Abstract
Figures and Tables
Table 1

Control: control diet, B6-: vitamin B6 deficient diet
BW: body weight, FER: food efficiency ratio =total weight gain/total food intake, PLP: pyridoxal-5-phosphate
*Significant difference between control group and B6-group, P < 0.05 (t-test), NS: no significant difference between control group and B6-group
Table 2

Control: control diet, B6-: vitamin B6 deficient diet
PreE: pre-exercise, PostE: post-exercise, recessE: recess after exercise
SOD: superoxide dismutase in liver cytosol, GSH/GSSG: the ratio of reduced glutathione and oxidized glutathione in liver cytosol
Values in the same row with different superscript symbols (a, b) is significantly different, P < 0.05, NS: no significant difference among exercised groups
*Significant difference between control group and B6-group, P < 0.05 (t-test), NS: no significant difference between control group and B6-group
Table 3
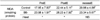
Control: control diet, B6-: vitamin B6 deficient diet
PreE: pre-exercise, PostE: post-exercise, recessE: recess after exercise
MDA: malondialdehyde in liver cytosol
Values in the same row with different superscript symbols (a, b) are significantly different, P < 0.05.
*Significant difference between control group and B6-group, P < 0.05 (t-test), NS: no significant difference between control group and B6-group
Table 4

Control: control diet, B6-: vitamin B6 deficient diet
PreE: pre-exercise, PostE: post-exercise, recessE: recess after exercise
TG: Triglyceride, TC: total cholesterol, HDL-C: high density lipoprotein cholesterol, Atherosclerotic index = (TC-HDL-C)/HDL-C
Values in the same row with different superscript symbols (a, b) is significantly different, P < 0.05, NS: no significant difference among exercised groups
*Significant difference between control group and B6-group, P < 0.05 (t-test), NS: no significant difference between control group and B6-group
References














