Abstract
BACKGROUND/OBJECTIVE
Oxidative stress plays a key role in neuronal cell damage, which is associated with neurodegenerative disease. The aim of present study was to investigate the neuroprotective effects of perilla oil (PO) and its active component, alpha-linolenic acid (ALA), against hydrogen peroxide (H2O2)-induced oxidative stress in SH-SY5Y neuronal cells.
MATERIALS/METHODS
The SH-SY5Y human neuroblastoma cells exposed to 250 µM H2O2 for 24 h were treated with different concentrations of PO (25, 125, 250 and 500 µg/mL) and its major fatty acid, ALA (1, 2.5, 5 and 25 µ/mL). We examined the effects of PO and ALA on H2O2-induced cell viability, lactate dehydrogenase (LDH) release, and nuclear condensation. Moreover, we determined whether PO and ALA regulated the apoptosis-related protein expressions, such as cleaved-poly ADP ribose polymerase (PARP), cleaved caspase-9 and -3, BCL-2 and BAX.
RESULTS
Treatment of H2O2 resulted in decreased cell viability, increased LDH release, and increase in the nuclei condensation as indicated by Hoechst 33342 staining. However, PO and ALA treatment significantly attenuated the neuronal cell death, indicating that PO and ALA potently blocked the H2O2-induced neuronal apoptosis. Furthermore, cleaved-PARP, cleaved caspase-9 and -3 activations were significantly decreased in the presence of PO and ALA, and the H2O2-mediated up-regulated BAX/BCL-2 ratio was blocked after treatment with PO and ALA.
CONCLUSIONS
PO and its main fatty acid, ALA, exerted the protective activity from neuronal oxidative stress induced by H2O2. They regulated apoptotic pathway in neuronal cell death by alleviation of BAX/BCL-2 ratio, and down-regulation of cleaved-PARP and cleaved caspase-9 and -3. Although further studies are required to verify the protective mechanisms of PO and ALA from neuronal damage, PO and ALA are the promising agent against oxidative stress-induced apoptotic neuronal cell death.
Accumulation of reactive oxygen species (ROS) has long been involved in disrupting cellular function, leading to cell death via apoptosis [1]. One of the well-known ROS, hydrogen peroxide (H2O2) is extensively used as a source of oxidative stress, due to its ability to easily cross the cell membrane and to convert into the highly reactive hydroxyl radical (·OH) through Fenton reaction. It reacts with nearly all cellular molecules resulting in their damage, thereby inducing apoptosis in various cell types [2]. In particular, compared to other tissues, the brain is susceptible to oxidative stress because of a high rate of oxidative metabolism [3]. Neuronal cell death induced by oxidative stress leads to progression of neurodegenerative disorders including Alzheimer's disease (AD) [4]. The human neuroblastoma SH-SY5Y cell has been commonly used as a cellular model for screening therapeutic agents having neuroprotective effects [56].
Recently, attention has increased in the search for natural sources that can protect neuronal cell damage against oxidative stress. Alpha-linolenic acid (ALA, C18:3) is one of the essential fatty acids rich in vegetable oils; especially, perilla oil (PO) contains a large amount of ALA (approximately 60%). Since ALA cannot be synthesized in humans due to a deficiency of the n-3 fatty acid desaturase, the prerequisite needs to be obtained through diet [7]. ALA is a principal precursor for eicosapentaenoic acid (EPA, C20:5) and docosahexaenoic acid (DHA, C22:6), which are abundantly found in fish oil. Previous studies have suggested that intake of n-3 polyunsaturated fatty acids correlates with decrease in coronary heart diseases, inflammatory diseases and some cancers [89]. Although there are evidences that dietary n-3 fatty acids have beneficial effects, the focus has mainly been on fish oil. ALA is another promising n-3 fatty acid found in vegetable oils, and having beneficial roles in health. However, the study on the molecular mechanism between ALA and H2O2-induced neuronal apoptosis has not been studied yet.
A hallmark of AD, amyloid beta (Aβ), has been implicated to play a key role in oxidative stress and neurotoxicity [10]. Previously, we reported that PO [11] and ALA [12] improved the cognitive ability in AD mice. Administration of PO and ALA significantly reversed the Aβ-induced learning and memory impairment as measured by T-maze, novel object recognition and Morris water maze tests. Furthermore, PO and ALA significantly reduced malondialdehyde (MDA) and nitric oxide (NO) levels in Aβ-injected mice brain tissue. Therefore, we speculated that the cognitive improvement against Aβ could be mediated by the anti-oxidative activity of PO and ALA. Previous studies demonstrated that PO and ALA ameliorate the oxidative stress by the inhibiting lipid peroxidation or regulating nuclear factor kappa B [131415]. In the rat brain, H2O2, which is generated from the superoxide anion (O2−), induces mitochondrial dysfunction resulting in cell death [16]. The SH-SY5Y cells are highly sensitive to oxidative stress such as H2O2. Therefore, we investigated the protective effects of PO and ALA (a major fatty acid isolated from PO), on H2O2-induced oxidative stress in SH-SY5Y neuronal cells, and the regulatory mechanisms involved of the apoptosis pathway.
PO was generously donated by the Southern Area Crop Science, Rural Development Administration (Miryang, Korea). The fatty acid composition of PO used in this study was ALA (515.20 mg/g), linoleic acid (141.16 mg/g), oleic acid (147.05 mg/g), stearic acid (23.08 mg/g) and palmitic acid (52.12 mg/g) [17]. ALA was extracted from PO after treatment with urea and cooling, using a high-yield method as described previously [18]. PO and ALA were prepared as a stock solution in dimethyl sulfoxide (DMSO), and the final concentration was adjusted with DMSO before use; the vehicle (DMSO) did not affect cell viability. The PO and ALA were stored in a refrigerator at 4℃ with nitrogen gas, for stability.
Fetal bovine serum (FBS), Dulbecco's modified eagle's medium (DMEM), and penicillin/streptomycin were supplied from Welgene (Daegu, Korea) for cell culture. H2O2 was purchased from Junsei chemical Co. (Tokyo, Japan). Radioimmunoprecipitation assay (RIPA) buffer, 30% acrylamide bis solution, and pre-stained protein size marker were obtained from Elpis Biotech (Daejeon, Korea). Polyvinylidene fluoride (PVDF) membrane was from Millipore (Billerica, MA, USA). Primary and secondary antibodies were purchased from Cell Signaling (Beverly, MA, USA).
SH-SY5Y cells were obtained from KCLB (Korean Cell Line Bank, Seoul, Korea). The cells were maintained at 37℃ in a 5% CO2 incubator, in DMEM supplemented with 1% penicillin/streptomycin and 10% FBS. Cells were sub-cultured with 0.05% trypsin-EDTA and phosphate buffered saline (PBS).
Confluent cells were harvested and plated at a density of 5 × 104 cells/mL, into 96 well plates. After overnight culture, the cells were treated with varying concentrations of PO (25, 125, 250 and 500 µg/mL) and ALA (1, 2.5, 5 and 25 µg/mL) for 30 min. The treated cells were then exposed to H2O2 and incubated 24 h. After incubation, cell viability was determined using the MTS (Promega, Madison, WI, USA) as described previously [19] according to the manufacturer's protocol. Absorbance of each well was read at 490 nm.
LDH activity was measured using the LDH cytotoxicity detection kit (Takara Bio, Shiga, Japan), according to the manufacturer's instructions. SH-SY5Y cells were plated at a density of 5 × 104 cells/mL in 96 well plates, and cultured overnight. Cells were treated with PO (25, 125, 250 and 500 µg/mL) and ALA (1, 2.5, 5 and 25 µg/mL) for 30 min, and they were exposed to H2O2 following 24 h. The supernatant (100 µL) and reaction mix (100 µL) were mixed in 96 well plates and incubated for 30 min at room temperature. All samples were measured using a microplate reader at absorbance 490 nm [20].
SH-SY5Y cells were seeded on 8 well chamber slides and treated with PO and ALA for 30 min. Cells were exposed to 250 µM H2O2 for 24 h. After removal of the medium, cells were washed with PBS (pH 7.4) and fixed in 4% paraformaldehyde for 10 min. The fixed cells were again washed with PBS and treated with Hoechst 33342 (NucBlue® Live Ready Probes® Reagent, Life Technologies, Grand Island, NY, USA) for 20 min. Cells were observed using the Fluorescence microscope (Olympus BX50, Tokyo, Japan). Apoptotic bodies of cells with characteristic nuclear fragmentation and condensation were counted (at least 300 cells). Four pictures from different fields were randomly chosen, and data were expressed as a percentage of apoptotic cells with respect to the total cell number [212223].
SH-SY5Y cell extracts were prepared according to the manufacturer's instructions, using RIPA buffer supplemented with 1× protease inhibitor cocktail. Proteins were separated by electrophoresis in a precast 10–13% sodium dodecyl sulfate polyacrylamide gel, and blotted onto PVDF membranes. The membrane was blocked with 5% skim milk solution for 1 h at room temperature, and incubated overnight at 4℃ with primary antibody (cleaved caspase-3, cleaved caspase-9, poly ADP ribose polymerase (PARP), BAX, BCL-2, and β-actin; each antibody at a dilution of 1:1000). Following washing, the membranes were incubated with the appropriate horseradish peroxidase-conjugated secondary antibodies. Western bands were visualized using a chemiluminescent imaging system (Davinci Chemi, Seoul, Korea).
To determine the neuroprotective effects of PO and ALA against oxidative stress in SH-SY5Y cells, the viability was measured by MTS assay. As illustrated in Fig. 1A, 250 µM H2O2 treatment decreased the cell viability to 65.85% compared with control group (100%). However, PO treatment significantly increased the cell viability. In particular, treatment with 500 µg/mL restored cell viability to 95.45%. Likewise, H2O2-treated vehicle group had a reduced cell viability of 60.76%, whereas an increase in cell viability was observed after ALA treatment, being 62.77% and 66.68% at 5 and 25 µg/mL concentrations, respectively (Fig. 1B).
The inhibitory effects of PO and ALA on H2O2-induced cytotoxicity were evaluated by measuring the LDH levels. As shown in Fig. 2A, the SH-SY5Y cells treated with H2O2 for 24 h expressed 53.49% LDH release, as compared to the un-treated group (2.59%). However, pre-incubation with PO significantly attenuated the LDH activity. Especially, PO at 500 µg/mL markedly decreased LDH release to 38.68%. In addition, treatment of ALA (25 µg/mL) also effectively reduced the H2O2-induced LDH release from 41.22% to 25.52%, indicating that PO and ALA protect against H2O2-mediated SH-SY5Y cell death (Fig. 2B).
The protective effects of PO and ALA were further investigated on H2O2-induced morphological changes in SH-SY5Y neuronal cells, using Hoechst 33342 staining. We observed increased nuclear condensation and bright blue color in the H2O2-treated vehicle group, as compared to the control group (Fig. 3A and B). In contrast, the SH-SY5Y cells treated with PO (500 µg/mL) and ALA (25 µg/mL) revealed attenuation of the H2O2-induced neuronal cell death. These results indicate that PO and ALA protect H2O2-induced SH-SY5Y cell death via inhibition of apoptosis.
To investigate the effects of PO and ALA on the underlying apoptotic pathway, we focused on evaluating the regulation of PARP and caspase activation. Treatment of 250 µM H2O2 resulted in enhancing the levels of cleaved PARP, and cleaved caspase-3 and -9. In contrast, treatment of SH-SY5Y cells with PO (500 µg/mL) and ALA (5 and 25 µg/mL) significantly attenuated these protein expression levels (Fig. 4,5,6). These results indicate that PO and ALA inhibit downstream of the apoptotic pathway, including PARP and caspase-9 and -3 activations.
We further investigated the effects of PO and ALA on BAX/BCL-2 expression ratio. In the H2O2-induced vehicle group, the expression of BCL-2 protein was significantly down-regulated, whereas the protein level of BAX was significantly up-regulated (Fig. 7). Our results showed a high BAX to BCL-2 ratio, demonstrating that H2O2-induced apoptosis. As compared to the vehicle group, treatment with PO and ALA led to decreased expression of the pro-apoptotic protein BAX, with simultaneous increased levels of the anti-apoptotic protein BCL-2.
Oxidative stress in brain is associated with neurodegenerative diseases [24]. Excessive production of ROS, such as O2−, ·OH and H2O2, induces protein oxidation, lipid peroxidation, and dysfunction of mitochondria and DNA, leading to neuronal cell death. H2O2 is known to significantly decrease the cell viability via the oxidative-dependent apoptotic process. Therefore, H2O2-induced neuronal cell death in SH-SY5Y cells is extensively used as a cellular model to investigate the apoptosis caused by oxidative stress. Many studies have reported that dietary modifications block the apoptosis-pathway and protect neuronal cell death, thereby resulting in the prevention or delaying of AD [252627].
The neurological health benefits of EPA and DHA supplements are well established [28]. Recently, concerns have arisen regarding fish, the main source of EPA and DHA, involving the geographical restriction in certain countries or heavy metal pollution in sea water. However, ALA is abundantly found in vegetable oils, and this has increased the attention in the functional effects of n-3 fatty acid derived from plants. Consumption of PO, which is the most abundant ALA, is a good alternative to increase the intake of n-3 fatty acid. Chung [29] demonstrated that consumption of ALA has a cardioprotective effect, and reported increased concentrations of both EPA and DPA in the plasma of PO-fed mice model. Although ALA is a precursor of EPA, and consequently DPA and DHA, it is not efficient. In fact, ingestion of ALA does not accelerate EPA or DHA levels, but only serves as a calorie source [30]. This was explained in multiple studies which report the extremely low bioconversion efficiency from ALA to EPA (0.4%) and DHA (less than 0.1%) [31]. Considering this point, Blondeau and Tauskela [32] demonstrated the direct protective effect of ALA on neurons against glutamate-mediated toxicity. We therefore speculated that in oxidative stress-induced SH-SY5Y cells, ALA may have a neuroprotective effect mediated by direct action. To investigate the protective effects of PO and ALA against oxidative stress-induced neuronal cell apoptosis, we evaluated the cell viability, LDH release, DNA condensation and signaling pathway involved in neuronal apoptosis.
The concentration of ALA in our study was based on composition rate in PO and physiological effect described in previous reports; (1) A bioavailability study has shown that treatment of PO 100–800 µg/mL displayed an anti-thrombotic activity [33]. In addition, PO at dose of 50 mg/mL reversed Aβ toxicity by attenuating oxidative stress in PC12 neuronal cells [34]. Our previous data also showed that 25–500 µg/mL of PO has radical scavenging effect in vitro [35]. (2) Generally, PO consists of about 60% ALA. The 25–500 µg/mL of PO may result in approximately 15–300 µg/mL of ALA. Treatment of ALA in concentration of 1–25 µg/mL to SH-SY5Y cells did not affect cellular conditions in our preliminary study, thus we decided to assess the neuroprotective effect of ALA at a concentration range of 1–25 µg/mL. In agreement with previous studies [3637], we observed that SH-SY5Y cells exposed to H2O2 treatment resulted in cell loss. However, treatment with PO and ALA prevented the cell death, as confirmed by the cell viability assay. Furthermore, PO and ALA treatment significantly decreased the LDH activity, indicating a cytoprotective effect against H2O2-induced oxidative stress. A number of studies have established that n-3 fatty acid contained in oils plays a role in reducing the oxidative stress [1438]. We also previously reported that ALA from PO exerted ·OH scavenging activity in vitro [35]. In this study, the protective effect of ALA against H2O2-induced oxidative stress was also observed, indicating that ALA is a promising agent as a free radical scavenger or antioxidant. The LDH leakage assay is based on the release of the enzyme after cell membrane damage, so it requires only a few minutes to measure signal. On the other hand, the MTS assay is mainly based on mitochondria activity, and other features such as reaction time and metabolic conditions affect the results [39]. According to Wang et al. [40], metabolic activity of cells may be affected by different conditions, chemicals or phytochemicals, which can cause change of interaction with MTT or MTS directly. There are several factors determining the cell function or viability such as enzyme activity, cell membrane permeability, cell adherence, nucleotide uptake activity as well as ATP production. Although, the effect of ALA on cell viability is seemed to be minimal compared to the effects on LDH release and apoptotic signaling pathway, other assays are required to evaluate the effect of ALA on cell proliferation and function.
Neuronal apoptotic cell death is characterized to cell shrinkage and DNA condensation, as well as morphological changes [414243]. The Hoechst 33342 staining method, which discriminates the live and apoptotic cells by showing brightly stained nuclei, was performed in order to observe apoptosis morphologically. Microscopic imaging showed that H2O2 treatment strongly induced neuronal cell death, whereas the PO- and ALA-treated cells showed decrease in chromatin condensation and cell shrinkage. These results indicate that PO and ALA inhibited the cellular morphological changes against H2O2-induced cell damage.
There are two classes of apoptotic regulatory proteins involved in the apoptosis pathway: the anti-apoptotic proteins (BCL-2, BCL-XL) and pro-apoptotic proteins (BAX, BID, BCLXS) [44]. In apoptotic processes, the expression of anti-apoptotic BCL-2 protein (inhibits cell death) is significantly reduced, and the pro-apoptotic BAX protein (promotes cell death) is significantly increased [45]. During initiation of apoptosis, BAX is translocated to the mitochondrial membrane and induces the activation of caspases, which is responsible for cell death [4647]. To further investigate whether pro-apoptotic and anti-apoptotic protein expressions were affected by PO and ALA treatment, we analyzed the ratio of BAX/BCL-2 expression. In agreement with a previous study, our results showed enhancement of the BAX/BCL-2 ratio in SH-SY5Y cells incubated with H2O2 for 24h, whereas treatment with PO and ALA suppressed the H2O2-induced increase of the BAX/BCL-2 ratio. Down-regulated BAX/BCL-2 protein levels indicate that PO and ALA had an apoptosisinhibitory effect against oxidative stress.
Caspases are comprised of more than 10 homologues; caspase-3 is majorly associated with neuronal apoptosis. Activated caspase-9 and -3 mediate the H2O2-induced neuronal cell death by up-regulation of cleaved-PARP, which is a hallmark of apoptosis [48]. Therefore, inhibition of activated caspase-3 and -9 is attributed as a protective activity against neuronal cell loss. In the present study, PO and ALA treatment significantly suppressed the PARP cleavage compared with those of the vehicle group, suggesting that PO and ALA protected against the H2O2-induced apoptotic process by inhibiting the activation of caspase-9 and -3. Taken together, our results indicate that treatment with PO and ALA has potential anti-apoptotic effects by down-regulation of caspase-9 and -3 activation via modulating the BCL-2 and BAX expressions.
Overall, our results indicate that treatment with PO and ALA increased the cell viability and inhibited LDH activity and nuclei condensation, thus protecting the H2O2-induced neuronal cell death. These neuroprotective capacities of PO and ALA in H2O2-induced SH-SY5Y cells might be related to the regulation of the apoptosis signaling pathway (i.e., down-regulation of cleaved PARP, cleaved caspase-9, and -3). In addition, increased levels of anti-apoptotic protein BCL-2 and down-regulated pro-apoptotic BAX expression indicate that PO and ALA block the H2O2-mediated neuronal apoptotic pathway. ALA in PO may contribute towards the up-regulation of the survival pathway in neuronal cells. There are very few comparison studies that have evaluated the direct influence of ALA, EPA and DHA on human health. Therefore, it remains unclear whether ALA has its own independent effect on anti-apoptosis against oxidative stress in neuronal cells. According to Egert et al. [49], although dietary ALA, EPA and DHA differ considerably in their mode of affect, ALA was as effective in improving lipid metabolism as EPA or DHA. In addition, some reports show that ALA has a significant anti-inflammatory [50] and cardioprotective effect [51], similar to EPA and DHA. We suggest that ALA itself exerts a protective role against oxidative stress in neuronal cells, and not by conversion to EPA or DHA. In our previous study, we confirmed that administration of PO and ALA has a protective effect against Aβ-induced cognitive impairment under behavioral tests in mice model. Also, the effects of PO and ALA on oxidative stress was reflected by inhibition of NO and MDA production in the Aβ-injected mice brain tissue [1112], suggesting that PO has a neuroprotective effect against neurotoxicity induced by oxidative stress. We are currently conducting further reverse transcription-PCR studies to investigate whether PO and ALA regulate the pro- or anti-apoptosis-related mRNA expressions. Taken together, our results indicate that PO and ALA have modulatory effects on oxidative stress-induced neuronal cell death.
Notes
This work was carried out with the support of the Cooperative Research Program for Agriculture Science & Technology Development (PJ01015603), Rural Development Administration, Republic of Korea. This research was also supported by Global PH.D Fellowship Program through the National Research Foundation of Korea (NRF) funded by the Ministry of Education (2015H1A2A1032531).
References
1. Jacobson MD. Reactive oxygen species and programmed cell death. Trends Biochem Sci. 1996; 21:83–86. PMID: 8882579.


2. Halliwell B, Aruoma OI. DNA damage by oxygen-derived species. Its mechanism and measurement in mammalian systems. FEBS Lett. 1991; 281:9–19. PMID: 1849843.


3. Braughler JM, Hall ED. Central nervous system trauma and stroke. I. Biochemical considerations for oxygen radical formation and lipid peroxidation. Free Radic Biol Med. 1989; 6:289–301. PMID: 2663662.
4. Moreira PI, Honda K, Liu Q, Santos MS, Oliveira CR, Aliev G, Nunomura A, Zhu X, Smith MA, Perry G. Oxidative stress: the old enemy in Alzheimer's disease pathophysiology. Curr Alzheimer Res. 2005; 2:403–408. PMID: 16248845.


5. Xue S, Jia L, Jia J. Hypoxia and reoxygenation increased BACE1 mRNA and protein levels in human neuroblastoma SH-SY5Y cells. Neurosci Lett. 2006; 405:231–235. PMID: 16901640.


6. Zheng L, Roberg K, Jerhammar F, Marcusson J, Terman A. Autophagy of amyloid beta-protein in differentiated neuroblastoma cells exposed to oxidative stress. Neurosci Lett. 2006; 394:184–189. PMID: 16297550.


7. Yadav NS, Wierzbicki A, Aegerter M, Caster CS, Pérez-Grau L, Kinney AJ, Hitz WD, Booth JR Jr, Schweiger B, Stecca KL, Allen SM, Blackwell M, Reiter RS, Carlson TJ, Russell SH, Feldmann KA, Pierce J, Browse J. Cloning of higher plant omega-3 fatty acid desaturases. Plant Physiol. 1993; 103:467–476. PMID: 8029334.


8. Oliver MF. It is more important to increase the intake of unsaturated fats than to decrease the intake of saturated fats: evidence from clinical trials relating to ischemic heart disease. Am J Clin Nutr. 1997; 66:980S–986S. PMID: 9322577.


9. Calder PC. n-3 polyunsaturated fatty acids, inflammation, and inflammatory diseases. Am J Clin Nutr. 2006; 83:1505S–1519S. PMID: 16841861.


10. Butterfield DA, Reed T, Newman SF, Sultana R. Roles of amyloid β-peptide-associated oxidative stress and brain protein modifications in the pathogenesis of Alzheimer's disease and mild cognitive impairment. Free Radic Biol Med. 2007; 43:658–677. PMID: 17664130.


11. Lee AY, Choi JM, Lee J, Lee MH, Lee S, Cho EJ. Effects of vegetable oils with different fatty acid compositions on cognition and memory ability in Aβ25–35-induced Alzheimer's disease mouse model. J Med Food. 2016; 19:912–921.
12. Lee AY, Lee MH, Lee S, Cho EJ. Alpha-linolenic acid from Perilla frutescens var. japonica oil protects Aβ-induced cognitive impairment through regulation of APP processing and Aβ degradation. J Agric Food Chem. 2017; 65:10719–10729. PMID: 29092397.
13. Skowyra M, Falguera V, Azman NA, Segovia F, Almajano MP. The effect of perilla frutescens extract on the oxidative stability of model food emulsions. Antioxidants (Basel). 2014; 3:38–54. PMID: 26784662.
14. Hassan A, Ibrahim A, Mbodji K, Coëffier M, Ziegler F, Bounoure F, Chardigny JM, Skiba M, Savoye G, Déchelotte P, Marion-Letellier R. An α-linolenic acid-rich formula reduces oxidative stress and inflammation by regulating NF-κB in rats with TNBS-induced colitis. J Nutr. 2010; 140:1714–1721. PMID: 20724486.


15. Seong J, Song YO. Perilla oil rich in α-linolenic acid inhibits neuronal apoptosis and the expression of inflammation-mediator protein in apoE KO mice. Biocatal Agric Biotechnol. 2012; 1:167–173.


16. Fahn S, Cohen G. The oxidant stress hypothesis in Parkinson's disease: evidence supporting it. Ann Neurol. 1992; 32:804–812. PMID: 1471873.


17. Lee J, Rodriguez JP, Kim YJ, Lee MH, Cho EJ, Lee S. Fatty acid content in perilla cultivars commercial oils determined by GC analysis. Nat Prod Sci. 2016; 22:259–262.
18. Lee J, Lee MH, Cho EJ, Lee S. High-yield methods for purification of α-linolenic acid from Perilla frutescens var. japonica oil. Appl Biol Chem. 2016; 59:89–94.
19. Cory AH, Owen TC, Barltrop JA, Cory JG. Use of an aqueous soluble tetrazolium/formazan assay for cell growth assays in culture. Cancer Commun. 1991; 3:207–212. PMID: 1867954.


20. Racher AJ, Looby D, Griffiths JB. Use of lactate dehydrogenase release to assess changes in culture viability. Cytotechnology. 1990; 3:301–307. PMID: 1366664.


21. Xiao GH, Jeffers M, Bellacosa A, Mitsuuchi Y, Vande Woude GF, Testa JR. Anti-apoptotic signaling by hepatocyte growth factor/met via the phosphatidylinositol 3-kinase/Akt and mitogen-activated protein kinase pathways. Proc Natl Acad Sci U S A. 2001; 98:247–252. PMID: 11134526.


22. Arias E, Gallego-Sandín S, Villarroya M, García AG, López MG. Unequal neuroprotection afforded by the acetylcholinesterase inhibitors galantamine, donepezil, and rivastigmine in SH-SY5Y neuroblastoma cells: role of nicotinic receptors. J Pharmacol Exp Ther. 2005; 315:1346–1353. PMID: 16144975.


23. Lopes JP, Oliveira CR, Agostinho P. Role of cyclin-dependent kinase 5 in the neurodegenerative process triggered by amyloid-beta and prion peptides: implications for Alzheimer's disease and prion-related encephalopathies. Cell Mol Neurobiol. 2007; 27:943–957. PMID: 17965932.


24. Shibata N, Kobayashi M. The role for oxidative stress in neurodegenerative diseases. Brain Nerve. 2008; 60:157–170. PMID: 18306664.
25. Aruoma OI, Bahorun T, Jen LS. Neuroprotection by bioactive components in medicinal and food plant extracts. Mutat Res. 2003; 544:203–215. PMID: 14644322.


26. Kitagishi Y, Nakanishi A, Ogura Y, Matsuda S. Dietary regulation of PI3K/AKT/GSK-3β pathway in Alzheimer's disease. Alzheimers Res Ther. 2014; 6:35. PMID: 25031641.


27. Zheng WX, Wang F, Cao XL, Pan HY, Liu XY, Hu XM, Sun YY. Baicalin protects PC-12 cells from oxidative stress induced by hydrogen peroxide via anti-apoptotic effects. Brain Inj. 2014; 28:227–234. PMID: 24456060.


28. Palacios-pelaez R, Lukiw WJ, Bazan NG. Omega-3 essential fatty acids modulate initiation and progression of neurodegenerative disease. Mol Neurobiol. 2010; 41:367–374. PMID: 20467837.


29. Chung KH, Hwang HJ, Shin KO, Jeon WM, Choi KS. Effects of perilla oil on plasma concentrations of cardioprotective (n-3) fatty acids and lipid profiles in mice. Nutr Res Pract. 2013; 7:256–261. PMID: 23964311.


30. Gerster H. Can adults adequately convert alpha-linolenic acid (18:3n-3) to eicosapentaenoic acid (20:5n-3) and docosahexaenoic acid (22:6n-3)? Int J Vitam Nutr Res. 1998; 68:159–173. PMID: 9637947.
31. Pawlosky RJ, Hibbeln JR, Novotny JA, Salem N. Physiological compartmental analysis of α-linolenic acid metabolism in adult humans. J Lipid Res. 2001; 42:1257–1265. PMID: 11483627.


32. Blondeau N, Tauskela JS. A new future in brain preconditioning based on nutraceuticals: a focus on α-linolenic omega-3 fatty acid for stroke protection. In : Gidday JM, Perez-Pinzon MA, Zhang JH, editors. Innate Tolerance in the CNS. New York (NY): Springer;2013. p. 133–163.
33. Jang JY, Kim TS, Kim J, Kim Y, Shin K, Kim K, Lee SP, Kang MH, Choi EK, Ree MH, Kim YB. Perilla oil improves blood flow through inhibition of platelet aggregation and thrombus formation. Lab Anim Res. 2014; 30:21–27. PMID: 24707301.


34. Senavong P, Kongkham S, Saelim S, Suangkavathin V. Neuroprotective effect of perilla extracts on PC12 cells. J Med Assoc Thai. 2016; 99:S256–S264.


35. Lee AY, Lee MH, Lee S, Cho EJ. Comparative study on antioxidant activity of vegetable oils under in vitro and cellular system. J Agric Sci. 2015; 7:58–65.


36. Kim SS, Park RY, Jeon HJ, Kwon YS, Chun W. Neuroprotective effects of 3,5-dicaffeoylquinic acid on hydrogen peroxide-induced cell death in SH-SY5Y cells. Phytother Res. 2005; 19:243–245. PMID: 15934031.


37. Chetsawang J, Govitrapong P, Chetsawang B. Hydrogen peroxide toxicity induces Ras signaling in human neuroblastoma SH-SY5Y cultured cells. J Biomed Biotechnol. 2010; 2010:803815. PMID: 20871828.


38. Barbosa DS, Cecchini RE, Kadri MZ, Rodriguez MA, Burini RC, Dichi I. Decreased oxidative stress in patients with ulcerative colitis supplemented with fish oil ω-3 fatty acids. Nutrition. 2003; 19:837–842. PMID: 14559317.


39. Fotakis G, Timbrell JA. In vitro cytotoxicity assays: Comparison of LDH, neutral red, MTT and protein assay in hepatoma cell lines following exposure to cadmium chloride. Toxicol Lett. 2006; 160:171–177. PMID: 16111842.
40. Wang P, Henning SM, Heber D. Limitation of MTT and MTS-based assays for measurement of antiproliferative activity of green tea polyphenols. PLoS One. 2010; 5:e10202. PMID: 20419137.
41. Enari M, Sakahira H, Yokoyama H, Okawa K, Iwamatsu A, Nagata S. A caspase-activated DNase that degrades DNA during apoptosis, and its inhibitor ICAD. Nature. 1998; 391:43–50. PMID: 9422506.


42. Kwon SH, Kim MJ, Ma SX, You IJ, Hwang JY, Oh JH, Kim SY, Kim HC, Lee SY, Jang CG. Eucommia ulmoides Oliv. Bark. protects against hydrogen peroxide-induced neuronal cell death in SH-SY5Y cells. J Ethnopharmacol. 2012; 142:337–345. PMID: 22735663.
43. Hu XL, Niu YX, Zhang Q, Tian X, Gao LY, Guo LP, Meng WH, Zhao QC. Neuroprotective effects of Kukoamine B against hydrogen peroxide-induced apoptosis and potential mechanisms in SH-SY5Y cells. Environ Toxicol Pharmacol. 2015; 40:230–240. PMID: 26164594.


44. Youle RJ, Strasser A. The Bcl-2 protein family: opposing activities that mediate cell death. Nat Rev Mol Cell Biol. 2008; 9:47–59. PMID: 18097445.


45. Reed JC. Double identity for proteins of the Bcl-2 family. Nature. 1997; 387:773–776. PMID: 9194558.


46. Mattson MP. Apoptosis in neurodegenerative disorders. Nat Rev Mol Cell Biol. 2000; 1:120–130. PMID: 11253364.


47. Murphy KM, Ranganathan V, Farnsworth ML, Kavallaris M, Lock RB. Bcl-2 inhibits Bax translocation from cytosol to mitochondria during drug-induced apoptosis of human tumor cells. Cell Death Differ. 2000; 7:102–111. PMID: 10713725.


48. Grütter MG. Caspases: key players in programmed cell death. Curr Opin Struct Biol. 2000; 10:649–655. PMID: 11114501.


49. Egert S, Kannenberg F, Somoza V, Erbersdobler HF, Wahrburg U. Dietary alpha-linolenic acid, EPA, and DHA have differential effects on LDL fatty acid composition but similar effects on serum lipid profiles in normolipidemic humans. J Nutr. 2009; 139:861–868. PMID: 19261730.
50. Zhao G, Etherton TD, Martin KR, Vanden Heuvel JP, Gillies PJ, West SG, Kris-Etherton PM. Anti-inflammatory effects of polyunsaturated fatty acids in THP-1 cells. Biochem Biophys Res Commun. 2005; 336:909–917. PMID: 16169525.


51. Albert CM, Oh K, Whang W, Manson JE, Chae CU, Stampfer MJ, Willett WC, Hu FB. Dietary α-linolenic acid intake and risk of sudden cardiac death and coronary heart disease. Circulation. 2005; 112:3232–3238. PMID: 16301356.


Fig. 1
Effects of PO (A) and ALA (B) on cell viability in hydrogen peroxide-induced SH-SY5Y cells.
PO, Perilla oil; ALA, Alpha-linolenic acid. Values are mean ± SD. a–dMeans with different letters are significantly different (P < 0.05) as determined by Duncan's multiple range test. The results were obtained in three independent experiments.
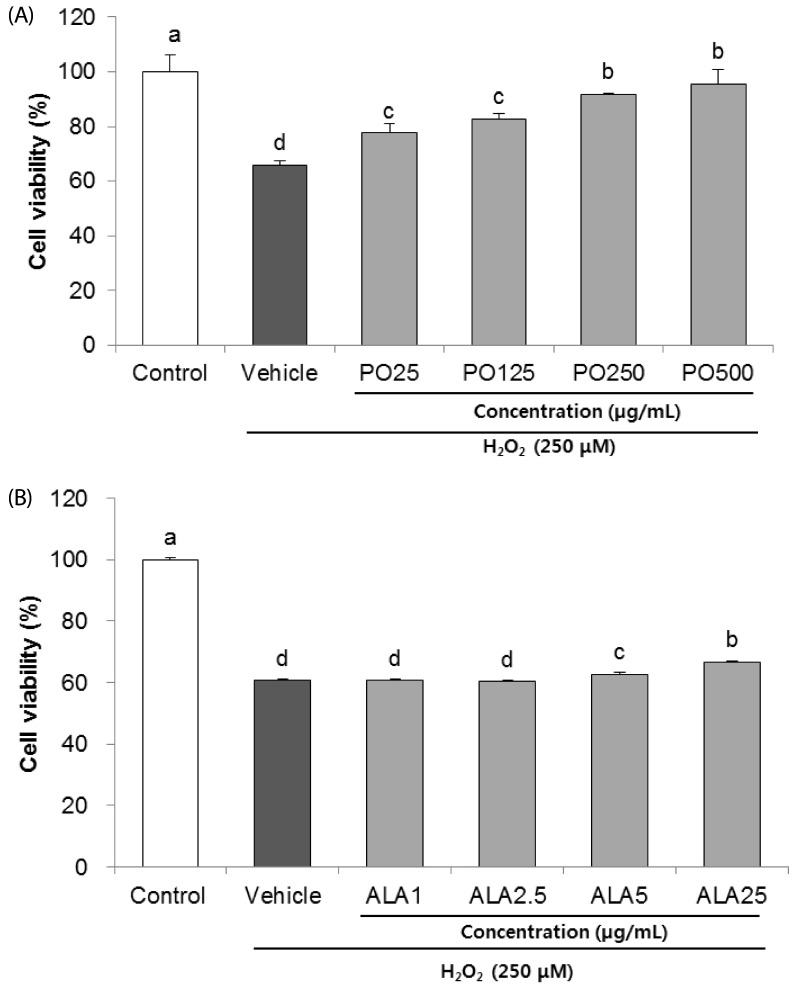
Fig. 2
Protective effect of PO (A) and ALA (B) on lactate dehydrogenase (LDH) release in SH-SY5Y cells treated with hydrogen peroxide.
PO, Perilla oil; ALA, Alpha-linolenic acid. Values are mean ± SD. a–dMeans with different letters are significantly different (P < 0.05) as determined by Duncan's multiple range test. The results were obtained in three independent experiments.
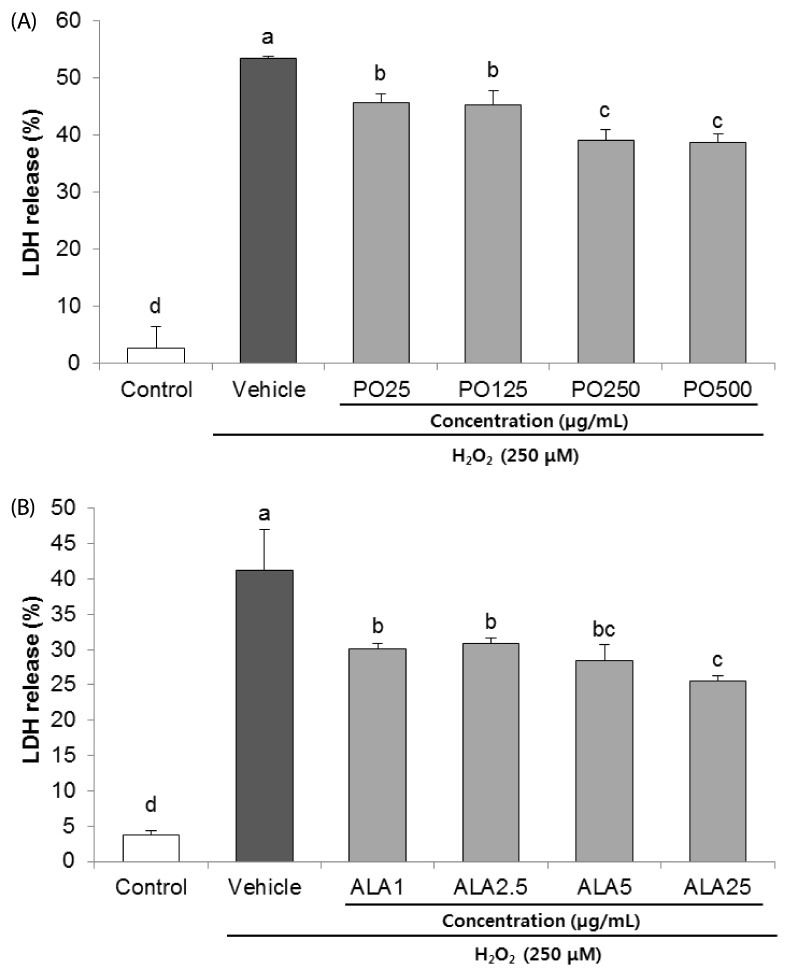
Fig. 3
Effects of PO and ALA on DNA condensation in SH-SY5Y cells treated with hydrogen peroxide.
The nuclear morphology of cells was determined by Hoechst 33342 staining. (A) Representative images of nuclei stained with Hoechst 33342. The apoptotic bodies are indicated with arrows. (B) Quantification of the nuclei demonstrating apoptotic bodies observed under a fluorescence microscope. Magnification was 400x. PO, Perilla oil; ALA, Alpha-linolenic acid. Values are mean ± SD. a–eMeans with different letters are significantly different (P < 0.05) as determined by Duncan's multiple range test. The results were obtained in three independent experiments.

Fig. 4
Effects of PO and ALA on levels of cleaved caspase-9 protein expression in SH-SY5Y cells treated with hydrogen peroxide.
PO, Perilla oil; ALA, Alpha-linolenic acid. Values are mean ± SD. a–cMeans with different letters are significantly different (P < 0.05) as determined by Duncan's multiple range test. β-actin was used as a loading control. The results were obtained in three independent experiments.
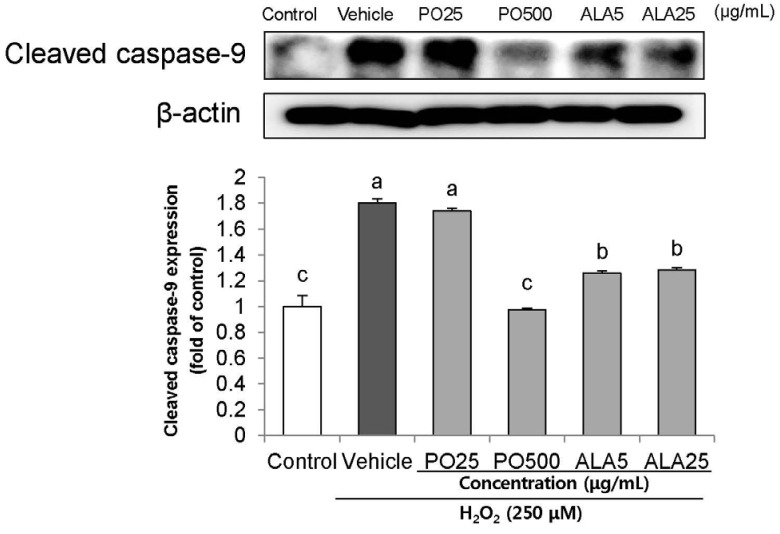
Fig. 5
Effects of PO and ALA on levels of cleaved caspase-3 protein expression in SH-SY5Y cells treated with hydrogen peroxide. PO, Perilla oil; ALA, Alpha-linolenic acid.
Values are mean ± SD. a–eMeans with different letters are significantly different (P < 0.05) as determined by Duncan's multiple range test. β-actin was used as a loading control. The results were obtained in three independent experiments.

Fig. 6
Effect of PO and ALA on levels of cleaved-poly ADP ribose polymerase protein expression in SH-SY5Y cells treated with hydrogen peroxide.
PO, Perilla oil; ALA, Alpha-linolenic acid. Values are mean ± SD. a–fMeans with different letters are significantly different (P < 0.05) as determined by Duncan's multiple range test. β-actin was used as a loading control. The results were obtained in three independent experiments.

Fig. 7
Effect of PO and ALA on levels of BAX/BCL-2 ratio protein expression in SH-SY5Y cells treated with hydrogen peroxide.
PO, Perilla oil; ALA, Alpha-linolenic acid. Values are mean ± SD. a–eMeans with different letters are significantly different (P < 0.05) as determined by Duncan's multiple range test. β-actin was used as a loading control. The results were obtained in three independent experiments.
