This article has been corrected. See "Erratum: Lutein decreases oxidative stress and inflammation in liver and eyes of guinea pigs fed a hypercholesterolemic diet" in Volume 7 on page 146.
Abstract
Guinea pigs were fed a hypercholesterolemic diet (0.25 g/100 g cholesterol) and randomly allocated either to a Control group (n = 9) or to a Lutein (0.1 g/100 g) group (n = 10) for 12 weeks to evaluate oxidative stress and inflammation in both liver and eyes. Malondialdehyde (MDA) concentrations and inflammatory cytokines were measured as well as hepatic nuclear factor-kappaB (NF-κB) binding. Lutein concentrations were greater in eyes (P < 0.01) and liver (P < 0.001) in the Lutein group. All guinea pigs had high concentrations of hepatic cholesterol as well as high plasma ALT and AST levels indicative of liver injury. However, the Lutein group had 43% lower hepatic free cholesterol than the Controls (P < 0.05). Hepatic MDA and MDA in the eye were lower in the Lutein compared to the Control group (P < 0.05). Hepatic tumor necrosis factor-α was 32% lower in the Lutein group (P < 0.05). Lastly, the Lutein group presented lower NF-κB DNA binding activity than the Control group (P < 0.001). These results suggest that in the presence of high cholesterol, lutein exerts both antioxidant and anti-inflammatory effects, which can be explained by attenuated NF-κB DNA binding activity. Furthermore, results also suggest that lutein accumulates in the eyes of guinea pigs to protect against oxidative stress.
The carotenoid lutein is preferentially accumulated in the macular region of the human retina where it is known to protect the eyes against light damage [1], preventing the development of degenerative conditions in the eye including age-related macular degeneration (AMD) [2]. Retinal inflammatory reaction is suggested as a pathogenic factor in most retinal diseases including AMD [3,4]. Furthermore, oxidative stress is known to contribute to retinal inflammatory reactions. In an experimental animal model, oxidative damage in the outer retina and induction of inflammation contribute to the development of AMD [4]. However, whether the administration of lutein reduces the level of reactive oxygen species (ROS) in eyes and has a protective effect during inflammatory states remains to be elucidated.
Recent studies have also suggested that lutein can reduce atherosclerosis and exert cardiac protection [5,6]. However, there are not sufficient reports on the effects of lutein on other degenerative conditions, such as liver injury, and its underlying mechanisms. A small number of in vivo studies have suggested that this carotenoid may protect the liver against oxidation [7,8]. In the aspect of anti-inflammatory effects, previous studies have shown that lutein inhibited lipopolysaccharide (LPS)-induced systemic inflammation in animal models and in RAW 264.7 macrophages [9,10]. A recent study also reported that dietary lutein decreased interleukin (IL)-1β mRNA levels in the liver of F-line turkeys challenged with LPS injection [11]. The mechanism by which lutein down-regulates inflammatory responses in the liver has not been fully investigated. However, several studies have suggested an association between nuclear factor-kappaB (NF-κB) signaling pathway and a wide variety of pathological conditions in the liver [12-14]. Based on these observations, it is possible that the mechanism by which lutein attenuates the inflammatory state in the liver may be mediated through NF-κB pathways.
The guinea pig is a suitable animal model to study hepatic cholesterol and lipoprotein metabolism because of similar responses to humans in regards to dietary interventions [15]. High cholesterol diets (0.25%) have been shown to increase hepatic cholesterol and triglyceride accumulation compared to low cholesterol diets (0.04%) [16,17]. This excess lipid accumulation in the liver may lead to the development of non-alcoholic fatty liver disease (NAFLD) [18]. We have previously demonstrated that lutein decreases cholesterol accumulation, oxidative damage and inflammation in aortas of guinea pigs [19]. Therefore, we hypothesized that lutein accumulation in the eyes of guinea pigs could protect against oxidative stress and inflammation. Further we tested the hypothesis that lutein could prevent degenerative conditions in the liver of guinea pigs fed with high cholesterol diet by reducing lipid peroxidation and attenuating pro-inflammatory cytokine production.
Nineteen male Hartley guinea pigs (Charles River Breeding Laboratories, Wilmington MA) were randomly assigned into either the Lutein group or the Control group. All animals were fed a high cholesterol diet (0.25 g/100 g) for 12 weeks, while the Lutein group was also given lutein supplementation (0.1 g/100 g) (FloraGLO® Lutein, Kemin Industries, Inc., USA). The percent energy distribution of the diet was 42:35:22 for carbohydrate: fat:protein and the micronutrient composition was formulated to meet the National Research Council requirements for guinea pigs [20]. Animal protocols were approved by the Institutional Animal Care and Use Committee of the University of Connecticut protocol number A08-070. After 12 hours of food depravation, guinea pigs were killed and the livers and eyes were harvested. The tissues samples were promptly frozen in liquid nitrogen and then stored in -80℃ until analyses.
Approximately 0.5g of liver or 500 uL of plasma were prepared for carotenoid analysis by saponification and extraction with hexane and ethyl ether. Samples were prepared as previously reported [21].
Two whole eyes were pooled for each animal and homogenized in 1mL PBS with a tissue tearor (Model 985370-07, BioSpec Products, Inc., Bartlesville, OK). An equal volume of tetrahydrofuran (THF) was added to the homogenate and vortexed then, 5 mL of ethyl ether were added and the mixture was vortexed and sonicated for 3 min at 3 watts RMS. After centrifugation at 1,000 × g for 10 min, the top organic layer was transferred to a second glass vial. The bottom phase was extracted again with 5 mL of ethyl ether and the organic phases of two extractions were combined. Solvents in the extract were evaporated under nitrogen gas. In preparation for high performance liquid chromatography (HPLC) analysis, the samples were re-dissolved with 1 mL of ethanol and analyzed as previously reported [21].
After total lipids extraction with chloroform-methanol (2:1), hepatic total cholesterol (Roche-Diagnostics, Indianapolis, IN), free cholesterol (Wako Chemicals USA, Inc., Richmond, VA) and triglyceride (Roche-Diagnostics, Indianapolis, IN) were determined according to Carr et al. [22] by using enzymatic reagents. Hepatic esterified cholesterol concentrations were calculated by subtracting free cholesterol from total cholesterol.
Photometric measurements were used to measure plasma aspartate aminotransferase (AST) and alanine aminotransferase (ALT) by using Cobas c 111 analyzer (Roche-Diagnostics, Indianapolis, IN).
Liver and eye MDA concentrations were measured by thiobarbituric acid reactive substances (TBARS) assay using a commercially available assay kit (Cayman Chemical, Ann Arbor, MI). In brief, 100 µL of homogenized liver and eye tissues suspended in RIPA buffer or 100 µL standards were added to 100 µL of SDS solution and 4 mL of color reagent. Vials were placed into vigorously boiling water for one hour and immediately removed and placed in ice for 10 min to stop the reaction. Samples were centrifuged for 10 min at 1,600 × g at 4℃ and incubated at room temperature for 30 min. Samples (150 µL) and standards were read by fluorescence at an excitation wavelength of 530 nm and an emission wavelength of 550 nm. Detected MDA were normalized to protein concentrations, which were measured using a commercially available Bradford assay (Bio-Rad Laboratories; Hercules, CA, USA).
Protein levels of TNF-α, IL-1β and monocyte chemotactic protein-1 (MCP-1) were evaluated from homogenates of liver and eye using the MILLIPLEX MAP Mouse Cytokine PREMIXED Immunoassay kit (Millipore coorporation, Charles, MO, USA) and Luminex system (Luminex 200 System, Austin, TX) as previously reported [19].
The nuclear fraction was extracted using Nuclear Extract kit (Active Motif, Carlsbad, CA). In brief, 100 mg of frozen liver tissue were ground using a cold mortar and pestle. The ground tissue samples were suspended in 300 µL of 1X stock hypotonic buffer and then transferred to a dounce homogenizer and dounced on ice approximately 20 strokes. Homogenized tissues were incubated on ice 15 minutes and then centrifuged at 850 × g for 10 minutes at 4℃. The pellet containing nuclei was re-suspended in 50 µL 1X stock hypotonic solution and after 15 minutes incubation on ice, 2.5 µL detergents were added to disrupt nuclear membrane. Samples were centrifuged at 14,000 × g for 30 sec at 4℃ and then the nuclear pellet was re-suspended in 50 µl complete lysis buffer (5 µL 10 mM DTT, 45 µL lysis buffer AM1, 0.5 µL protease inhibitor cocktail). To extract the nuclear proteins, samples were incubated on ice for 30 minutes, after vortexing, they were centrifuged at 14,000 × g for 10 minutes at 4℃ to remove insoluble material.
DNA-binding activity of p65 was assessed using an NF-κB p65 EZ-TFA transcription factor assay colorimetric kit (Millipore, Danvers, MA). Briefly, 50 µL of transcription factor assay (TFA) buffer containing 12.5 µg of nuclear extract and 2 µL of NF-κB capture probe were added to a well and incubated for 2 h. Primary NF-κB antibody was added and incubated for 1 h, after which wells were washed 3 times with an enhanced TFA buffer. IgG-HRP conjugated secondary antibody was added and after 30 minutes incubation, wells were washed 4 times with a TFA buffer. To develop color, the TMB/E substrate was added and after 10 min incubation, the plate was read by a spectrophotometric microplate reader (Bio-Tek, Winooski, VT) at 450 nm. Positive and negative controls were also analyzed to validate this assay.
Independent t-tests were used for comparisons between groups and bivariate correlations were used when appropriate. Statistical analyses were conducted by using SPSS 17.0 (SPSS, Chicago, IL) and the values reported as mean ± standard deviation (SD). Values with P < 0.05 were considered to be significant.
Eye lutein concentrations of guinea pigs from the Lutein group were 2.87 ± 0.98 ng/eye and this value was significantly higher than the Control group (1.03 ± 0.69 ng/eye) (P < 0.01) Hepatic lutein concentrations were 23.8 ± 5.6 nmol/g in the Lutein group while the concentrations was < 1 nmol/g in the Control group. Plasma lutein concentrations in the Lutein group were 75.1 ± 17.1 nmol/L compared to < 5 nmol/L in the Control group.
There were no significant differences in liver weight between the Lutein and the Control group (data not shown). Similarly, hepatic total cholesterol and triglyceride concentrations were not different between groups (Table 1). However, the percent distribution of free cholesterol in hepatic total cholesterol was significantly lower whereas the percent distribution of cholesterol ester was significantly higher in the Lutein group (31.5 ± 25.5% and 68.5 ± 25.5%) compared to those of the Control group (57.5 ± 20.7% and 42.5 ± 20.7%) (P < 0.05). Plasma values for ALT and AST were not different between groups. However, both ALT and AST levels were positively correlated with percent distribution of free cholesterol in the liver (r = 0.57; P < 0.025 and r = 0.66; P < 0.001, respectively) (Fig. 1).
Guinea pigs from the Lutein group had lower hepatic MDA concentrations (6.0 ± 1.0 nmol/mg protein) than the Control group (7.0 ± 1.0 nmol/mg protein) (P < 0.05) (Fig. 2). Hepatic lutein concentration was negatively correlated with hepatic MDA concentration (r = -0.47, P < 0.05). The eyes from the Lutein group also presented significantly lower MDA concentration (3.08 ± 1.43 nmol/mg protein) than those from the Control group (6.20 ± 3.80 nmol/mg protein) (P < 0.05). In addition, eye lutein concentrations were negatively associated with eye MDA concentration (r = -0.63, P < 0.05) (data now shown).
Guinea pigs from the Lutein group had significantly lower hepatic concentrations of TNF-α (338.9 ± 100.7 pg/mg protein) than the Control guinea pigs (499.9 ± 182.5 pg/mg protein, P < 0.05) (Fig. 3A). There were no significant differences in MCP-1 (509.4 ± 100.2 pg/mg protein vs 623.3 ± 152.8 pg/mg protein, P = 0.069) or IL-1β (614.5 ± 125.5 pg/mg protein vs 668.1 ± 173.1 pg/mg protein) concentrations in the liver between groups.
In the eye, the Lutein group showed significantly lower TNF-α (125.5 ± 14.1 pg/mg protein vs 138.9 ± 9.6 pg/mg protein, P < 0.05) and IL-1β (323.6 ± 41.4 pg/mg protein vs 413.8 ± 128.0 pg/mg protein P < 0.05) (Fig. 3B) concentrations than the Control group. However, there were no significant differences in MCP-1 concentration (P = 0.321) between groups.
Along with lower TNF-α concentrations in the liver, the Lutein group also presented 18.3% lower NF-κB p65 DNA binding activity than the Control group (P < 0.0001) (Fig. 4).
The present study demonstrates that guinea pigs with lutein treatment successfully accumulate this carotenoid in both liver and eyes. In addition, lutein exerts antioxidant and anti-inflammatory effects. Furthermore, lutein treatment protects against degenerative conditions in the liver of guinea pigs fed with high cholesterol diet by reducing hepatic free cholesterol, decreasing lipid peroxidation and attenuating the inflammatory responses known to contribute to the development of NAFLD. Data also indicate that the attenuated inflammatory response with lutein treatment was due to the decreased NF-κB p65 DNA binding activity. However, interestingly, there were no differences in ALT and AST concentrations between groups, two major indicators of liver injury.
A previous study conducted with guinea pigs identified lutein in both eyes and liver after feeding the animals lutein at the supplemental level of 7.97 mg/kg diet [23]. Based on this result, we decided to use 0.1 g/100 g lutein to ensure not only the presence of this carotenoid in eye and liver but also to prove its protective effects on these tissues. Consistent with this study [24], we successfully detected lutein in both eyes and liver.
Hepatic lipid overloading has been considered the first hit in NAFLD development [18] and high cholesterol diet treated guinea pigs present greater hepatic total cholesterol and triglyceride accumulation than those fed a low cholesterol diet [17]. In this study, we observed greater lipid accumulation in guinea pig liver compared to those treated with low concentrations of dietary cholesterol [24]. It is important to note that there were no differences in hepatic total cholesterol and triglyceride concentrations between the two groups in the present study. Consistent with these results, it is reported that fat content of liver was independent of amounts of dietary lutein in Japanese quail [25]. However the Lutein group presented significantly lower percent distribution of free cholesterol in hepatic total cholesterol compared to the Control group. Additionally, percent distribution of free cholesterol presented strong positive correlations with both plasma ALT and AST levels. Therefore, the larger portion of free cholesterol in the Control group may increase the risk of liver injury. Lipid composition of human liver demonstrated almost 2-fold increase in free cholesterol content in subjects with NAFLD or nonalcoholic steatohepatitis (NASH) compared to normal subjects [26]. It is suggested that several abnormalities that can be observed in NAFLD including mitochondrial abnormalities and impaired adenosine triphosphate synthesis may potentially contribute to the increase in hepatic free cholesterol contents [27,28].
Oxidative stress also has been considered as an important etiological factor in many progressive liver diseases [29]. The excessive lipid peroxidation caused by free radicals leads to oxidative stress, which results in the accumulation of MDA. MDA is one of the end products of lipid peroxidation and oxidative stress [30]. It is well known that lutein is a beneficial antioxidant due to its structural characteristics consisting in centrally localized conjugated C = C double bonds, which can readily quench reactive oxygen species [31]. Additionally, hydroxyl groups attached to each of the 2 terminal β, ε-ionone rings make lutein scavenge free radicals more efficiently than other carotenoids [32]. Collectively, the antioxidant effects of lutein may attenuate the oxidative stress and the production of MDA. In the present study, the lutein treated guinea pigs presented lower MDA production in liver compared to the control guinea pigs. In addition, hepatic lutein concentrations were negatively correlated with hepatic MDA concentrations, which clearly indicates that lutein may inhibit lipid peroxidation.
Liver injury by pro-inflammatory cytokines is another major factor to induce liver disease [18]. In particular, NF-κB, a major transcriptional factor involved in cell survival, immunity and inflammation, is activated during liver injury [12]. NF-κB signaling pathway and subsequent pro-inflammatory cytokine production regulate the maintenance of liver homoeostasis as well as the pathogenesis of a wide variety of liver diseases, including viral hepatitis, steatohepatitis, cirrhosis and hepatocellular carcinoma [14]. It has been shown that lutein directly decreases NF-κB activation and suppresses both mRNA and protein levels of cyclooxygenase (COX)-2, inducible nitric oxide synthase (iNOS), TNF-α, and IL-1β. Peroxisome proliferator X receptor (PPAR) and retinoic acid X receptor (RXR) are members of nuclear hormone receptors and they regulate NF-κB signaling pathway and inflammatory cytokine production [33]. One recent study suggested that LPS-induced PPARα and RXRα down-regulation was partially reversed by increasing the dietary lutein content to 50 mg/kg in chickens [34]. Therefore, based on these studies, an anti-inflammatory effect of lutein may be explained by direct inhibition of NF-κB activation or PPARα/RXRα-mediated regulation. Consistent to these studies, we found that guinea pigs from the Lutein group presented significantly lower hepatic TNF-α concentration than guinea pigs from the Control group. In agreement with our study, mice with D-galactose-induced liver injury also showed that 40 mg/kg/day of lutein treatment lowers iNOS content in the liver [35].
Although lutein treatment inhibited lipid peroxidation and the inflammatory state in guinea pigs fed a high cholesterol diet, there were no differences on liver enzyme concentrations in the plasma between two groups. Serum ALT concentrations have been associated with decreased serum antioxidant concentrations, particularly carotenoids [36]. Additionally, Several animal studies suggest that lutein lowers ALT and AST concentrations due to scavenging of reactive oxygen radicals in rats with paracetamol-, carbon tetrachloride- and ethanol-induced hepatic injury [7,37]. However, these studies used higher amount of lutein (50, 100, 250 mg/day) than in the current investigation (30 mg/day).
The retina in the eyes is extremely rich in membranes with polyunsaturated fatty acids and this characteristic makes the eye susceptible to lipid peroxidation [38]. Not only by filtering the blue light, which is the most damaging wavelength of light that reaches the retina [39] but also by readily quenching singlet oxygen species [40], lutein protects the eye from oxidative stress. In the current study, lutein was successfully detected in the eyes of guinea pigs with lutein treatment and they presented lower concentrations of MDA compared to guinea pigs without lutein treatment. We also observed lower pro-inflammatory cytokine productions with lutein treatment in the eye and consistent with our study, pro-inflammatory cytokine productions in the aqueous humor of rats with endotoxin-induced uveitis were reduced with 100 mg/kg lutein administration [9]. This result was explained by suppression of NF-κB activation with lutein, therefore lutein might be involved in the regulation NF-κB signaling pathway in the eye as well.
In summary, our findings suggest that lutein might have antioxidant and anti-inflammatory functions in the eye. Additionally, this carotenoid could prevent degenerative conditions of the liver by reducing the free cholesterol pool and attenuating lipid peroxidation and pro-inflammatory cytokine production. Further, attenuated inflammatory state in the liver could be explained by decreased NF-κB p65 binding activity.
Figures and Tables
Fig. 1
Correlations between AST and free cholesterol (r = 0.57, P < 0.025) (Panel A) and ALT and free cholesterol (r = 0.66, P < 0.001) for 19 guinea pigs (n = 9, control and n = 10 for the lutein group). ALT and AST were determined by use of a COBAS C-111 analyzer.
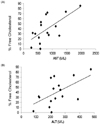
Fig. 2
Malonaldehyde concentrations in liver and in eye of guinea pigs fed control (n = 9) or lutein (n = 10) diets. MDA concentrations were measured by thiobarbituric acid reactive substances (TBARS) *indicates P < 0.05.
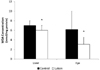
References
1. Khachik F, Beecher GR, Smith JC Jr. Lutein, lycopene, and their oxidative metabolites in chemoprevention of cancer. J Cell Biochem Suppl. 1995. 22:236–246.


2. Krinsky NI, Landrum JT, Bone RA. Biologic mechanisms of the protective role of lutein and zeaxanthin in the eye. Annu Rev Nutr. 2003. 23:171–201.


3. Donoso LA, Kim D, Frost A, Callahan A, Hageman G. The role of inflammation in the pathogenesis of age-related macular degeneration. Surv Ophthalmol. 2006. 51:137–152.


4. Hollyfield JG, Bonilha VL, Rayborn ME, Yang X, Shadrach KG, Lu L, Ufret RL, Salomon RG, Perez VL. Oxidative damage-induced inflammation initiates age-related macular degeneration. Nat Med. 2008. 14:194–198.


5. Dwyer JH, Navab M, Dwyer KM, Hassan K, Sun P, Shircore A, Hama-Levy S, Hough G, Wang X, Drake T, Merz CN, Fogelman AM. Oxygenated carotenoid lutein and progression of early atherosclerosis: the Los Angeles atherosclerosis study. Circulation. 2001. 103:2922–2927.


6. Dwyer JH, Paul-Labrador MJ, Fan J, Shircore AM, Merz CN, Dwyer KM. Progression of carotid intima-media thickness and plasma antioxidants: the Los Angeles Atherosclerosis Study. Arterioscler Thromb Vasc Biol. 2004. 24:313–319.


7. Sindhu ER, Firdous AP, Preethi KC, Kuttan R. Carotenoid lutein protects rats from paracetamol-, carbon tetrachloride- and ethanol-induced hepatic damage. J Pharm Pharmacol. 2010. 62:1054–1060.


8. Sindhu ER, Preethi KC, Kuttan R. Antioxidant activity of carotenoid lutein in vitro and in vivo. Indian J Exp Biol. 2010. 48:843–848.
9. Jin XH, Ohgami K, Shiratori K, Suzuki Y, Hirano T, Koyama Y, Yoshida K, Ilieva I, Iseki K, Ohno S. Inhibitory effects of lutein on endotoxin-induced uveitis in Lewis rats. Invest Ophthalmol Vis Sci. 2006. 47:2562–2568.


10. Kim JH, Na HJ, Kim CK, Kim JY, Ha KS, Lee H, Chung HT, Kwon HJ, Kwon YG, Kim YM. The non-provitamin A carotenoid, lutein, inhibits NF-kappaB-dependent gene expression through redox-based regulation of the phosphatidylinositol 3-kinase/PTEN/Akt and NF-kappaB-inducing kinase pathways: role of H(2)O(2) in NF-kappaB activation. Free Radic Biol Med. 2008. 45:885–896.


11. Shanmugasundaram R, Selvaraj RK. Lutein supplementation alters inflammatory cytokine production and antioxidant status in F-line turkeys. Poult Sci. 2011. 90:971–976.


12. He G, Karin M. NF-kappaB and STAT3 - key players in liver inflammation and cancer. Cell Res. 2011. 21:159–168.


13. Luedde T, Schwabe RF. NF-kappaB in the liver--linking injury, fibrosis and hepatocellular carcinoma. Nat Rev Gastroenterol Hepatol. 2011. 8:108–118.


14. Robinson SM, Mann DA. Role of nuclear factor kappaB in liver health and disease. Clin Sci (Lond). 2010. 118:691–705.
15. Fernandez ML. Guinea pigs as models for cholesterol and lipoprotein metabolism. J Nutr. 2001. 131:10–20.


16. Roy S, Vega-Lopez S, Fernandez ML. Gender and hormonal status affect the hypolipidemic mechanisms of dietary soluble fiber in guinea pigs. J Nutr. 2000. 130:600–607.


17. Torres-Gonzalez M, Shrestha S, Sharman M, Freake HC, Volek JS, Fernandez ML. Carbohydrate restriction alters hepatic cholesterol metabolism in guinea pigs fed a hypercholesterolemic diet. J Nutr. 2007. 137:2219–2223.


18. Anstee QM, Goldin RD. Mouse models in non-alcoholic fatty liver disease and steatohepatitis research. Int J Exp Pathol. 2006. 87:1–16.


19. Kim JE, Leite JO, DeOgburn R, Smyth JA, Clark RM, Fernandez ML. A lutein-enriched diet prevents cholesterol accumulation and decreases oxidized LDL and inflammatory cytokines in the aorta of guinea pigs. J Nutr. 2011. 141:1458–1463.


20. Fernandez ML, McNamara DJ. Regulation of cholesterol and lipoprotein metabolism in guinea pigs mediated by dietary fat quality and quantity. J Nutr. 1991. 121:934–943.


21. Clark RM, Herron KL, Waters D, Fernandez ML. Hypo- and hyperresponse to egg cholesterol predicts plasma lutein and beta-carotene concentrations in men and women. J Nutr. 2006. 136:601–607.


22. Carr TP, Andresen CJ, Rudel LL. Enzymatic determination of triglyceride, free cholesterol, and total cholesterol in tissue lipid extracts. Clin Biochem. 1993. 26:39–42.


23. Schäffer MW, Sinha Roy S, Mukherjee S, Das SK. Identification of lutein, a dietary antioxidant carotenoid in guinea pig tissues. Biochem Biophys Res Commun. 2008. 374:378–381.


24. Lin EC, Fernandez ML, McNamara DJ. Dietary fat type and cholesterol quantity interact to affect cholesterol metabolism in guinea pigs. J Nutr. 1992. 122:2019–2029.


25. Shanmugasundaram R, Selvaraj RK. Dietary lutein and fish oil interact to alter atherosclerotic lesions in a Japanese quail model of atherosclerosis. J Anim Physiol Anim Nutr (Berl). 2011. 95:762–770.


26. Puri P, Baillie RA, Wiest MM, Mirshahi F, Choudhury J, Cheung O, Sargeant C, Contos MJ, Sanyal AJ. A lipidomic analysis of nonalcoholic fatty liver disease. Hepatology. 2007. 46:1081–1090.


27. Sanyal AJ, Campbell-Sargent C, Mirshahi F, Rizzo WB, Contos MJ, Sterling RK, Luketic VA, Shiffman ML, Clore JN. Nonalcoholic steatohepatitis: association of insulin resistance and mitochondrial abnormalities. Gastroenterology. 2001. 120:1183–1192.


28. Nair S, V PC, Arnold C, Diehl AM. Hepatic ATP reserve and efficiency of replenishing: comparison between obese and nonobese normal individuals. Am J Gastroenterol. 2003. 98:466–470.


29. McClain CJ, Mokshagundam SP, Barve SS, Song Z, Hill DB, Chen T, Deaciuc I. Mechanisms of non-alcoholic steatohepatitis. Alcohol. 2004. 34:67–79.


30. Janero DR. Malondialdehyde and thiobarbituric acid-reactivity as diagnostic indices of lipid peroxidation and peroxidative tissue injury. Free Radic Biol Med. 1990. 9:515–540.


31. Young AJ, Lowe GM. Antioxidant and prooxidant properties of carotenoids. Arch Biochem Biophys. 2001. 385:20–27.


32. Ribaya-Mercado JD, Blumberg JB. Lutein and zeaxanthin and their potential roles in disease prevention. J Am Coll Nutr. 2004. 23:567S–587S.


33. Debril MB, Renaud JP, Fajas L, Auwerx J. The pleiotropic functions of peroxisome proliferator-activated receptor gamma. J Mol Med (Berl). 2001. 79:30–47.


34. Selvaraj RK, Shanmugasundaram R, Klasing KC. Effects of dietary lutein and PUFA on PPAR and RXR isomer expression in chickens during an inflammatory response. Comp Biochem Physiol A Mol Integr Physiol. 2010. 157:198–203.


35. Mai J, Shen X, Shi D, Wei Y, Shen H, Wu M. Effect of lutein on relieving oxidative stress in mice induced by D-galatose. Wei Sheng Yan Jiu. 2010. 39:430–432.
36. Ruhl CE, Everhart JE. Relation of elevated serum alanine aminotransferase activity with iron and antioxidant levels in the United States. Gastroenterology. 2003. 124:1821–1829.


37. Preethi KC, Kuttan R. Hepato and reno protective action of Calendula officinalis L. flower extract. Indian J Exp Biol. 2009. 47:163–168.
38. Catala A. Lipid peroxidation of membrane phospholipids in the vertebrate retina. Front Biosci (Schol Ed). 2011. 3:52–60.

