat. Invest Urol. 1973. 11:190–193.
31. Chen H, Liu J, Luo L, Zirkin BR. Dibutyryl cyclic adenosine monophosphate restores the ability of aged Leydig cells to produce testosterone at the high levels characteristic of young cells. Endocrinology. 2004. 145:4441–4446.


32. Horstman AM, Dillon EL, Urban RJ, Sheffield-Moore M. The role of androgens and estrogens on healthy aging and longevity. J Gerontol A Biol Sci Med Sci. 2012. 67:1140–1152.


33. Bormann CL, Smith GD, Padmanabhan V, Lee TM. Prenatal testosterone and dihydrotestosterone exposure disrupts ovine testicular development. Reproduction. 2011. 142:167–173.


34. Sofikitis N, Giotitsas N, Tsounapi P, Baltogiannis D, Giannakis D, Pardalidis N. Hormonal regulation of spermatogenesis and spermiogenesis. J Steroid Biochem Mol Biol. 2008. 109:323–330.


35. Schwartz E, Holtorf K. Hormone replacement therapy in the geriatric patient: current state of the evidence and questions for the future. Estrogen, progesterone, testosterone, and thyroid hormone augmentation in geriatric clinical practice: part 1. Clin Geriatr Med. 2011. 27:541–559.


36. Schwartz E, Morelli V, Holtorf K. Hormone replacement therapy in the geriatric patient: current state of the evidence and questions for the future--estrogen, progesterone, testosterone, and thyroid hormone augmentation in geriatric clinical practice: part 2. Clin Geriatr Med. 2011. 27:561–575.


37. Heinemann LA. Aging Males' Symptoms scale: a standardized instrument for the practice. J Endocrinol Invest. 2005. 28:34–38.