Abstract
The purpose of the present study was to investigate the effect of ethanol extracts from red pepper seeds on the antioxidative defense system and oxidative stress in rats fed a high fat · high cholesterol diet. Rats were divided into four experimental groups which were composed of high fat · high cholesterol diet group (HF), high fat · high cholesterol diet with 0.1% ethanol extracts from red pepper seeds supplemented group (HEA), high fat · high cholesterol diet with 0.2% ethanol extracts from red pepper seeds supplemented group (HEB) and high fat·high cholesterol diet with 0.5% ethanol extracts from red pepper seeds supplemented group (HEC). Supplementation of ethanol extracts from red pepper seeds groups (HEA, HEB and HEC) resulted in significantly increased activities of hepatic glutathione peroxidase and catalase. Hepatic superoxide radical contents in microsome and mitochondria were significantly reduced in the groups supplemented with red pepper seeds ethanol extracts. Hepatic hydrogen peroxide content in the mitochondria was reduced in ethanol extracts from red pepper seeds supplemented groups. TBARS values in the liver were reduced in red pepper seeds ethanol extracts supplemented groups. Especially, HEB and HEC groups were significantly decreased compared to the HF group. Hepatic carbonyl values were significantly reduced in mitochondria in these supplemented groups. These results suggest that red pepper seeds ethanol extracts may reduce oxidative damage, by activation of antioxidative defense system in rats fed high fat · high cholesterol diets.
Currently, the westernization of dietary lifestyle is followed by chronic adult diseases including hyperlipidemia, arteriosclerosis, diabetes and hypertension. This is likely because of various factors, such as environmental pollution and lack of exercise. Oxidative stress provoked by imbalance between free radicals and antioxidative defense system is a major cause of various oxidative diseases, such as cancer, inflammation, heart disease, brain disease, decreased immunity and aging [1]. Reactive oxygen species (ROS) are generated as free radicals in the body such as superoxide anion, hydroxyl radical, and hydrogen peroxide, and known to induce many adult disease, as well as to promote the aging process. The human body uses the free radical scavenger system as a physiological defense system to protect tissues from peroxidative damage by free radicals and lipid peroxides. This defense system is divided into a non-enzyme defense system (vitamin E, β-carotene, etc.) and an enzyme defense system (superoxide dismutase (SOD), glutathione peroxidase (GSHpx), catalase, etc.) [2]. It is said that in normal physiological condition these defense systems protect the body from oxidative damages, however, in a pathological condition like diabetes, the free radical scavenger system can become weak whereas the free radical production system is promoted resulting in an imbalance between the systems [3]. Therefore, attention to the intake of antioxidants in order to repress oxidative stress and to prevent various diseases and aging has increased [4]. Antioxidative substances are widely distributed in animals and plants. Antioxidants such as phenol chemical compounds, flavone derivatives, tocopherol, ascorbic acid and selenium in fruits and vegetables play a key role in the inhibition of aging by delaying or preventing the oxidation of fats, cancer and cardiovascular diseases [5]. Being a food containing such bioactive materials, hot pepper, an indispensable spice of Korean diet, can be referred to representatively. Hot pepper contains phenolic compounds such as ascorbic acid, capsanthin, capsorubin, cryptocapsin, quarcetin, luteolin and capsaicinoid [6], many of which have been reported as being antioxidants [7]. Pepper seeds oil has about 68~72% linoleic acid, 13~15% palmitic acid, and 9~11% oleic acid [8]. However, researches on the antioxidative effect of pepper seeds have been rarely conducted, although some studies have investigated the antimutation and anti-cancer effect of the red pepper seeds [9]. Especially red pepper seed has antimutagenic effects against the aflatoxin B1 and MNNG, and the anticarcinogenic effects in human cancer cells have been reported to be high [10]. Therefore, this study was carried out to investigated the effect of ethanol extracts from red pepper seed on antioxidative system and oxidative damage in the high fat · high cholesterol rat.
The red pepper seed was purchased from the Namandong Agriculture cooperative. Air-dried red pepper seed was crushed using a grinder (Desung power mixer/grinder DA-280G, Seoul, Korea) and shattered pepper seeds were used in samples by cold storage. Grinded pepper seeds and six times 100% ethanol were mixed and then extraction was performed at 4℃ for 24 hours. This process was repeated three times and the supernatant was collected and filtered with a Whatman No.1 filter paper. The solvent of combined extracts was evaporated under reduced pressure using a rotary vacuum evaporator (HS-2001N, Hanshin Science Co., Korea) and dried in a freeze drier (Bondiro, Ilshin, Korea).
Male Sprague-Dawley rats weighing 100 ± 10 g were purchased from KRITC (Daejeon, Korea). Rats were individually housed in stainless steel cages in a room with controlled temperature (20~23℃) and lighting (alternating 12 h periods of light and dark). Rats were divided into four high fat · high cholesterol groups which were composed of HF group (red pepper seeds ethanol extract free), HEA group (0.1 g red pepper seeds ethanol extract/100 g diet), HEB group (0.2 g red pepper seeds ethanol extract/100 g diet), and HEC group (0.5 g red pepper seeds ethanol extract/100 g diet) (Table 1). The rats fed the experimental diets for a period of 4 weeks. The experimental design was approved by the committee of Korea International University regarding the care and use of laboratory animals.
Sprague-Dawley rat liver was removed and washed with ice-cold 9 g/l NaCl. A rat liver homogenate was prepared according to the described method of Slater and Sawyer [11]. The liver was homogenized in Hepes buffer (mmol/l N-2-hydroxyethypiperazine-N'-2-ethanesulfonic acid, 10 mmol/l KCl and 280 mmol/l sucrose, pH 7.4), centrifuged at 3,000 rpm for 15 min, recentrifuged at 9,000 rpm for 20 min to remove any mitochondrial contamination, and then centrifuged at 41,500 rpm for 60 min to yield the microsomal fraction. The supernatant was further centrifuged at 105,000 g for 602 min, at 4℃. Protein in the liver tissues was determined using method of Lowry et al. [12].
Results were analyzed by ANOVA and Tukey's honestly significant difference test [20]. Statistical significance is defined as P < 0.05.
Table 2 shows the activity of SOD, an antioxidant enzyme which reduces superoxide radicals to H202, which in turn is excreted as H20, based on the activity of GSHpx and CAT, thereby protecting the body from oxygen toxicity. The result of SOD activity in the liver was greater in the HEA, HEB and HEC groups than in the HF group. However, these differences were not significant among the experimental groups. The GSHpx activity in the HEA, HEB and HEC groups were significantly greater than that of the HF group. The CAT activity in the HEA, HEB and HEC groups were 104%, 138% and 152% greater, respectively, than that in the HF group. The HEB and HEC groups were significantly increased compared to the HF group.
The hepatic superoxide radical content is shown in Fig. 1. The liver microsomal superoxide radical contents in the HEA, HEB and HEC groups were significantly lower than that of the HF group. As well, the hepatic mitochondrial superoxide radical contents in the HEA, HEB and HEC groups were 35%, 42% and 53% significantly lower, respectively, than that in the HF group. The result of hepatic mitochondria fraction of hydrogen peroxide content is shown in Fig. 2. The content of hydrogen peroxide in the liver mitochondria of the HEC group was significantly lower than that of the HF group.
The result of TBARS contents as an index oxidative damage is shown in Fig. 3. These TBARS concentrations, an index of lipid peroxidation, were 83%, 74% and 63% lower in HEA, HEB and HEC groups, respectively, compared with the HF groups. The HEB and HEC groups were significantly decreased compared to the HF group. The liver microsomal carbonyl values (Fig. 4) in the HEA, HEB and HEC groups were lower than that in the HF group, but these differences were not significant among the experimental groups. The liver mitochondrial carbonyl values in the HEA, HEB and HEC groups were 34%, 35% and 37% lower, respectively, than that in the HF group. However, there were no significant differences among the red pepper seed extracts supplemented groups.
The purpose of the present study was to investigate the effect of ethanol extracts from red pepper seeds on antioxidative system and oxidative stress of liver in rats fed high fat · high cholesterol diet. Oxidative stress means the production of highly reactive oxygen radicals that are toxic to the cells, particularly to the cell membrane in which these radicals interact with the lipid bilayer and produce lipid peroxides. Endogenous antioxidant enzymes (e.g, SOD, GSHpx and CAT) are responsible for the detoxification of deleterious oxygen radicals. Superoxide dismutase is concerned with the elimination of 02˙-(superoxide) that develops from natural substances and is retained in the body. SOD activity is necessary to restrain this development as free radical oxidation is an obstacle and enemy to the body [21,22]. The SOD activity in the liver was greater in the HEA, HEB and HEC groups than in the HF group. During the reduction of hydrogen peroxide, GSH is converted to an oxidized form, glutathione disulfide (GSSG), and GSSG is reduced back to GSH by NADPH-dependant glutathione reductase [23,24]. Rocha et al. [25] showed that hepatic GSH tended to be higher in high fat-fed rats than in standard diet-fed rats. From the current result of observing the activities of the antioxidative defense system in the liver, the GSHpx activity in the HEA, HEB and HEC groups was significantly greater than that in the HF group. The CAT activity in the HEA, HEB and HEC groups was 104%, 138% and 152% greater, respectively, than that in the HF group. These results were considered to be caused by polyphenols within the pepper seeds. The phenolic hydroxyl of the phenolic compounds distributed widely in plants was bound well to macromolecules such as zymoproteins, and these compounds have been recognized as substances showing such bioactivity [26]. In a study of Ku et al. [27], the total polyphenol content of the ethanol extracts of the pepper seeds was 8.00 ± 0.57~33.99 ± 0.08mg/g. The content was higher than those of seeds and nuts, for instance, reported by Lee and Lee [28] in their study examining the total polyphenol contents of vegetative foods, showing 27 mg/g of sesame seeds, 0.83 mg/g of perilla seeds, 0.12 mg/g of apricot stones, 2.06 mg/g of walnuts, 0.13 mg/g of pumpkin seeds and 2.02 mg/g of sunflower seeds. Antioxidative substances obtained from natural materials are mainly phenolic compounds and flavonoids. Caffeic acid and chlorogenic acid have been reported to have a strong antioxidant capacity [29]. ROS was known to damage cells by producing lipid peroxides and by acting on polyunsaturated fatty acids of cellular membranes [30]. ROS concentration was significantly decreased in the group supplied with ethanol extracts of pepper seeds compared to the HF group, which is thought to be due to antioxidative activity by the supply of the pepper seeds causing inactivated or eliminated free radicals [31]. This result was in line with the finding of Sim and Han who reported that a greater content of red pepper seeds leads to higher electron donating ability using DPPH [32].
Ki and Han reported that superoxide radical is an effective free radical scavenger and such effect is also contained in the red pepper seed [21]. Lipid peroxidation caused by free radicals following oxidative stress in a cell has been known to produce metabolic abnormalities in an organism and to provoke cancer, mutation, loss of genes and aging, by damaging DNA [33]. The TBARS concentrations, an index of lipid peroxidation, were lower in HEA, HEB and HEC groups, compared with the HF group. Especially, HEB and HEC groups were significantly decreased compared to the HF group. Several studies have also shown elevated lipid peroxidation products in high fat diet [34,35]. High intake of dietary fat directly enhanced ROS overproduction which increased lipid peroxidation [36]. This decrease in the lipid peroxides in the liver tissue resulted from red pepper seed extract activating the function of removing free radicals, indicating that the antioxidative defense system was activated to reduce the formation of peroxides. The carbonyl value concentrations, an index of protein peroxidation, were 34%, 35% and 37% lower in HEA, HEB and HEC groups, respectively, compared with the HF group. This result was considered to be the action of antioxidative substances such as polyphenols contained by ethanol extracts of the seed, which suppressed oxidation of proteins by inhibiting lipid peroxidation and finally repressing the oxidation of tissues. In conclusion, an ethanol extract of red pepper seed strengthened the antioxidative defense system with increased activity of SOD, GSHpx and CAT in the liver of high fat · high cholesterol diet fed rats. Accordingly, ethanol extract of red pepper seed was found to reduce the accumulation of ROS, such as superoxide radical and H202, which decrease the generation of oxidatively damaging substances such as TBARS and the carbonyl value.
Figures and Tables
Fig. 1
Effects of ethanol extracts from red pepper seeds on hepatic superoxide radical contents in rats fed high fat · high cholesterol diets. Values are the means ± SE (n=10). Those with different superscript letters are significantly different at P < 0.05 by Tukey's test. The experimental groups are the same as in Table 1.
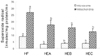
Fig. 2
Effects of ethanol extracts from red pepper seeds on mitochondrial hydrogen peroxide (H2O2) contents in rats fed high fat · high cholesterol diets. Values are the means ± SE (n=10). Those with different superscript letters are significantly different at P < 0.05 by Tukey's test. The experimental groups are the same as in Table 1.
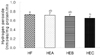
Fig. 3
Effects of ethanol extracts from red pepper seeds on hepatic thiobarbituric acid reactive substances (TBARS) values in rats fed high fat · high cholesterol diets. Values are the means ± SE (n=10). Those with different superscript letters are significantly different at P < 0.05 by Tukey's test. The experimental groups are the same as in Table 1.
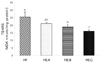
Fig. 4
Effects of ethanol extracts from red pepper seeds on hepatic carbonyl value in rats fed high fat · high cholesterol diets. Values are the means ± SE (n=10). Those with different superscript letters are significantly different at P < 0.05 by Tukey's test. The experimental groups are the same as in Table 1. NS : not significant
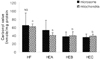
Table 1
Compositions of diets in experiment group (g/kg diet)
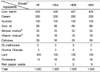
1)HF: high fat · high cholesterol diet, HEA: high fat · high cholesterol diet + 0.1% red pepper seeds ethanol extract, HEB: high fat · high cholesterol diet + 0.2% red pepper seeds ethanol extract, HEC: high fat · high cholesterol diet + 0.5% red pepper seeds ethanol extract
2)AIN-76 mineral mixture (g/kg mixture)
3)AIN-76 vitamin mixture (g/kg mixture)
Table 2
Effects of ethanol extracts from red pepper seeds on hepatic superoxide dismutase (SOD), glutathione peroxidase (GSH-px) and catalase (CAT) activities in rats fed high fat · high cholesterol diets
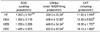
Values are the means ± SE (n=10). Those with different superscript letters are significantly different at P < 0.05 by Tukey's test. The experimental groups are the same as in Table 1.
NS : not significant
References
1. Halliwell B, Gutteridge JMC. Free radicals, ageing, and disease. Free radicals in biology and medicine. 1996. London. England: Clarendon Press Publishing Co;416.
2. Hammers HD, Martin S, Fedesrlin K, Geisen K, Brownlee M. Aminoguanidine treatment inhibit the development of experimental diabetic retinopathy. Proc Natl Acad Sci U S A. 1991. 8:11555–11558.
3. Park GY, Rhee SJ, Im JG. Effects of green tea catechin on cytochrome P450, xanthine oxidase activities in liver and liver damage in streptozocin induced diabetic rats. J Korean Soc Food Sci Nutr. 1997. 26:901–907.
4. Lenaz G. Role of mitochondria in oxidative stress and ageing. Biochim Biophys Acta. 1998. 1366:53–67.


5. Block G, Langseth L. Antioxidant vitamins and disease prevention. Food Technol. 1994. 48:80–85.
6. Manjeshwar SB, Ganesh CJ, Shaial KR, Kiran BS. Evaluation of nitric oxide scavenging activity of certain spices in vitro: A preliminary study. Nahrung. 2003. 47:261–264.


7. Hasler CM, Kundrat S, Wool D. Functional foods and cardiovascular disease. Curr Atheroscler Rep. 2000. 2:467–475.


8. Lee KJ, Han JS, Lee SW, Park CR. Investigation of Lipids in Hot Pepper I. Neutral lipids of hot pepper seeds. Korean Journal of Food Science and Technology. 1975. 7:91–95.
9. Sim KH, Han YS. The antimutagenic and antioxidant effects of red pepper and red pepper pericarp (Capsicum annum L.). Journal of Food Science and Nutrition. 2007. 12:273–278.


10. Morimoto C, Tsujita T, Okuda H. Ephinephrine-induced lipolysis in rats fat cell from visceral and subcutaneous sites: role of hormone-sensitive and lipid droplets. J Lipid Res. 1997. 38:132–138.


11. Slater TF, Sawyer BC. The Stimulatory effects of carbon tetrachloride and other halogenoalkanes on peroxidative reactions on rat liver fractions in vivo. Biochem J. 1971. 123:805–814.


12. Lowry OH, Roseborough NJ, Ferr AL, Randall RJ. Protein measurement with the folin phenol reagent. J Biol Chem. 1951. 193:265–275.


13. Marklund S, Marklund G. Involvement of the superoxide anion radical in the autoxidation of pyrogallol and a convenient assay for superoxide dismutase. Eur J Biochem. 1974. 47:469–474.


14. Lawrence RA, Burk RF. Glutathione peroxidase activity in selenium deficient rat liver. Biochem Biophys Res Commun. 1976. 71:952–958.
15. Aebi H, Wyss SR, Scherz B, SKvaril F. Heterogeneity of erythrocyte catalase. Isolation and characterixation of normal and variant erythrocyte catalase and their subunits. Eur J Biochem. 1974. 48:137–145.


16. Azzi A, Montecucco C, Richter C. The use of acetylated ferric cytochrome c for the detection of superoxide radicals produced in biological membranes. Biochem Biophy Res Commun. 1975. 65:597–603.


17. Gay C, Gebicki JM. A critical evaluation of the effect of sorbitol on the ferric-xylenol orange hydroperoxide assay. Anal Biochem. 2000. 284:217–220.


18. Levin RL, Garland D, Oliver CN, Amici A, Climent I, lenz AG, Ahn B, Shatiel S, Stadtman ER. Determination of carbonyl content intoxidatively modified proteins. Meth Enzymol. 1990. 186:464–478.
19. Satoh K. Serum lipid peroxide in cerebrovascular disorders determined by a new colorimetric method. Clin Chim Acta. 1978. 90:37–43.


20. Sreel RGD, Torrie JH. Principles and procedures of statistics. 1990. New York: McGrow Hill.
21. Ki HS, Han YS. Antioxidant activities of red pepper (Capsicum annuum) pericarp and seed extracts. International Journal of Food Science & Technology. 2008. 43:1813–1823.


22. Lee YJ, Han JP. Antioxidant activities and nitrite scavenging abilities of extracts from Ulmus devidianan. J Korean Soc Food Sci Nutr. 2000. 29:893–899.
24. Lee SJ, Choi SK, Seo JS. Grape skin improves antioxidant capacity in rats fed high fat diet. Nutr Res Pract. 2009. 3:279–285.


25. Rocha KK, Souza GA, Ebaid GX, Seivia FRF, Cataneo AC, Novelli ELB. Resveratrol toxicity: Effects on risk factors for atherosclerosis and hepatic oxidative stress in standard an high-fat diets. Food Chem Toxicol. 2009. 47:1362–1367.


26. Park SY, Bok SH, Jeon SM, Park YB, Lee SJ, Jeong TS, Choi MS. Effect of rutin and tannic acid supplements on cholesterol metabolism in rats. Nutr Res. 2002. 22:283–295.


27. Ku KH, Choi EJ, Park WS. Functional Activity of Water and Ethanol Extracts from Red Pepper (Capsicum annuum L.) Seeds. Journal of the Korean Society of Food Science and Nutrition. 2008. 37:1357–1362.


28. Lee JH, Lee SR. Analysis of phenolic substances content in Korean plant foods. Journal of the Korean Society of Food Science and Nutrition. 1994. 26:310–316.
29. Cuvelier ME, Richard H, Berset C. Antioxidative activity and phenolic composition of pilot-plant and commercial extracts of sage and rosemary. J Am Oil Chem Soc. 1996. 73:645–652.


30. Jimenez-Escrig A, Rincon M, Pulido , Saura-Clixto F. Guava fruit (Psidium guajava L.) as a new source of antioxidant dietary fiber. J Agric Food Chem. 2001. 49:5489–5493.


31. Fridovich I. Superoxide dismutase. An adaptation to a paramagnetic gas. J Biol Chem. 1989. 264:7761–7764.
32. Sim KH, Han YS. Effect of red pepper seed on Kimchi antioxidant activity during fermentation. Food Sci Biotechnol. 2008. 17:295–301.
33. Sohal RS, Allen RG. Oxidative stress as a causal factor indifferentiation and aging: A unifying hypothesis. Exp Gerontol. 1990. 25:499–522.


34. Feillet-Coudray C, Sutra T, Fouret G, Ramos J, Wrutniak-Cabello C, Cabello G, Cristol JP, Coudray C. Oxidative stress in rats fed a high-fat high-sucrose diet and preventive effect of polyphenols: Involvement of mitochondrial and NAD(P)H oxidase systems. Free Radic Biol Med. 2009. 46:624–632.


35. Fan J, Zeng M, Li J. Correlation between hepatic fat, lipid peroxidation and hepatic fibrosis in rats chronically fed with ethanol and/or high fat diet. Zhonghua Nei Ke Za Zhi. 1997. 36:808–811.