Abstract
Resveratrol (3,4,5-trihydroxy-trans-stilbene), a phytoalexin found in grape skin, grape products, and peanuts as well as red wine, has been reported to have various biological and pharmacological properties. The purpose of this study was to investigate the anti-obesity effect of resveratrol-amplified grape skin extracts on adipocytes. The anti-obesity effects of grape skin extracts were investigated by measuring proliferation and differentiation in 3T3-L1 cells. The effect of grape skin ethanol extracts on cell proliferation was detected by the MTS assay. The morphological changes and degree of adipogenesis of preadipocyte 3T3-L1 cells were measured by Oil Red-O staining assay. Treatment with extracts of resveratrol-amplified grape skin decreased lipid accumulation and glycerol-3-phosphate dehydrogenase activity without affecting 3T3-L1 cell viability. Grape skin extract treatment resulted in significantly attenuated expression of key adipogenic transcription factors, including peroxisome proliferator-activated receptor, CCAAT/enhancer-binding proteins, and their target genes (FAS, aP2, SCD-1, and LPL). These results indicate that resveratrol-amplified grape skin extracts may be useful for preventing obesity by regulating lipid metabolism.
Resveratrol (3,4,5-trihydroxystilbene) is a naturally occurring polyphenol found in different plant species [1]. Considerable amounts of resveratrol are found in grapes, berries, and peanuts. Besides natural sources, this compound has become recently available in tablets and is recommended as a dietary supplement. In the past few years, interest in resveratrol has increased substantially, and its broad biological activity at the cellular level has been demonstrated [2]. Supplementing mice fed a high fat diet with resveratrol increases mitochondrial content/activity in skeletal muscle brown adipose tissue and the liver to protect against developing diet-induced obesity and improving metabolic disturbances [3]. Moreover, the cardioprotective, anti-cancer, anti-inflammatory, and antioxidant properties of resveratrol are well characterized [4-7]. Recent data derived from animal studies have opened a new, promising perspective for the potential use of resveratrol to prevent serious metabolic disorders such as obesity and diabetes [1,8].
The prevalence of obesity is increasing worldwide [9]. According to the World Health Organization, obesity is a global epidemic, due to the parallel rise of related morbidity and risk factors. Obesity, which is characterized by excess fat, results from interactions between genetic and environmental factors. It is well known that a fat-enriched diet leads to the accumulation of adipose tissue and to the development of metabolic alterations associated with weight gain, particularly in genetically predisposed individuals [10]. Obesity is linked to a variety of metabolic disorders such as insulin resistance and atherosclerosis [11]. Environment, lifestyle, and genetic susceptibility probably contribute to the increased risk of obesity.
Recent data indicate that resveratrol plays a crucial role in the cardiovascular protection provided by grapes and wines [12]. Grapes contain various nutritive elements such as vitamins, minerals, carbohydrates, and fiber. Polyphenols are the most important phytochemicals in grapes, because they possess many biological activities and health-promoting benefits [13]. Recent studies have revealed that resveratrol and proanthocyanidins are the important polyphenolic antioxidants present in grapes. Proanthocyanidins are found in both the skin and seeds of grapes, but resveratrol is mainly found in grape skin [14].
Resveratrol content decreases due to direct sunlight exposure, but ultraviolet (UV) irradiation induces resveratrol biosynthesis in grapes [15]. Recently, Cho et al. [16] showed that resveratrol content in grapes treated with UV irradiation increases five times more than that in untreated grapes.
The present study was conducted to evaluate the antiobesity activity of resveratrol-amplified grape skin extracts in 3T3-L1 preadipocytes and to elucidate the mechanism underlying such an effect as exerted in visceral adipose tissue.
Grape (Vitis labruscana Bailey, Campbell Early) was purchased from Yeongcheon-si Gyeongsangbuk-do, Korea. The grapes were cleaned by sonication (45-50 kHz) for 3-5min and passed through an amplifier (UV-irradiation, 0.5-1.0 mW/cm2) at 253.7nm and then stored at 15-25℃ for 3-6 days to enhance resveratrol content. Grape skin was separated from grape flesh and dried with lyophilizer (MCFD 5510, Eyela, Tokyo, Japan). A powder form of the grape skin was obtained by grinding the skins with a grinder. The grape skin powder was stored at -70℃ until use.
Resveratrol-amplified grape skin powder was weighed (500 g) and extracted twice with ethanol/water (50/50 [v/v]) mixture and ethanol/water (80/20 [v/v]) mixture, respectively. The filtrates were combined and evaporated under a vacuum (Buchi, Berlin, Germany). The yields of the 50% and 80% ethanol extracts of resveratrol-amplified grape skin were 59.3% and 47.9% based on dry weight, respectively.
Total polypehnol content was determined using a colorimetric assay based on the method of Singleton and Rossi [17]. Total antioxidant capacity was measured according to the method of Re et al. [18] and expressed as a Trolox equivalent value. Resveratrol content was analyzed using high performance liquid chromatography (Shimadzu SCL-10Avp, Japan) with a UV-Vis detector at 320 nm.
Mouse embryo 3T3-L1 fibroblasts were obtained from the American Type Culture Collection (Manassas, VA, USA) and grown at 37℃ under a humidified 5% CO2 atmosphere in Dulbecco's modified Eagle's medium containing 10% bovine calf serum and 100 U/ml penicillin-streptomycin. Two days after confluence, preadipocytes of 3T3-L1 (designated as day 0) were cultured in differentiation medium (DM) containing 10% fetal bovine serum [FBS], 10 µg/mL insulin, 0.5 mM isobutylmethylxanthine, and 1 µM dexamethasone. After 2 days, the medium was switched to post DM containing 10% FBS and 10 µg/mL insulin for four days, and then changed to 10% FBS medium for an additional 2 days to induce differentiation.
Cells (5 × 103/96 well) were incubated with resveratrol-amplified grape skin extracts at various concentrations (0, 30, 60, 120, 250, 500, and 1,000 µg/ml) for 24 and 48 h. The control cells were received the same amount of DMSO. Twenty µL of 3-(4,5-dimethylthiazol-2-yl)-5-(3-carboxymethoxyphenyl)-2-(4-s ulfophenyl)-2H-tetrazolium (MTS) solution was added to each well. After incubation for 20 min, absorbance was measured at 490 nm using a microplate reader (Sensident scan, Labsystems, Helsinki, Finland).
Oil Red-O staining was performed on day 8. 3T3-L1 adipocyte cells were washed with phosphate buffered saline (PBS) and fixed with 10% formalin. After Oil Red-O stain, cells were photographed using a phase-contrast microscope (Olympus CKX41, Tokyo, Japan) in combination with a digital camera at 100 × magnification. The lipid droplets were dissolved in isopropanol and measured at 540 nm.
Resveratrol-amplified grape skin extract-treated 3T3-L1 adipocytes were harvested on day 8, washed with PBS, and collected using lysis buffer (25 mM Tris buffer containing 1 mM EDTA and 1 mM DTT (pH 7.5)). The harvested cells were sonicated and then centrifuged at 10,000 rpm for 5 min. The GPDH activity in supernatants was measured with assay kit (Takara Bio, Shiga, Japan).
Total RNA was isolated from 3T3-L1 cells using the EASY-BLUE total RNA extraction kit (Intron Bio, Beverly, MA, USA). cDNA synthesis was performed with 1 µg of total RNA, oligo (15) dT primers, and reverse transcriptase in a total volume of 50 µL. PCR reactions were performed in a total volume of 20 µL comprised of 2 µL cDNA product, 0.2 mM of each dNTP, 20 pmol of each primer, and 0.8 unit of Taq polymerase. The oligonucleotide primer sequences used in the PCR amplification are described in Table 1.
The PCR was performed at 95℃ for 30 s, followed by 58℃ (PPAR-γ, C/EBPα, SREBP1, LPL, actin), 50℃ (FAS), or 56℃ (SCD-1), or 60℃ (aP2) for 30 s, and 72℃ for 1 min. The last cycle was followed by a final extension step at 72℃ for 10 min. The RT-PCR products were electrophoresed on 0.8% agarose gels under 100 V and stained with 0.5 µg/mL ethidium bromide. Scanning densitometry was determined with the i-MAX™ Gel Image Analysis System (Core-Bio, Seoul, Korea).
3T3-L1 cells pads were prepared using lysis buffer. Proteins were separated by 8% SDS-polyacrylamide gel electrophoresis, transferred to polyvinylidene difluoride membranes (Millipore, Billerica, MA, USA), and hybridized overnight with 1:1,000 diluted PPARγ, C/EBPα primary antibodies (Santa Cruz Biotechnology, Santa Cruz, CA, USA). After a 1 h incubation at room temperature with 1:2,000 diluted horseradish-peroxidase-conjugated goat anti-mouse or donkey anti-rabbit secondary antibody (Santa Cruz Biotechnology) immunoreactive proteins were visualized with an enhanced chemiluminescent solution (Amersham, Uppsala, Sweden) and quantified by chemiluminescence with WEST-ZOL Western blotting detection (Intron, Seoul, Korea).
Results are expressed as mean ± standard deviation. Statistical significance was evaluated with a one-way analysis of variance using SPSS ver. 18.0 (Chicago, IL, USA) and the significant differences between means were identified with the Student's t-test at P < 0.05, P < 0.01, and P < 0.001.
The physiological activities of resveratrol-amplified grape skin are shown in Table 2. The amount of total phenols was 37.4 mg/g GAE in the 50% ethanol extract of resveratrol-amplified grape skin and 50.8 mg/g GAE in the 80% ethanol extract of resveratrol-amplified grape skin, respectively. The resveratrol content of resveratrol-amplified grape skin extracts was higher in the 80% ethanol extract (3.06 mg/g) than that in the 50% ethanol extract (2.57 mg/g). Total antioxidant status in the 80% ethanol extract of resveratrol-amplified grape skin increased compared with that in the 50% ethanol extract of resveratrol-amplified grape skin.
The MTS assay was performed to assess the effect of the resveratrol-amplified grape skin extracts on 3T3-L1 cell viability. As shown in Fig. 1 the 50% and 80% ethanol extracts of resveratrol-amplified grape skin at 30, 60, 120, 250, 500, and 1,000 µg/ml showed no significant effect on viability after 24 or 48 h treatment. Therefore, the cells did not become toxic when the 50% and 80% resveratrol-amplified grape skin ethanol extracts were added even at the highest concentration (1,000 µg/ml) for 24 h or 48 h. In a preparatory experiment (data not presented), a dose-dependent effect of resveratrol-amplified grape skin extracts was not observed at extracts doses > 500 µg/ml.
The effect of resveratrol-amplified grape skin extracts on preventing lipid accumulation was examined by Oil Red-O staining of 3T3-L1 adipocytes. The results represent lipid droplet accumulation, because triglycerides in adipocytes stain with Oil-Red-O staining solution apart from free fatty acids and phospholipids. As shown in Fig. 2A, resveratrol-amplified grape skin extracts reduced lipid accumulation, as indicated by decreased Oil Red-O staining. Adipocytes treated with the 50% and 80% ethanol extracts showed reduced lipid droplet size compared with that in differentiated media cells. When differentiation of 3T3-L1 adipocytes was inhibited with the extracts, the size of the lipid droplets was smaller. Triglyceride content in 3T3-L1 adipocytes decreased in response to the 50% ethanol extracts at 400 µg/ml (P < 0.01) and decreased significantly in 80% ethanol extract-treated cells at 200 and 400 µg/ml (P < 0.001, Fig. 2B). Adipocyte differentiation was inhibited more effectively when the cells were treated with the 80% ethanol extract.
GPDH activity was measured to determine the effect of resveratrol-amplified grape skin extracts on differentiation of 3T3-L1 preadipocytes into adipocytes. As shown in Fig. 3, the 80% ethanol extract at 200 (P < 0.01) and 400 µg/ml (P < 0.001) decreased GPDH activity dose-dependently by 71.1 ± 5.3% and 58.4 ± 4.3%, respectively. In contrast, the 50% ethanol extract showed no significant effect on GPDH activity.
RT-PCR and Western blotting analysis were performed to examine the effect of the extracts on the expression of transcriptional factors (Figs. 4 and 5). The extracts decreased PPARγ and C/EBPα mRNA expression. The expression of the PPARγ and C/EBPα genes following treatment with the extracts was significantly lower compared with that of differentiated control adipocytes (P < 0.01, P < 0.001, respectively). Treatment with the extracts inhibited PPARγ and C/EBPα protein expression in a dose-dependent manner (P < 0.01, P < 0.001, respectively).
Gene expression of SREBP1c, FAS, aP2, FAS, SCD-1, and LPL were analyzed using RT-PCR to determine the effect of the extracts on expression of the C/EBPα and PPARγ target genes (Fig. 6). SREBP1c, FAS, aP2, FAS, and SCD-1 gene expression was dose-dependently and significantly downregulated by the grape skin extract treatment. Although LPL expression was significantly blocked by the 80% ethanol extract (P < 0.01), the 50% ethanol extract did not inhibit LPL expression.
Obesity is a risk factor for metabolic diseases such as diabetes, hypertension, and cardiovascular disease. Although many studies have investigated the causes and prevention of obesity, the prevalence of obesity has increased greatly during the past few decades [19]. As adipocytes are the main site for lipid homeostasis, it is useful to conduct studies on obesity-related disorders [20]. The 3T3-L1 cell line is widely used to investigate adipocyte differentiation. In the present study, we focused on the antiobesity effects of resveratrol-amplified grape skin extracts for inhibiting adipogenesis in 3T3-L1 adipocytes.
Resveratrol, a polyphenol found mainly in red grape, has various physiological function including antioxidant, antimutagenic, and immune activity [2,5,7]. Resveratrol has also anticancer and antiobesity effects, although the precise mechanism has not yet been established [21]. The aim of the present study was to investigate the effect of resveratrol-amplified grape skin extracts on the antiobesity and to determine the dose-dependent response and extraction condition for its application in food industry.
Grape skin was treated resveratrol-amplification process by UV-irradiation in this study. In the previous study [22], resveratrol content of grape skin increased 7.2 times by UV irradiation. Therefore, resveratrol-amplification process by UV irradiation was shown to increase resveratrol content of grape skin.
In this study, we found that resveratrol-amplified grape skin extracts suppressed triglyceride accumulation by inhibiting GPDH and decreasing fat droplets during 3T3-L1 adipocyte differentiation. GPDH is a key enzyme of fatty acid and triacylglycerol synthesis in adipocytes, which increases during differentiation of preadipocytes into adipocytes [23]. Park et al. [24] indicated that GPDH activity in primary human adipocytes decreases 31.1% following 12.5 µM resveratrol treatment. These results suggest that the effect on reducing triglyceride content by the 80% resveratrol-amplified grape skin extract treatment was partly due to decreased GPDH activity.
Lipid accumulation and GPDH activity were lower in 80% ethanol extracts of resveratrol-amplified grape skin than in 50% ethanol extracts. Although the difference of resveratrol content between 50% and 80% ethanol extracts was about 20%, it resulted in a significant antiobesity effect in 80% ethanol extracts. Rayalam et al. [25] reported that lipid accumulation significantly decreased in resveratrol treatment at 25 and 50 µM by 43% and 94.3%, respectively. However, further studies are needed to investigate whether another physiological components of grape skin may exert additional beneficial effects in 3T3-L1 adipocytes.
The antiobesity mechanism works by inhibiting fat accumulation and fatty acid synthesis and by activating fatty acid oxidation. PPARγ, a lipid-activated transcription factor, is present in adipose tissue and regulates genes involved in lipid metabolism including FAS and aP2 [26]. Because PPARγ is activated by fatty acids, fat accumulation is associated with PPARγ activation [27,28]. Thus, modulation of PPARγ activity may be an effective way to regulate obesity. In our study, resveratrol-amplified grape skin extracts significantly suppressed PPARγ mRNA and protein expression. Additionally, the expression of C/EBPα, a key regulator of lipogenesis, decreased markedly following extract treatments compared with that in differentiated control cells. PPARγ and C/EBPα play an important role in adipocyte differentiation, because they are transcriptional activators for adipocyte genes including aP2, lipoprotein lipase, and SCD-1 [29]. PPARγ and C/EBPα cooperate to regulate adipocyte differentiation, because there is a positive feedback loop between PPARγ and C/EBPα [30].
Yang et al. [31] demonstrated that a combination of resveratrol and quercetin down-regulates PPARγ and C/EBPα and induces apoptosis, resulting in attenuated fat accumulation. Floyd et al. [32] showed that resveratrol treatment modulates insulin sensitivity by suppressing PPARγ gene and protein expression in 3T3-L1 adipocytes. The inhibition of PPARγ expression by resveratrol treatment leads to improved insulin sensitivity, which may be associated with decreased lipid accumulation and increased lipid oxidation [33,34]. Consistent with these results, the present study demonstrated that resveratrol-amplified grape skin extract treatment may inhibit adipocyte differentiation and modulate insulin sensitivity by suppressing PPARγ and C/EBPα transcriptional activity in 3T3-L1 adipocytes.
As a result of decreased PPARγ and C/EBPα gene expression, SREBP-1c also decreased significantly in resveratrol-amplified grape skin extract treated cells. Expression of the PPARγ and C/EBPα genes is associated with SREBP1c expression, which may influence lipogenesis [35,36]. SREBP1c, which is mainly expressed in adipose and liver tissues, is not only involved in fatty acid synthesis but also implicated in adipose tissue differentiation [37,38]. In the present study, inhibited SREBP1c mRNA expression was observed in resveratrol-treated cells compared with that in the control group. This result may explain the mechanism by which resveratrol suppresses fatty acid synthesis and adipocyte differentiation, and, thus, inhibits lipid accumulation.
We observed that resveratrol-amplified grape skin extracts also reduced FAS, aP2, and SCD-1 expression, which is associated with lipid metabolism. Activation of PPARγ, C/EBPα, and SREBP-1c induces upstream regulation of adipogenesis genes such as aP2, FAS, LPL, and SCD-1 [39-41]. aP2 is a marker of the late stage of differentiation in adipocytes and is involved in the control of lipid availability [42]. Additionally, aP2 may prevent atherosclerosis and metabolic syndrome by modulating insulin sensitivity and the immune response [43]. FAS and LPL play an important role regulating fatty acid metabolism. FAS, a terminal marker of adipocyte differentiation, is activated by PPAR and SREBP1c [44]. Because the level of LPL is related with triglyceride concentration in adipose tissues, upregulation of LPL activity is closely associated with obesity [45]. SCD-1 is an important regulator of leptin activity and is the rate limiting enzyme in monounsaturated fatty acid biosynthesis [46]. Cohen et al. [47] demonstrated that reduced SCD-1 activity may help prevent obesity by suppressing fatty acid biosynthesis and activating fatty acid oxidation. In this study, resveratrol-amplified grape skin extract treatment led to dose-dependent down-regulation of aP2, FAS, LPL, and SCD-1. Expression of adipogenesis gene in 80% ethanol extracts of resveratrol-amplified grape skin was down-regulated compared with that in 50% ethanol extracts. It may be related to increased resveratrol content in 80% ethanol extracts of resveratrol-amplified grape skin. Natural grape skin treatment to 3T3-L1 cells resulted in decrease in the adipogenic transcription factor gene and protein expression [48]. However, as the resveratrol content of grape skin is different according to strain, maturity of grape and sample preparation condition it is not understandable to compare the antiobesity effect quantitatively with the result of our study. Resveratrol increased by UV irradiation in the present study might enhance the antiobesity effect, since resveratrol decreased lipid accumulation in dose-dependent manner [25]. These results demonstrate that downregulating aP2, FAS, LPL, and SCD-1 by resveratrol-amplified grape skin extract treatment might reduce fatty acid synthesis and adipocyte differentiation, resulting in the inhibition of lipid accumulation by suppressing adipogenesis.
In conclusion, resveratrol-amplified grape skin extracts inhibited adipocyte differentiation by downregulating PPARγ and C/EBPα gene and protein expression in 3T3-L1 cells. Reduced PPARγ and C/EBPα gene expression may have contributed to the low expression of adipogenesis genes that regulate lipid synthesis and accumulation. The present results suggest that resveratrol-amplified grape skin extract exerts a beneficial effect on lipid metabolism to prevent obesity by attenuating adipogenic differentiation.
Figures and Tables
Fig. 1
Effect of the 50% (A) and 80% (B) resveratrol-amplified grape skin extracts on preadipocyte viability. 3T3-L1 preadipocytes were incubated with resveratrol-amplified grape skin extracts at various concentrations (0, 30, 60, 120, 250, 500, and 1,000 µg/ml) for 24 and 48 h. Mean ± SD (n = 3).
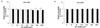
Fig. 2
Effect of the resveratrol-amplified grape skin extracts on inhibiting 3T3-L1 adipocyte differentiation. 3T3-L1 cells differentiated with differentiation media in the absence or presence of resveratrol-amplified grape skin extract (RAGE, 200 and 400 µg/ml) for 8 days. (A) Intracellular lipids were stained with Oil Red-O. The results were confirmed by three independent experiments. (B) Stained triglyceride content was quantified by measuring absorbance. Non, undifferentiated cells; DM, differentiated media cells. Values are mean ± SD (n = 3). Test for DM vs. Non: †††P < 0.001. Test for RAGE vs. DM: **P < 0.01, ***P < 0.001.
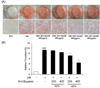
Fig. 3
Effect of resveratrol-amplified grape skin extracts on glycerol-3-phosphate dehydrogenase (GPDH) activity during 3T3-L1 adipocyte differentiation. Confluent 3T3-L1 preadipocytes were differentiated into adipocytes in medium with or without different concentrations of resveratrol-amplified grape skin extracts (RAGE) for 8 days. Non, undifferentiated cells; DM, differentiated media cells. Values are mean ± SD (n = 3). Test for DM vs. Non: †††P < 0.001. Test for RAGE vs. DM: **P < 0.01, ***P < 0.001.
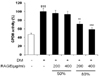
Fig. 4
Effect of resveratrol-amplified grape skin extracts on PPARγ and C/EBPα gene expression. Confluent 3T3-L1 preadipocytes were differentiated into adipocytes in medium with or without different concentrations of resveratrol-amplified grape skin extracts (RAGE) for 8 days. Non, undifferentiated cells; DM, differentiated media cells. Values are mean ± SD (n = 3). Test for DM vs. Non: †††P < 0.001. Test for RAGE vs. DM: **P < 0.01, ***P < 0.001.
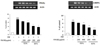
Fig. 5
Effect of resveratrol-amplified grape skin extracts on PPARγ and C/EBPα protein expression. Confluent 3T3-L1 preadipocytes were differentiated into adipocytes in medium with or without different concentrations of resveratrol-amplified grape skin extracts (RAGE) for 8 days. Non, undifferentiated cells; DM, differentiated media cells. Values are mean ± SD (n = 3). Test for DM vs. Non: †††P < 0.001. Test for RAGE vs. DM: **P < 0.01, ***P < 0.001.
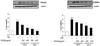
Fig. 6
Effect of resveratrol-amplified grape skin extracts on adipogenesis gene expression. Effects of the resveratrol-amplified grape skin extracts on adipogenic gene (SREBP1c, FAS, aP2, SCD-1, and LPL) expression during 3T3-L1 cell differentiation for 8 days. The results represent three independent experiments; actin was used as the internal control. Values are mean ± SD (n = 3). Test for DM vs. Non: ††P < 0.01, †††P < 0.001. Test for RAGE vs. DM: *P < 0.05, **P < 0.01, ***P < 0.001. DM, differentiated media cells; SREBP1c, adipocyte determination and differentiation dependent factor 1; aP2, adipocyte fatty acid binding protein; FAS, fatty acid synthetase; SCD-1, stearyl-CoA-desaturase-1; LPL, lipoprotein lipase.
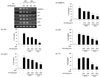
References
2. Das DK, Maulik N. Resveratrol in cardioprotection: a therapeutic promise of alternative medicine. Mol Interv. 2006. 6:36–47.


3. Lagouge M, Argmann C, Gerhart-Hines Z, Meziane H, Lerin C, Daussin F, Messadeq N, Milne J, Lambert P, Elliott P, Geny B, Laakso M, Puigserver P, Auwerx J. Resveratrol improves mitochondrial function and protects against metabolic disease by activating SIRT1 and PGC-1α. Cell. 2006. 127:1109–1122.


4. Filip V, Plockova M, Smidrkal J, Spickova Z, Melzoch K, Schmidt S. Resveratrol and its antioxidant and antimicrobial effectiveness. Food Chem. 2003. 83:585–593.


5. Bradamante S, Barenghi L, Villa A. Cardiovascular protective effects of resveratrol. Cardiovasc Drug Rev. 2004. 22:169–188.


6. Athar M, Back JH, Tang X, Kim KH, Kopelovich L, Bickers DR, Kim AL. Resveratrol: a review of preclinical studies for human cancer prevention. Toxicol Appl Pharmacol. 2007. 224:274–283.


7. de la Lastra CA, Villegas I. Resveratrol as an antioxidant and pro-oxidant agent: mechanisms and clinical implications. Biochem Soc Trans. 2007. 35:1156–1160.


8. Baur JA, Pearson KJ, Price NL, Jamieson HA, Lerin C, Kalra A, Prabhu VV, Allard JS, Lopez-Lluch G, Lewis K, Pistell PJ, Poosala S, Becker KG, Boss O, Gwinn D, Wang M, Ramaswamy S, Fishbein KW, Spencer RG, Lakatta EG, Le Couteur D, Shaw RJ, Navas P, Puigserver P, Ingram DK, de Cabo R, Sinclair DA. Resveratrol improves health and survival of mice on a high-calorie diet. Nature. 2006. 444:337–342.


9. Malterud K, Tonstad S. Preventing obesity: challenges and pitfalls for health promotion. Patient Educ Couns. 2009. 76:254–259.


10. Dewulf EM, Cani PD, Neyrinck AM, Possemiers S, Van Holle A, Muccioli GG, Deldicque L, Bindels LB, Pachikian BD, Sohet FM, Mignolet E, Francaux M, Larondelle Y, Delzenne NM. Inulin-type fructans with prebiotic properties counteract GPR43 overexpression and PPARγ-related adipogenesis in the white adipose tissue of high-fat diet-fed mice. J Nutr Biochem. 2011. 22:712–722.


11. Hosogai N, Fukuhara A, Oshima K, Miyata Y, Tanaka S, Segawa K, Furukawa S, Tochino Y, Komuro R, Matsuda M, Shimomura I. Adipose tissue hypoxia in obesity and its impact on adipocytokine dysregulation. Diabetes. 2007. 56:901–911.


12. Hao HD, He LR. Mechanisms of cardiovascular protection by resveratrol. J Med Food. 2004. 7:290–298.


13. Leifert WR, Abeywardena MY. Cardioprotective actions of grape polyphenols. Nutr Res. 2008. 28:729–737.


14. Bertelli AA, Das DK. Grapes, wines, resveratrol, and heart health. J Cardiovasc Pharmacol. 2009. 54:468–476.


15. Langcake P, Pryce RJ. The production of resveratrol and the viniferins by grapevines in response to ultraviolet irradiation. Phytochemistry. 1977. 16:1193–1196.


16. Cho YJ, Maeng JS, Kim CT, Pyee J. Enrichment of resveratrol content in harvested grape using modulation of cell metabolism with UV treatment. J East Asian Soc Diet Life. 2011. 21:739–745.
17. Singleton VL, Rossi JA Jr. Colorimetry of total phenolics with phosphomolybdic-phosphotungstic acid reagents. Am J Enol Vitic. 1965. 16:144–158.
18. Re R, Pellegrini N, Proteggente A, Pannala A, Yang M, Rice-Evans C. Antioxidant activity applying an improved ABTS radical cation decolorization assay. Free Radic Biol Med. 1999. 26:1231–1237.


19. Flegal KM, Carroll MD, Ogden CL, Curtin LR. Prevalence and trends in obesity among US adults, 1999-2008. JAMA. 2010. 303:235–241.


20. Lee CH, Olson P, Evans RM. Minireview: lipid metabolism, metabolic diseases, and peroxisome proliferator-activated receptors. Endocrinology. 2003. 144:2201–2207.


21. Harper CE, Patel BB, Wang J, Arabshahi A, Eltoum IA, Lamartiniere CA. Resveratrol suppresses prostate cancer progression in transgenic mice. Carcinogenesis. 2007. 28:1946–1953.


22. Bae HS. Effect of grape skin with resveratrol amplification on lipid metabolism and antioxidative system in rats fed high cholesterol diet [master's thesis]. 2008. Gyeongsan: Yeungnam University.
23. Wise LS, Green H. Participation of one isozyme of cytosolic glycerophosphate dehydrogenase in the adipose conversion of 3T3 cells. J Biol Chem. 1979. 254:273–275.


24. Park HJ, Yang JY, Ambati S, Della-Fera MA, Hausman DB, Rayalam S, Baile CA. Combined effects of genistein, quercetin, and resveratrol in human and 3T3-L1 adipocytes. J Med Food. 2008. 11:773–783.


25. Rayalam S, Yang JY, Ambati S, Della-Fera MA, Baile CA. Resveratrol induces apoptosis and inhibits adipogenesis in 3T3-L1 adipocytes. Phytother Res. 2008. 22:1367–1371.


26. Szatmari I, Rajnavolgyi E, Nagy L. PPARgamma, a lipid-activated transcription factor as a regulator of dendritic cell function. Ann N Y Acad Sci. 2006. 1088:207–218.


27. Zhang L, Chawla A. Role of PPARγ in macrophage biology and atherosclerosis. Trends Endocrinol Metab. 2004. 15:500–505.


28. Tontonoz P, Hu E, Spiegelman BM. Stimulation of adipogenesis in fibroblasts by PPARγ2, a lipid-activated transcription factor. Cell. 1994. 79:1147–1156.


29. Tang QQ, Zhang JW, Lane MD. Sequential gene promoter interactions of C/EBPβ, C/EBPα, and PPARγ during adipogenesis. Biochem Biophys Res Commun. 2004. 319:235–239.


30. Wu Z, Rosen ED, Brun R, Hauser S, Adelmant G, Troy AE, McKeon C, Darlington GJ, Spiegelman BM. Cross-regulation of C/EBPα and PPARγ controls the transcriptional pathway of adipogenesis and insulin sensitivity. Mol Cell. 1999. 3:151–158.


31. Yang JY, Della-Fera MA, Rayalam S, Ambati S, Hartzell DL, Park HJ, Baile CA. Enhanced inhibition of adipogenesis and induction of apoptosis in 3T3-L1 adipocytes with combinations of resveratrol and quercetin. Life Sci. 2008. 82:1032–1039.


32. Floyd ZE, Wang ZQ, Kilroy G, Cefalu WT. Modulation of peroxisome proliferator-activated receptor γ stability and transcriptional activity in adipocytes by resveratrol. Metabolism. 2008. 57:S32–S38.


33. Miles PD, Barak Y, He W, Evans RM, Olefsky JM. Improved insulin-sensitivity in mice heterozygous for PPAR-gamma deficiency. J Clin Invest. 2000. 105:287–292.
34. Chen LL, Zhang HH, Zheng J, Hu X, Kong W, Hu D, Wang SX, Zhang P. Resveratrol attenuates high-fat diet-induced insulin resistance by influencing skeletal muscle lipid transport and subsarcolemmal mitochondrial β-oxidation. Metabolism. 2011. 60:1598–1609.


35. Fajas L, Schoonjans K, Gelman L, Kim JB, Najib J, Martin G, Fruchart JC, Briggs M, Spiegelman BM, Auwerx J. Regulation of peroxisome proliferator-activated receptor γ expression by adipocyte differentiation and determination factor 1/sterol regulatory element binding protein 1: implications for adipocyte differentiation and metabolism. Mol Cell Biol. 1999. 19:5495–5503.


36. Payne VA, Au WS, Lowe CE, Rahman SM, Friedman JE, O'Rahilly S, Rochford JJ. C/EBP transcription factors regulate SREBP1c gene expression during adipogenesis. Biochem J. 2010. 425:215–223.


37. Le Lay S, Lefrère I, Trautwein C, Dugail I, Krief S. Insulin and sterol-regulatory element-binding protein-1c (SREBP-1C) regulation of gene expression in 3T3-L1 adipocytes. Identification of CCAAT/enhancer-binding protein beta as an SREBP-1C target. J Biol Chem. 2002. 277:35625–35634.
38. Gosmain Y, Dif N, Berbe V, Loizon E, Rieusset J, Vidal H, Lefai E. Regulation of SREBP-1 expression and transcriptional action on HKII and FAS genes during fasting and refeeding in rat tissues. J Lipid Res. 2005. 46:697–705.


39. Bennett MK, Lopez JM, Sanchez HB, Osborne TF. Sterol regulation of fatty acid synthase promoter. Coordinate feedback regulation of two major lipid pathways. J Biol Chem. 1995. 270:25578–25583.
40. Kim JB, Spiegelman BM. ADD1/SREBP1 promotes adipocyte differentiation and gene expression linked to fatty acid metabolism. Genes Dev. 1996. 10:1096–1107.


41. Horton JD, Shimomura I, Brown MS, Hammer RE, Goldstein JL, Shimano H. Activation of cholesterol synthesis in preference to fatty acid synthesis in liver and adipose tissue of transgenic mice overproducing sterol regulatory element-binding protein-2. J Clin Invest. 1998. 101:2331–2339.


42. Makowski L, Brittingham KC, Reynolds JM, Suttles J, Hotamisligil GS. The fatty acid-binding protein, aP2, coordinates macrophage cholesterol trafficking and inflammatory activity. Macrophage expression of aP2 impacts peroxisome proliferator-activated receptor gamma and IkappaB kinase activities. J Biol Chem. 2005. 280:12888–12895.


43. Makowski L, Hotamisligil GS. Fatty acid binding proteins--the evolutionary crossroads of inflammatory and metabolic responses. J Nutr. 2004. 134:2464S–2468S.


44. Kong CS, Kim JA, Ahn BN, Vo TS, Yoon NY, Kim SK. 1-(3',5'-dihydroxyphenoxy)-7-(2",4",6-trihydroxyphenoxy)-2,4,9-t rihydroxydibenzo-1,4-dioxin inhibits adipocyte differentiation of 3T3-L1 fibroblasts. Mar Biotechnol (NY). 2010. 12:299–307.


45. Bulló M, García-Lorda P, Peinado-Onsurbe J, Hernández M, Del Castillo D, Argilés JM, Salas-Salvadó J. TNFα expression of subcutaneous adipose tissue in obese and morbid obese females: relationship to adipocyte LPL activity and leptin synthesis. Int J Obes Relat Metab Disord. 2002. 26:652–658.


46. Cohen P, Friedman JM. Leptin and the control of metabolism: role for stearoyl-CoA desaturase-1 (SCD-1). J Nutr. 2004. 134:2455S–2463S.

