Abstract
BACKGROUND/OBJECTIVES
Although the antioxidative effects of lycopene are generally known, the molecular mechanisms underlying the anti-inflammatory properties of lycopene are not fully elucidated. This study aimed to examine the role and mechanism of lycopene as an inhibitor of inflammation.
METHODS/MATERIALS
Lipopolysaccharide (LPS)-stimulated SW 480 human colorectal cancer cells were treated with 0, 10, 20, and 30 µM lycopene. The MTT assay was performed to determine the effects of lycopene on cell proliferation. Western blotting was performed to observe the expression of inflammation-related proteins, including nuclear factor-kappa B (NF-κB), inhibitor kappa B (IκB), mitogen-activated protein kinase (MAPK), extracellular signal-related kinase (ERK), c-jun NH2-terminal kinase (JNK), and p38 (p38 MAP kinase). Real-time polymerase chain reaction was performed to investigate the mRNA expression of tumor necrosis factor α (TNF-α), interleukin-1 beta (IL-1β), interleukin-6 (IL-6), inducible nitric oxide synthase (iNOS), and cyclooxygenase-2 (COX-2). Concentrations of nitric oxide (NO) and prostaglandin E2 (PGE2) were determined via enzyme-linked immunosorbent assays.
RESULTS
In cells treated with lycopene and LPS, the mRNA expression of TNF-α, IL-1β, IL-6, iNOS, and COX-2 were decreased significantly in a dose-dependent manner (P < 0.05). The concentrations of PGE2 and NO decreased according to the lycopene concentration (P < 0.05). The protein expressions of NF-κB and JNK were decreased significantly according to lycopene concertation (P < 0.05).
In Korea, cancer has emerged as one of the most problematic diseases. Although the rates of lung cancer, liver cancer, and gastric cancer have decreased continuously since 2000, the death rate associated with colorectal cancer (CRC) has increased with age from 1999 to 2012 at an average annual increase rate of 5.2% [12]. In 2013, CRC was the third-most common type of cancer among South Korean cancer patients, with an incidence of 12.3% (behind thyroid and stomach cancer) and was the second-most common type among men (14.6%) and third-most common among women (9.9%) [3].
Inflammation is the local reaction of an area of the body to tissue damage and is characterized by symptoms such as swelling, fever, and pain. During acute inflammation, immune cells eliminate viruses or other pathogens through various processes. In the context of a sustained or repeated inflammatory response, various cytokines are secreted to damage the functions of macrophages [4]. It is generally accepted that chronic sustained inflammation, caused by high fat diet, obesity, or low vegetable consumption, are related with the CRC development [456]. Furthermore, CRC patients who exhibit inflammatory responses tend to have a higher tumor recurrence rate and increased number of side effects to medical and surgical treatment than those without inflammatory responses [7].
The inflammation cascade signaling molecules are the source of clinical targets that when antagonized, block the physiological effect of the cascade or pathway. Some of inflammatory cytokines, including tumor necrosis factor α (TNF-α), interleukin-1 beta (IL-1β), and interleukin-6 (IL-6), as well as inflammation-inducing enzymes, such as cyclooxygenase-2 (COX-2) and inducible nitric oxide synthase (iNOS), were revealed to implicate in CRC [8]. Therefore inhibitors of these inflammatory pathways could be ideal against CRC.
Lycopene is a main component of red-colored fruits and vegetables, composing of 40 carbon atoms linked through unsaturated and conjugated double bonds [9]. Lycopene has been reported to exert anti-inflammatory and anticancer effects and lycopene intake has been found to relate with decreases in the incidence of breast cancer, prostate cancer, and lung cancer [9101112]. Giovannucci et al. [13] reported that the consumption of tomatoes or lycopene correlated negatively with the incidence rate of CRC. However, no previous study has investigated the anti-inflammatory effects of lycopene in SW 480 cell (CRC cell). Therefore here we evaluated whether lycopene proliferates SW480 human CRC cell and targets nuclear factor-kappa B (NF-κB) activation and associated signaling as a mechanism of its anti-inflammatory effects in CRC.
Lycopene was purchased from Sigma (St. Louis, MO, USA) and was dissolved in dimethyl sulfoxide (DSMO). Cell culture media was purchased from Welgene (Gyeongsangbuk-do, South Korea). Western blotting antibodies against β-actin, extracellular signal-related kinase (ERK), c-jun NH2-terminal kinase (JNK), p38 (p38 MAP kinase), NF-κB and inhibitor kappa B (IκB) were purchased from Cell Signaling Technologies (Danvers, MA, USA).
SW480 human colorectal cancer cells were purchased from a Korean cell line bank. Cells were maintained in Dulbecco's modified Eagle's medium/Nutrient Mixture Ham's F12 (DMEM/F12; Welgene, Korea) containing 10% fetal bovine serum (FBS; Welgene, Korea), 100 units/mL penicillin, and 100 µg/mL streptomycin in a 37℃, 5% CO2 incubator. The medium was replaced every 2-3 days for subculture.
Cells were plated in 24-well plates at a density of 2.5 × 104 cells/mL. After 48 h, the medium was replaced with serum-free media (SFM) to induce serum starvation for the next 24 h. After an overnight incubation, the cells were treated with various concentrations of lycopene (0–30 µM) in the presence or absence of Lipopolysaccharide (LPS) to induce inflammation (100 ng/mL), according to the experimental design. An MTT assay was performed, as previously described [14], every 0, 24, 48, and 96 h during experimental treatments, after which the optical density of the plates was measured at 490 nm on a microplate reader (Tecan, Groedig, Austria).
Cells treated with lycopene in the presence or absence of LPS were collected and washed with ice-cold phosphate-buffered saline (PBS), and the resulting cell pellets were suspended in 400 µL of RIPA lysis buffer (Cell Signaling Technology). Subsequently, the lysates were then mixed in an oscillator for 30 min and centrifuged (12,000 g at 4℃) for 5 min to obtain the cytosolic fraction. Protein concentrations were determined using the Bradford assay (Bio-Rad, Munich, Germany) according to the manufacturer's instructions.
Samples of cellular protein from cell extracts were separated using 8–10% sodium dodecyl sulfate polyacrylamide gel electrophoresis (SDS-PAGE) and transferred to membranes. Antibodies against β-actin, ERK, JNK, p38, NF-κB, and IκB were diluted in 5% milk or BSA/TBST (20 mM Tris-HCl, 0.1% Tween 20, 137 mM NaCl, pH 7.4) and applied to membranes, followed by an overnight incubation at 4℃. After three 10–20 min washes in TBST, the membranes were incubated for 2–3 h in anti-mouse or rabbit IgG (Cell Signaling). After another series of washes in TBST, enhanced chemiluminescent (ECL) detection solution (Bio-Rad) was added to the blots for 0.5-2 min. The blots were then exposed to X-Omat Film (Kodak), and the molecular weights of the detected bands were compared against a high molecular weight marker. Each band concentration was calculated using Image J Launcher (National Institutes of Health, Bethesda, MD, USA), imaging programs [15].
Total RNA was extracted using TRIzol reagent (Sigma). A 5 µg aliquot of extracted RNA was subjected to reverse transcription to generate cDNA. A 2 µL sample of cDNA was then mixed with 6 µL of nuclease-free water, 10 µL of 2X SYBR Green Master Mix (Applied Biosystems, Foster City, CA, USA), and 1 µL each of primer (forward/reverse) and subjected to PCR. The primers used for each experiment are shown in Table 1. Applied Biosystems Step One software v2.1 was used to set the following conditions: 10 min at 95℃, followed by 40 cycles of 15 min in 95℃, 1 min at 60℃, 15 min at 95℃, 1 min at 60°, and 15 min in 95℃. Data were analyzed using a program included in the Applied Biosystems StepOne software v2.1 package, using the ΔΔCT method. Each experiment was repeated three times [1617].
After treating SW480 cells with media containing 100 ng/mL LPS and 0, 10, 20, or 30 µM lycopene, cell supernatants were collected and prostaglandin E2 (PGE2) concentrations were determined using an enzyme-linked immunosorbent assay (ELISA) kit (ADI-900-001; Enzo Life Sciences, Farmingdale, NY, USA) [18]. To determine the concentrations of nitric oxide (NO), the cell supernatant was mixed with Griess reagent at a ratio of 1:1 in a 96-well plate and allowed to react for 10 min. Subsequently, the absorbance in each well at 540 nm was determined using a microplate reader (Infinite 200; TECAN, Grödig, Austria) [19].
At least three independent repeats of all experiments in this study were performed, and the obtained results were analyzed using SPSS (version 23; SPSS, Inc., Chicago, IL, USA) to calculate the mean and standard deviation of each groups. After performing the analysis of variance (ANOVA), the differences among the groups were calculated using Duncan's multiple range test; significance was defined as an α level < 0.05.
When cells treated with lycopene (0–30 µM) for 0, 24, 48, and 96 h were subjected to the MTT assay, lycopene was found to inhibit proliferation in a concentration-dependent manner at 96 h (P < 0.05; Fig. 1). Subsequently, cells were treated with lycopene for 24 h and additionally with LPS (100 ng/mL) for another 18 h to induce inflammation. Treatment with both LPS and lycopene did not affect cell proliferation; therefore, this condition was used for the remainder of the experiments.
When MTT assay was performed after SW 480 cell were treated with 0, 10, 20, and 30 µM of lycopene and incubated for 0, 24, 48, and 96 h, it was found that lycopene did not affect cell proliferation as a function of time after exposure at 0, 24, and 48 h. After 96 h, however, the lycopene suppressed SW 480 cell proliferation accordingly with its concentration (P < 0.05) (Fig. 1). This study tried to see the effects of lycopene in suppressing inflammation under the condition that the lycopene treatment did not affect cell proliferation, and so the time for incubation was set at 24 h. Another reason we decided to incubate SW 480 cells only for 24 h was that 24 h incubation of SW 480 cell has no effect on protein and mRNA expression due to apoptosis.
The expression levels of mRNAs encoding the inflammatory cytokines TNF-α, IL-1β, and IL-6 in SW480 cells were measured. The expression of cytokine mRNAs was found to increase significantly with LPS treatment and to decrease in response to lycopene treatment in a concentration-dependent manner (P < 0.05; Fig. 2).
LPS treatment led to a significant increase in the expression of COX-2 mRNA, whereas treatment with lycopene significantly decreased the expression of COX-2 mRNA in a lycopene dose-dependent manner (P < 0.05; Fig. 3). PGE2 production was also found to decrease significantly in response to lycopene treatment in a dose-dependent manner (P < 0.05).
The expression of iNOS mRNA and production of NO were increased significantly with LPS treatment but decreased significantly in a dose-dependent manner with lycopene treatment (P < 0.05; Fig. 4).
The expression levels of NF-κB and mitogen-activated protein kinase (MAPK) (ERK, JNK, p38) proteins, which are related to inflammation, were measured in SW480 cells. The expression of NF-κB and IκB were increased significantly with LPS treatment, and these levels differed significantly according to the lycopene concentration (P < 0.05; Fig. 5). Regarding MAPKs, the expression levels of all three proteins were increased significantly with LPS treatment. The expression of JNK protein were decreased significantly according to the lycopene concentration (P < 0.05). The expression of ERK were decreased significantly in response to treatment only with 30 µM lycopene (P < 0.05). The expression of p38 tended to decrease in a lycopene concentration-dependent manner, but this trend was not significant (Fig. 6).
Colorectal cancer (CRC) represents a paradigm for the connection between inflammation and cancer, based on studies which indicate a causal association of CRC with genes encoding for pro-inflammatory mediators [20]. Lycopene has been reported to exert anti-inflammatory and anticancer effects and is known to prevent chronic vascular diseases and cancer [9101112]. However, the anti-inflammatory effects of lycopene in CRC cell and the related mechanisms have not been sufficiently studied.
To see the cytotoxic effect of lycopene on SW 480 cell (human CRC cell), SW 480 cells were treated with various concentrations of lycopene (0–100 µM), concentrations used in previous lycopene and CRC cell study [21222324]. As a result of MTT assay, 100 µM concentration of lycopene showed minimal membrane damage on SW 480 cells after 24 h of exposure compared to the culture control. At 30 µM, lycopene showed no cell damage (99% cell viability), indicating that the any inhibition effect of lycopene up to the concentration of 30 µM lycopene on SW 480 cell was not due to cell death. Thus in this study, SW 480 cells were treated with 0, 10, 20, and 30 µM of lycopene, which elicited an effective anti-inflammatory activity.
In this study, the inhibitory effect of various lycopene treatment was detected at 24, 48 and 96 h of incubation. Lycopene treatment to SW 480 cells led to a significant decrease in the proliferation of SW480 cells at 96 h after treatment. Several in vitro studies have also been reported that lycopene inhibited cell growth in CRC cells. Lee et al. [21] found that 0–100 µM of lycopene has a suppressive effect, as a dose dependent matter, on the proliferation of colorectal cancer cells through apotosis. Huang et al. [22] reported that with lycopene treatment (12 µM), the proportion of CRC cells undergoing late apoptosis or necrosis was higher than early apoptosis. Other studies also reported the antiproliferation effect of lycopene treatment (2–20 µM) on HT-29 cells, another CRC cells [2324]. The difference in antiproliferation effect may be accounted for by the source of lycopene used in cell culture and the amount of lycopene used in each study. In order to identify the anti-inflammatory effects of lycopene in SW 480 cell without affecting cell proliferation, the time of 24 h of lycopene treatment was used to perform dose-response experiments.
LPS is a potent inducer of inflammation, triggering an early inflammatory response that constitutes the first mechanism of defense by the host. The host response to LPS is characterized by the production of various cytokines and proinflammatory mediators [25]. The effective dosages of LPS to induce inflammation were reported in a wide range, from 10 ng to 1 mg/mL [26272829]. In order to see the anti-inflammatory effect of lycopene on colon cancer cell, SW 480 cells were treated with lycopene for 24 h and then cells were again treated with LPS for 18 h to induce inflammation. As a result of MTT assay, it was found the both LPS (100 ng/mL) and lycopene treatment (0–30 µM) did not affect cell proliferation, and so this condition was used for the remainder of the experiment. In response to LPS treatment to SW 480 cells, the mRNA expression levels of TNF-α, IL-1β, and IL-6 were increased significantly but decreased significantly in response to lycopene in a dose-dependent manner. Lycopene was also significantly effective in inhibiting mRNA expressions of COX-2 and iNOS with subsequent inhibition of PGE2 and NO production, which confirming the anti-inflammatory effects of this molecule in colon cancer cells.
COX-2, the expression of which is induced by inflammatory stimuli, biosynthesizes PGE2 from arachidonic acid, thus inducing inflammation and atropy. In response to an inflammatory stimulus, iNOS produces NO, a harmful compound in the body that increases vascular permeability, causes edema and septic shock consequent to excessive vasodilatation, inhibits wound healing, and intensifies inflammation in damaged nerve tissues, cytotoxicity, and other types of tissue damage [30].
Although no previous study of anti-inflammatory effects of lycopene on CRC cell exist, lycopene reduced several proinflammatory mediators, such as IL-1β, TNF-a, or proinflammatory enzyme activities, such as COX-2 in a variety of cell lines, including blood mononuclear cells (PBMC) from human subjects [27], human macrophages [28], and mouse macrophage [29]. Several In vivo and human studies have also suggested promising anti-inflammatory effects of lycopene in the development of colon cancer [132031323334]. Lycopene was found to have anti-inflammatory effects in a rat model of induced-colitis [31]. In that study, a more attenuated inflammatory reaction in terms of microscopic changes and decreased myeloperoxidase (MPO) activity, as a marker of inflammation, were observed in the group fed a diet supplemented with lycopene (300 µg/rat/day) while no significant effect was noted in the beta-carotene-supplemented group. In human studies, lycopene consumption interacted with MAPK-signaling genes to alter risk of colorectal cancer [32]. Giovannucci et al. [13] reported that the intake level of tomato or lycopene was negatively correlated with the incidence of colorectal cancer. Those above findings support our in vitro study reporting that lycopene treatment may help relieve inflammation in CRC. This study showed that LPS treatment induces the activation of NF-κB, as confirmed by IκB phosphorylation and lycopene inhibited expression of NF-κB as a dose dependent manner. Inactivation of NF-κB by lycopene was strongly linked the decreased expression of the proinflammatory cytokines, such as TNF-α, IL-1β, and IL-6, and decreased expression of inflammatory releasing enzymes, COX-2 and iNOS with subsequent inhibition of PGE2 and NO production.
Several studies have also been reported that lycopene modulates NF-κB activation, showing anti-inflammatory properties related to the inhibition of the NF-κB signaling cascade [26272829]. Lycopene has been suggested to induce NF-κB inactivation by inhibiting the phosphorylation of i kappa B kinase alpha (IKKα) and i kappa B alpha (IKBα) and by decreasing the translocation of the NF-κBp65 subunit from the cytosol to the nucleus [28]. NF-κB is activated constitutively in many tumors/tumor cells, including human CRC cells [3819]. This is one of the major reasons why the chemotherapeutic agents are ineffective in inducing apoptosis in cancer cells including CRC [815]. Constitutively active NF-κB provides growth and survival signals in human malignancies, suggesting that the agents that inhibit NF-κB activation could be effective in CRC prevention and therapy [811].
We investigated whether the inhibitory effect of lycopene on LPS-induced NF-κB activation pathway extends to MAPK signaling pathway. Among MAP kinases, only the expression of JNK protein decreased significantly according to the lycopene concentration. The three major groups of distinctly regulated MAPK cascades are characterized by ERK1/2, JNK, and p38 MAP kinase [35]. The MAP kinases (ERK1/2, p38 and JNK) has been reported to activate NF-κB signaling and the consequent expression of pro-inflammatory cytokines [3637]. JNK pathway, also known as stress-activated protein kinase 1 (SAPK1), is activated by pro-inflammatory cytokines and oxidative stress. JNK have been shown to be modulated by obesity and insulin resistance. A study by Hardwick and colleagues observed that both p38 and JNK were highly expressed in colonic adenomatous polyps [38]. However, no in vitro study regarding lycopene and MAPK signaling pathway in CRC cell exist. Only one gene study showed that dietary factors involved in inflammation and oxidative stress interact with MAPK-signaling genes to alter risk of CRC and MAPK-signaling pathway interacted with lycopene consumption [32].
In conclusion, This study found that lycopene inhibits the protein expression of NF-κB and JNK in SW480 colorectal cancer cells, thus leading to (1) reduced production of TNF-α, IL-1, and IL-6 and (2) inhibited expression of COX-2 and iNOS mRNA with subsequent inhibition of PGE2 and NO production. These findings represent a direct evidence that lycopene may act as an anti-inflammatory compound in cells exposed to LPS and provide novel data on important molecular mechanisms by which lycopene regulates LPS-driven NF-κB-dependent inflammation in human CRC cells. Further in vivo and in vitro study regarding the role of lycopene in modulation of the immune system and dietary supplementation of lycopene for reducing inflammation in CRC are needed to verify our in vitro findings.
Figures and Tables
Fig. 1
Effect of lycopene on cell proliferation in SW480 cells.
The effect of lycopene on viable cell numbers were estimated by the MTT assay (A). The effect of lycopene and LPS on viable cell numbers after 24 h incubation the MTT assay (B). Each graph represents the mean ± SE of three independent experiments. Comparison among concentration of lycopene that yielded significant differences at α = 0.05 as indicated by different letters above each graph by Duncan's multiple range test.
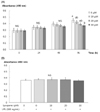
Fig. 2
Effects of lycopene on the mRNA expression of TNF-α, IL-1β and IL-6 in LPS-stimulated SW480 cells.
Total RNA was isolated and real-time PCR was performed. Each graph represents the mean ± SE of three independent experiments. Comparison among concentration of lycopene that yielded significant differences at α = 0.05 as indicated by different letters above each graph by Duncan's multiple range test.
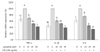
Fig. 3
Effects of lycopene on the mRNA expression of COX-2 (A) and PGE2 concentration (B) in LPS-stimulated SW480 cells.
Total RNA was isolated and real-time PCR was performed. PGE2 concentration was measured at 405 nm by ELISA assay. Each graph represents the mean ± SE of three independent experiments. Comparison among concentration of lycopene that yielded significant differences at α = 0.05 as indicated by different letters above each graph by Duncan's multiple range test.
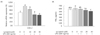
Fig. 4
Effects of lycopene on the mRNA expression of iNOS (A) and NO (B) concentration in LPS-stimulated SW480 cells.
Total RNA was isolated and real-time PCR was performed. NO concentration was measured at 540 nm by Griess reaction assay. Each graph represents the mean ± SE of three independent experiments. Comparison among concentration of lycopene that yielded significant differences at α = 0.05 as indicated by different letters above each graph by Duncan's multiple range test.
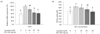
Fig. 5
Effects of lycopene on protein expression of NF-κB (A) and IκB (B) in LPS-stimulated SW480 cells.
Protein expression was measured by western blot assay. Actin was used as an internal control in a densitometric analysis. Each graph represents the mean ± SE of three independent experiments. Comparison among concentration of lycopene that yielded significant differences at α = 0.05 as indicated by different letters above each graph by Duncan's multiple range test.
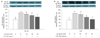
Fig. 6
Effects of lycopene on protein expression of JNK (A), ERK (B), p38 (C) in LPS-stimulated SW480 cells.
Protein expression was measured by western blot assay. Actin was used as an internal control in a densitometric analysis. Each graph represents the mean ± SE of three independent experiments. Comparison among concentration of lycopene that yielded significant differences at α = 0.05 as indicated by different letters above each graph by Duncan's multiple range test.
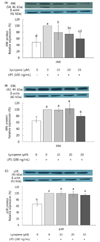
Notes
References
1. Korean Statistical Information Service. Cause of death [Internet]. Daejeon: Statistics Korea;c2014. cited 2016 August 29. Available from: http://kosis.kr/statHtml/statHtml.do?orgId=101&tblId=DT_1B34E01&conn_path=I2.
2. Korean Statistical Information Service. Cancer registration statistics [Internet]. Daejeon: Statistics Korea;c2013. cited 2016 August 29. Available from: http://kosis.kr/statHtml/statHtml.do?orgId=117&tblId=DT_117N_A00022&conn_path=I2.
5. Tuan J, Chen YX. Dietary and lifestyle factors associated with colorectal cancer risk and interactions with microbiota: fiber, red or processed meat and alcoholic drinks. Gastrointest Tumors. 2016; 3:17–24.


6. Cho YA, Lee J, Oh JH, Shin A, Kim J. Dietary inflammatory index and risk of colorectal cancer: a case-control study in Korea. Nutrients. 2016; 8:E469.


7. Youn SW. Systemic inflammatory response as a prognostic factor in patients with cancer. J Korean Orient Oncol. 2012; 17:1–7.
8. Danese S, Mantovani A. Inflammatory bowel disease and intestinal cancer: a paradigm of the Yin-Yang interplay between inflammation and cancer. Oncogene. 2010; 29:3313–3323.


9. Holzapfel NP, Holzapfel BM, Champ S, Feldthusen J, Clements J, Hutmacher DW. The potential role of lycopene for the prevention and therapy of prostate cancer: from molecular mechanisms to clinical evidence. Int J Mol Sci. 2013; 14:14620–14646.


10. Koh MS, Hwang JS, Moon AR. Lycopene inhibits proliferation, invasion and migration of human breast cancer cells. Biomol Ther. 2010; 18:92–98.


11. Giovannucci E. Tomatoes, tomato-based products, lycopene, and cancer: review of the epidemiologic literature. J Natl Cancer Inst. 1999; 91:317–331.


12. Michaud DS, Feskanich D, Rimm EB, Colditz GA, Speizer FE, Willett WC, Giovannucci E. Intake of specific carotenoids and risk of lung cancer in 2 prospective US cohorts. Am J Clin Nutr. 2000; 72:990–997.


13. Giovannucci E, Ascherio A, Rimm EB, Stampfer MJ, Colditz GA, Willett WC. Intake of carotenoids and retinol in relation to risk of prostate cancer. J Natl Cancer Inst. 1995; 87:1767–1776.


14. Seo EY, Lee HS, Kim WK. Effect of [6]-gingerol on inhibition of cell proliferation in MDA-MB-231 human breast cancer cells. Korean J Nutr. 2005; 38:656–662.
15. Lin D, Ma J, Xue Y, Wang Z. Penehyclidine hydrochloride preconditioning provides cardioprotection in a rat model of myocardial ischemia/reperfusion injury. PLoS One. 2015; 10:e0138051.


16. Livak KJ, Schmittgen TD. Analysis of relative gene expression data using real-time quantitative PCR and the 2(-Delta Delta C(T)) method. Methods. 2001; 25:402–408.


17. Son CH, Lee SH, Park CH, Park JT, Park SG, Lee JA, Suh GH, Oh KS. Confirmation of male specific fetal free RNA in maternal plasma and comparison of accuracy on the sex determination using real-time pcr method in Korean native cattle. Korean J Embryo Transf. 2013; 28:343–348.


18. McCarthy MK, Procario MC, Wilke CA, Moore BB, Weinberg JB. Prostaglandin E2 production and T cell function in mouse adenovirus type 1 infection following allogeneic bone marrow transplantation. PLoS One. 2015; 10:e0139235.


19. Kang HJ, Hong SH, Kang KH, Park C, Choi YH. Anti-inflammatory effects of Hwang-Heuk-San, a traditional Korean herbal formulation, on lipopolysaccharide-stimulated murine macrophages. BMC Complement Altern Med. 2015; 15:447.


20. Slattery ML, Lundgreen A, Wolff RK. Dietary influence on MAPK-signaling pathways and risk of colon and rectal cancer. Nutr Cancer. 2013; 65:729–738.


21. Lee HS, Jung JI, Kang YH, Khachik F, Yoon JH. Effect of lycopene on the insulin-like growth factor-I receptor signaling pathway in human colon cancer HT-29 cells. J Korean Soc Food Sci Nutr. 2003; 32:437–443.


22. Huang RF, Wei YJ, Inbaraj BS, Chen BH. Inhibition of colon cancer cell growth by nanoemulsion carrying gold nanoparticles and lycopene. Int J Nanomedicine. 2015; 10:2823–2846.
23. Tang FY, Shih CJ, Cheng LH, Ho HJ, Chen HJ. Lycopene inhibits growth of human colon cancer cells via suppression of the Akt signaling pathway. Mol Nutr Food Res. 2008; 52:646–654.


24. Lin MC, Wang FY, Kuo YH, Tang FY. Cancer chemopreventive effects of lycopene: suppression of MMP-7 expression and cell invasion in human colon cancer cells. J Agric Food Chem. 2011; 59:11304–11318.


25. Triantafilou M, Triantafilou K. The dynamics of LPS recognition: complex orchestration of multiple receptors. J Endotoxin Res. 2005; 11:5–11.


26. Hung CF, Huang TF, Chen BH, Shieh JM, Wu PH, Wu WB. Lycopene inhibits TNF-alpha-induced endothelial ICAM-1 expression and monocyte-endothelial adhesion. Eur J Pharmacol. 2008; 586:275–282.


27. Bessler H, Salman H, Bergman M, Alcalay Y, Djaldetti M. In vitro effect of lycopene on cytokine production by human peripheral blood mononuclear cells. Immunol Invest. 2008; 37:183–190.


28. Feng D, Ling WH, Duan RD. Lycopene suppresses LPS-induced NO and IL-6 production by inhibiting the activation of ERK, p38MAPK, and NF-κB in macrophages. Inflamm Res. 2010; 59:115–121.


29. Rafi MM, Yadav PN, Reyes M. Lycopene inhibits LPS-induced proinflammatory mediator inducible nitric oxide synthase in mouse macrophage cells. J Food Sci. 2007; 72:S069–S074.


30. Bonizzi G, Karin M. The two NF-κB activation pathways and their role in innate and adaptive immunity. Trends Immunol. 2004; 25:280–288.


31. Reifen R, Nur T, Matas Z, Halpern Z. Lycopene supplementation attenuates the inflammatory status of colitis in a rat model. Int J Vitam Nutr Res. 2001; 71:347–351.


32. Riso P, Visioli F, Grande S, Guarnieri S, Gardana C, Simonetti P, Porrini M. Effect of a tomato-based drink on markers of inflammation, immunomodulation, and oxidative stress. J Agric Food Chem. 2006; 54:2563–2566.


33. Vrieling A, Voskuil DW, Bonfrer JM, Korse CM, van Doorn J, Cats A, Depla AC, Timmer R, Witteman BJ, van Leeuwen FE, Van't Veer LJ, Rookus MA, Kampman E. Lycopene supplementation elevates circulating insulin-like growth factor binding protein-1 and -2 concentrations in persons at greater risk of colorectal cancer. Am J Clin Nutr. 2007; 86:1456–1462.


34. Levy J, Bosin E, Feldman B, Giat Y, Miinster A, Danilenko M, Sharoni Y. Lycopene is a more potent inhibitor of human cancer cell proliferation than either alpha-carotene or beta-carotene. Nutr Cancer. 1995; 24:257–266.


35. Zhang N, Ahsan MH, Zhu L, Sambucetti LC, Purchio AF, West DB. NF-kappaB and not the MAPK signaling pathway regulates GADD45beta expression during acute inflammation. J Biol Chem. 2005; 280:21400–21408.


36. Palozza P, Parrone N, Catalano A, Simone R. Tomato lycopene and inflammatory cascade: basic interactions and clinical implications. Curr Med Chem. 2010; 17:2547–2563.

