Abstract
BACKGROUND/OBJECTIVES
Obesity-induced steatohepatitis accompanied by activated hepatic macrophages/Kupffer cells facilitates the progression of hepatic fibrinogenesis and exacerbates metabolic derangements such as insulin resistance. Heme oxyganase-1 (HO-1) modulates tissue macrophage phenotypes and thus is implicated in protection against inflammatory diseases. Here, we show that the flavonoid quercetin reduces obesity-induced hepatic inflammation by inducing HO-1, which promotes hepatic macrophage polarization in favor of the M2 phenotype.
MATERIALS/METHODS
Male C57BL/6 mice were fed a regular diet (RD), high-fat diet (HFD), or HFD supplemented with quercetin (HF+Que, 0.5g/kg diet) for nine weeks. Inflammatory cytokines and macrophage markers were measured by ELISA and RT-PCR, respectively. HO-1 protein was measured by Western blotting.
RESULTS
Quercetin supplementation decreased levels of inflammatory cytokines (TNFα, IL-6) and increased that of the anti-inflammatory cytokine (IL-10) in the livers of HFD-fed mice. This was accompanied by upregulation of M2 macrophage marker genes (Arg-1, Mrc1) and downregulation of M1 macrophage marker genes (TNFα, NOS2). In co-cultures of lipid-laden hepatocytes and macrophages, treatment with quercetin induced HO-1 in the macrophages, markedly suppressed expression of M1 macrophage marker genes, and reduced release of MCP-1. Moreover, these effects of quercetin were blunted by an HO-1 inhibitor and deficiency of nuclear factor E2-related factor 2 (Nrf2) in macrophages.
Obesity is a major risk factor for non-alcoholic fatty liver diseases (NAFLD). Macrophages/Kupffer cells are resident macrophages in the liver and are essential in both homeostasis and disease [1]. Excessive hepatic lipid accumulation in obesity promotes activation of hepatic macrophages/Kupffer cells, a major step in initiating hepatic inflammation, and steatohepatitis exacerbates insulin resistance and facilitates progression of non-alcoholic steatohepatitis (NASH) [23]. It is likely that the hepatic inflammatory responses largely depend on the macrophage phenotypes, which are characterized by their activation/polarization state into classically activated (M1) proinflammatory macrophages or alternatively-activated (M2) anti-inflammatory macrophages [45]. M1 macrophages are characterized by production of nitrogen oxide and other inflammatory cytokines such as tumor necrosis factor alpha (TNFα), interleukin-6 (IL-6); M2 macrophages are marked by upregulation of Mrc1, CD163, and IL-10 [6]. Macrophages/Kupffer cells in the liver are activated towards M1 phenotype in obese condition [7], whereas M2-polarized macrophages/Kupffer cells in the liver appear to protect against insulin resistance [5], alcoholic liver disease, and NAFLD [8]. Thus, strategies restraining M1 polarization and/or driving the alternative M2 activation of macrophages/Kupffer cells may be beneficial for protecting against hepatic inflammation and the progression of NASH.
Heme oxygenase-1 (HO-1), which is transcribed by activated nuclear factor erythroid 2-related factor 2 (Nrf2), a basic leucine zipper transcription factor [9], is the rate-limiting enzyme in the conversion of heme to biliverdin, releasing free iron and carbon monoxide (CO). It is critical in preventing oxidative stress, inflammation and metabolic dysregulation [91011]. HO-1 inducers such as hemin or substances that produce carbon monoxide such as cobalt protoporphyrin, reduce inflammatory cytokine expression in ob/ob mice and Zucker rats and improve insulin sensitivity in diabetic animals [1213]. Moreover, induction of HO-1 is likely to protect against macrophage-mediated inflammatory responses by preferentially promoting the M2 phenotype [1014]. With respect to this, we and others have shown that HO-1 induction by hemin reduces obesity-induced inflammation by polarizing macrophages in obese adipose tissue [15] and in alcohol-induced hepatic damage [16].
Some dietary components such as curcumin and resveratrol appear to control hepatic inflammation through regulation of HO-1 expression via the Nrf2 pathway [17], and these components affect phenotype switching of macrophages/Kupffer cells in liver [8]. Quercetin is a flavonoid that is abundant in fruits and vegetables such as apples, grapes, and onions. It possesses antioxidant and anti-inflammatory properties, and seems to inhibit obesity-induced inflammation and metabolic disorders such as insulin resistance in genetically and/or diet-induced obese rodents [181920]. We previously showed that it reduced obesity-induced inflammatory responses in both adipose tissue and skeletal muscle by inhibiting activation of inflammatory signaling pathways [2122]. It also protects against lipid accumulation, inflammation, fibrosis, and insulin resistance associated with NAFLD [232425]. However, the mechanism by which quercetin protects against obesity-induced steatohepatitis, in terms of its association with hepatic macrophage polarization, is unclear.
In this study, we demonstrate that quercetin reduces levels of inflammatory cytokines in the fatty liver of HFD-fed obese mice. This effect is associated with its action on HO-1 induction, and leads to polarization of hepatic macrophages toward the M2 phenotype. Furthermore, we show that the Nrf2/HO-1 pathway is involved in these effects. Quercetin may therefore be a useful dietary factor for protecting against obesity-induced steatohepatic inflammation.
Six-week-old (C57BL/6, male) mice were purchased from Orient Ltd. (Orient, Busan, Korea). After a one-week adaptation period, mice were randomly divided into three dietary groups (n = 6 per group). Mice fed on (1) a regular diet (RD) (13% of calories as fat from soybean oil; Harlan Teklad, Madison, WI, USA); (2) a high-fat diet (HFD) (60% of calories as fat from lard and soybean oil; Research Diets, New Brunswick, NJ, USA); and (3) an HFD supplemented with 0.05% (w/w) quercetin (HFD+0.05% Que) for 9 weeks. Quercetin was purchased from Jena Bioscience (Jena, Germany) with a purity of > 98%. All animal care and procedures were conducted according to the protocols and guidelines approved by the University of Ulsan Animal Care and Use Committee (2011-HA-003). The animals were killed by CO2 asphyxiation, and their livers were dissected.
Mouse primary hepatocytes were isolated and cultured using Life Technologies liver media and reagents (Grand Island, NY, USA) as described. The livers of anesthetized 6-8 week-old C57BL/6 male mice were perfused with liver perfusion and digestion medium at a flow rate of 5 ml/min. Digested livers were dissected and dissociated in liver wash medium. After filtration through a 100 µm nylon mesh filter, hepatocytes were purified by repeated centrifugation (three times) at 50 × g for 2 min. Mouse primary hepatocytes on 12-well plates (2 × 105 cells) were incubated with 200 µM free fatty acid, a palmitate coupled to fatty acid-free BSA (molar ratio, 5:1) in DMEM medium (Life technologies, Grand Island, NY, USA) supplemented with 10% fetal bovine serum (FBS, Life technologies), and 1% penicillin-streptomycin (Life technologies) for 12 h. Murine macrophage Raw 264.7 cells were obtained from the Korean Cell Line Bank (KCLB40071, Seoul, Korea) and cultured in RPMI-1640 medium (Life Technologies) supplemented with 10% FBS, 1% penicillin-streptomycin and 0.2% gentamicin (Life Technologies) at 37℃ and 5% CO2. Raw 264.7 macrophages (8 × 104 cells) were plated with the lipid-laden hepatocytes, and the co-cultures were incubated in serum-free DMEM for 12 h in the presence of 20 µM of quercetin and/or 0.1 µM ZnPP, an HO-1 inhibitor.
Nrf2-deficient mice (B6/SJL background) were injected i.p. with 2 ml of 3% thioglycollate four days prior to being killed. Peritoneal macrophages were collected by lavaging the peritoneal cavity with 7 ml of HBSS. The cells were collected by centrifugation, washed, and suspended in EMEM medium supplemented with 10% FBS, 1% penicillin-streptomycin and 0.2% gentamicin. The cells were purified by adherence to tissue culture plates for 2 h. Peritoneal macrophages at 2 × 106 cells/ml were treated with 100 ng/ml LPS, 20 µM of quercetin for 24 h in serum-free medium.
Liver tissues were homogenized in lysis buffer (100 mM Tris-HCl and 250 mM sucrose, pH 7.4) with protease inhibitors (Sigma, St. Louis, MO, USA) and centrifuged at 10,000 g for 10 min. The levels of cytokines in the homogenates were evaluated according to the manufacture's instruction with a TNFα, MCP-1 ELISA set (BD Biosciences, San Diego, CA, USA) and an IL-6, IL-10 ELISA kit (R&D Systems, Minneapolis, MN, USA). Amounts of cytokine were adjusted for protein content, which was determined by using a BCA protein assay kit (Pierce, Rockford, IL, USA).
Total RNA was extracted from liver tissue and cultured cells by using a Trizol reagent (Life technologies, Carlsbad, CA, USA) and then reverse-transcribed into cDNA using M-MLV reverse transcriptase (Promega, Madison, WI, USA). For target genes expression analysis, cDNA was amplified using a SYBR premix Ex Taq kit (TaKaRa Bio Inc., Foster, CA, USA) using a Thermal Cycler Dice (TaKaRa Bio Inc., Japan). Results were analyzed with real-time system TP800 software (Takara Bio Inc.) and all values for genes were normalized to values for a housekeeping gene. The primers used in the analysis are listed in Table 1.
Samples of equal amounts were separated on SDS-PAGE and transferred onto nitrocellulose membranes (Millipore, Tullagreen, Carrigtwohill, Ireland). After incubation with antibodies against HO-1 (Enzo Life Sciences, Farmingdale, NY, USA) and β-actin (Sigma), membrane proteins were detected by HRP-conjugated secondary antibody (Cell Signaling, Beverly, MA, USA), and enhanced chemiluminescent substrate kit (PerkinElmer, Waltham, MA, USA). Intensity of protein bands was quantified by densitometry using Image J program.
Results are presented as means ± SEMs. Statistical comparisons were performed using one-way analysis of variance (ANOVA) with Dunnett and/or Student's t test using GraphPad Prism 5 (San Deiego, CA, USA). Null hypotheses of no difference were rejected if P-values were less than 0.05.
Our previous study demonstrated that quercetin did not alter food intake, and the HFD-fed mice supplemented with quercetin tended to gain less weight than the control HFD-fed mice [26]. In this study, we first examined whether quercetin supplementation reduced hepatic inflammation in HFD-fed obese mice. The obese mice supplemented with quercetin had lower levels of inflammatory cytokines (TNFα, and IL-6) in their livers than the HFD-fed controls (Fig. 1A) and higher levels of the anti-inflammatory cytokine, IL-10 (Fig. 1B). Quercetin had no effect on the expression of the macrophage marker F4/80 (Fig. 1C). To examine the subsets of macrophages/Kupffer cells, we further analyzed levels of the transcripts of macrophage phenotype specific markers. Dietary quercetin suppressed TNFα, and NOS2 transcripts, which are markers of M1 macrophages, (Fig. 1D) and increased those of the M2 macrophage markers Arg-1, and Mrc1 (Fig. 1E).
To test for involvement of HO-1 in the effect of quercetin on the phenotypes of hepatic macrophages, we first confirmed that quercetin treatment upregulated HO-1 protein in macrophages (Fig. 2A), as previously reported [27]. Next, we co-cultured lipid-laden hepatocytes and macrophages to mimic steatohepatitis, and found that co-cultures resulted in increased inflammatory responses. Quercetin markedly reduced levels of MCP-1 release and expression of the M1 markers TNFα and NOS2 in the co-cultures (Fig. 2B-C), and these effects were antagonized by the HO-1 inhibitor ZnPP (Fig. 2B-C).
The Nrf2, a transcriptional regulator of HO-1, is important in the regulation of oxidative stress and the inflammatory state. We examined whether the HO-1 induction by quercetin occurred via the Nrf2 pathway. When we isolated peritoneal macrophages from Nrf2-deficient and wild-type mice, and stimulated them with LPS to induce macrophage M1 polarization and inflammation, we found that quercetin markedly increased HO-1 expression in the wild type and much less so in Nrf2-deficient mice (Fig. 3A). Along with this, we observed that quercetin suppressed the transcript levels of M1 macrophage marker CD274, and enhanced M2 macrophages marker CD163 in the LPS-stimulated WT peritoneal macrophages (Fig. 3B-C), but not in those from the Nrf2-deficient mice (Fig. 3B-C).
Obesity-induced steatohepatitis is characterized by increased activation of hepatic macrophages/Kupffer cells and infiltration of circulating monocytes/macrophages [2829]. In this situation, modulation of the inflammatory responses of hepatic macrophages might be beneficial for protection against inflammatory liver disease progression. In this study, we found that quercetin reduced levels of the inflammatory cytokines TNFα and IL-6, and enhanced the anti-inflammatory cytokine IL-10 in the livers of HFD-fed obese mice, indicating that quercetin protects against obesity-induced hepatic inflammation. Because hepatic inflammatory cytokine production is largely responsible for the activation/polarization state of hepatic macrophages in terms of M1 and M2 phenotypes [4530], we inquired whether quercetin promoted phenotype switching of M1/M2 macrophages. We indeed found that quercetin supplementation markedly reduced transcripts of M1 marker genes such as TNFα, and NOS2 and enhanced those of M2 markers such as Arg-1, and Mrc1. This indicates that the beneficial effect of quercetin on hepatic inflammatory responses in the HFD-fed obese mice may be attributed to polarizing macrophages towards the M2 phenotype. Alternatively, hepatic lipid accumulation promotes macrophages/Kupffer cells activation, which initiates and perpetuates the inflammatory responses [2831]. Given that quercetin supplementation reduces hepatic lipid accumulation in the HFD-fed obese mice by enhancing mitochondrial oxidative capacity [32], the beneficial effect of quercetin may be partly associated with reduction of hepatosteatosis.
Quercetin reduces the production of lipopolysaccharide-stimulated inflammatory mediators such as nitric oxide, TNFα, IL-1β, and IL-6 in Raw 264.7 macrophages [2733], and does so by inducing HO-1 and disrupting activation of components of inflammatory signaling pathways such as phosphatidylinositol 3-kinase [2733]. Consistent with these findings, we found that quercetin enhanced expression of HO-1 in macrophages. Because HO-1 preferentially promotes the M2 phenotype [1014], we thought that quercetin might promote macrophage polarization by inducing HO-1. We confirmed this in experiments on co-cultures of lipid-laden hepatocytes/macrophages mimicking steatohepatitis. Furthermore, given that HO-1 expression is regulated by the activation of transcription factor Nrf2 [9], we inquired whether the quercetin action is mediated by Nrf2/HO-1 pathway. Indeed, we found that quercetin-induced alteration in M1/M2 phenotype switching: reduction of M1 marker and increase of M2 marker was completely blunted by Nrf2-deficient macrophages. More importantly, the effect of quercetin on the induction of HO-1 was completely disrupted in the LPS-treated peritoneal macrophages of the Nrf2-deficient mice. Our findings suggest that quercetin promotes macrophage M2 polarization and this effect is mediated, at least in part, by HO-1 induction, which is dependent on activation of the transcription factor Nrf2.
In conclusion, quercetin reduces obesity-induced steatohepatic inflammation and this is accompanied by macrophage phenotype switching towards the M2 phenotype dependent on the induction of HO-1 by the transcription factor Nrf2. Quercetin may be a useful dietary component to reduce obesity-induced hepatic inflammation.
Figures and Tables
Fig. 1
Effects of quercetin on hepatic inflammatory responses and macrophage phenotypes in HFD-fed obese mice.
(A) Inflammatory cytokines (TNFα, IL-6, and MCP-1) and (B) an anti-inflammatory cytokine (IL-10) in liver were determined as described in Methods. Results are means ± SEM of six mice per group. * P < 0.05, ** P < 0.01, *** P < 0.001 compared with obese mice fed an HFD. (C) F4/80 as a macrophage marker and (D-E) M1 and M2 macrophages mRNAs in livers were determined by real-time PCR. The intensity of the bands was densitometrically measured and normalized to GAPDH transcripts. Results are means ± SEM of six mice per group. * P < 0.05, ** P < 0.01 compared with obese mice fed an HFD.
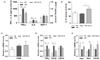
Fig. 2
Effect of HO-1 inhibition on quercetin action in co-cultures of lipid-laden hepatocytes with macrophages.
Primary hepatocytes were treated with palmitic acid (BSA: palmitic acid ratio 1:5) for 12h. Raw 264.7 macrophages were then co-cultured with the lipid-laden hepatocytes for 12h in the presence or absence of quercetin or ZnPP. L-Hepa; lipid-laden hepatocytes, Co-culture; lipid-laden hepatocytes and macrophages, Que; quercetin. (A) HO-1 protein was measured by western blotting in macrophages. Results are means ± SEM of two experiments with duplicate determinations. * P < 0.05, *** P < 0.001 compared with no treatment. (B) MCP-1 production detected by ELISA in co-cultured lipid-laden hepatocytes and macrophages. Results are means ± SEM of triplicate samples. * P < 0.05, *** P < 0.001 compared with quercetin treatment. (C) M1 macrophage mRNAs in co-cultured lipid-laden hepatocytes and macrophages determined by real-time PCR. Results are means ± SEM of quadruplicate samples. * P < 0.05, ** P < 0.01, *** P < 0.001 compared with quercetin treatment.
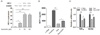
Fig. 3
Effect of Nrf2 deficiency on quercetin action in LPS-stimulated macrophages.
(A) HO-1 protein was measured by western blotting in peritoneal macrophages of Nrf2 KO and WT mice. Results are means ± SEM of three independent experiments. ** P < 0.01 compared with LPS treatment. (B-C) M1/M2 marker mRNAs were determined by real-time PCR. The intensity of the bands was densitometrically measured and normalized to GAPDH transcripts. Results are means ± SEM of two experiments with duplicate determinations. * P < 0.05, ** P < 0.01, *** P < 0.001 compared with LPS treatment.
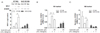
Notes
References
1. Bilzer M, Roggel F, Gerbes AL. Role of Kupffer cells in host defense and liver disease. Liver Int. 2006; 26:1175–1186.


3. Lanthier N, Molendi-Coste O, Horsmans Y, van Rooijen N, Cani PD, Leclercq IA. Kupffer cell activation is a causal factor for hepatic insulin resistance. Am J Physiol Gastrointest Liver Physiol. 2010; 298:G107–G116.


4. Mantovani A, Sica A, Sozzani S, Allavena P, Vecchi A, Locati M. The chemokine system in diverse forms of macrophage activation and polarization. Trends Immunol. 2004; 25:677–686.


5. Lumeng CN, Bodzin JL, Saltiel AR. Obesity induces a phenotypic switch in adipose tissue macrophage polarization. J Clin Invest. 2007; 117:175–184.


6. Dixon LJ, Barnes M, Tang H, Pritchard MT, Nagy LE. Kupffer cells in the liver. Compr Physiol. 2013; 3:785–797.


7. Odegaard JI, Ricardo-Gonzalez RR, Red Eagle A, Vats D, Morel CR, Goforth MH, Subramanian V, Mukundan L, Ferrante AW, Chawla A. Alternative M2 activation of Kupffer cells by PPARdelta ameliorates obesity-induced insulin resistance. Cell Metab. 2008; 7:496–507.


8. Wan J, Benkdane M, Teixeira-Clerc F, Bonnafous S, Louvet A, Lafdil F, Pecker F, Tran A, Gual P, Mallat A, Lotersztajn S, Pavoine C. M2 Kupffer cells promote M1 Kupffer cell apoptosis: a protective mechanism against alcoholic and nonalcoholic fatty liver disease. Hepatology. 2014; 59:130–142.


9. Alam J, Stewart D, Touchard C, Boinapally S, Choi AM, Cook JL. Nrf2, a Cap'n'Collar transcription factor, regulates induction of the heme oxygenase-1 gene. J Biol Chem. 1999; 274:26071–26078.


10. Lee TS, Chau LY. Heme oxygenase-1 mediates the anti-inflammatory effect of interleukin-10 in mice. Nat Med. 2002; 8:240–246.


11. Ndisang JF. Role of heme oxygenase in inflammation, insulin-signalling, diabetes and obesity. Mediators Inflamm. 2010; 2010:359732.


12. Kim DH, Burgess AP, Li M, Tsenovoy PL, Addabbo F, McClung JA, Puri N, Abraham NG. Heme oxygenase-mediated increases in adiponectin decrease fat content and inflammatory cytokines tumor necrosis factor-alpha and interleukin-6 in Zucker rats and reduce adipogenesis in human mesenchymal stem cells. J Pharmacol Exp Ther. 2008; 325:833–840.


13. L'Abbate A, Neglia D, Vecoli C, Novelli M, Ottaviano V, Baldi S, Barsacchi R, Paolicchi A, Masiello P, Drummond GS, McClung JA, Abraham NG. Beneficial effect of heme oxygenase-1 expression on myocardial ischemia-reperfusion involves an increase in adiponectin in mildly diabetic rats. Am J Physiol Heart Circ Physiol. 2007; 293:H3532–H3541.
14. Alcaraz MJ, Fernández P, Guillén MI. Anti-inflammatory actions of the heme oxygenase-1 pathway. Curr Pharm Des. 2003; 9:2541–2551.


15. Tu TH, Joe Y, Choi HS, Chung HT, Yu R. Induction of heme oxygenase-1 with hemin reduces obesity-induced adipose tissue inflammation via adipose macrophage phenotype switching. Mediators Inflamm. 2014; 2014:290708.


16. Yao P, Hao L, Nussler N, Lehmann A, Song F, Zhao J, Neuhaus P, Liu L, Nussler A. The protective role of HO-1 and its generated products (CO, bilirubin, and Fe) in ethanol-induced human hepatocyte damage. Am J Physiol Gastrointest Liver Physiol. 2009; 296:G1318–G1323.


17. Son Y, Lee JH, Chung HT, Pae HO. Therapeutic roles of heme oxygenase-1 in metabolic diseases: curcumin and resveratrol analogues as possible inducers of heme oxygenase-1. Oxid Med Cell Longev. 2013; 2013:639541.


18. Anjaneyulu M, Chopra K. Quercetin, an anti-oxidant bioflavonoid, attenuates diabetic nephropathy in rats. Clin Exp Pharmacol Physiol. 2004; 31:244–248.


19. Overman A, Chuang CC, McIntosh M. Quercetin attenuates inflammation in human macrophages and adipocytes exposed to macrophage-conditioned media. Int J Obes (Lond). 2011; 35:1165–1172.


20. Panchal SK, Poudyal H, Brown L. Quercetin ameliorates cardiovascular, hepatic, and metabolic changes in diet-induced metabolic syndrome in rats. J Nutr. 2012; 142:1026–1032.


21. Noh HJ, Kim CS, Kang JH, Park JY, Choe SY, Hong SM, Yoo H, Park T, Yu R. Quercetin suppresses MIP-1alpha-induced adipose inflammation by downregulating its receptors CCR1/CCR5 and inhibiting inflammatory signaling. J Med Food. 2014; 17:550–557.


22. Le NH, Kim CS, Park T, Park JH, Sung MK, Lee DG, Hong SM, Choe SY, Goto T, Kawada T, Yu R. Quercetin protects against obesity-induced skeletal muscle inflammation and atrophy. Mediators Inflamm. 2014; 2014:834294.


23. Ying HZ, Liu YH, Yu B, Wang ZY, Zang JN, Yu CH. Dietary quercetin ameliorates nonalcoholic steatohepatitis induced by a high-fat diet in gerbils. Food Chem Toxicol. 2013; 52:53–60.


24. Li X, Wang R, Zhou N, Wang X, Liu Q, Bai Y, Bai Y, Liu Z, Yang H, Zou J, Wang H, Shi T. Quercetin improves insulin resistance and hepatic lipid accumulation in vitro in a NAFLD cell model. Biomed Rep. 2013; 1:71–76.


25. Marcolin E, San-Miguel B, Vallejo D, Tieppo J, Marroni N, González-Gallego J, Tuñón MJ. Quercetin treatment ameliorates inflammation and fibrosis in mice with nonalcoholic steatohepatitis. J Nutr. 2012; 142:1821–1828.


26. Kim CS, Yu R. The inhibitory effect of quercetin on adipose tissue inflammation in mice fed on a high-fat diet. Korean J Obes. 2014; 23:170–178.


27. Lin HY, Juan SH, Shen SC, Hsu FL, Chen YC. Inhibition of lipopolysaccharide-induced nitric oxide production by flavonoids in RAW264.7 macrophages involves heme oxygenase-1. Biochem Pharmacol. 2003; 66:1821–1832.


28. Marra F, Lotersztajn S. Pathophysiology of NASH: perspectives for a targeted treatment. Curr Pharm Des. 2013; 19:5250–5269.


29. Tosello-Trampont AC, Landes SG, Nguyen V, Novobrantseva TI, Hahn YS. Kuppfer cells trigger nonalcoholic steatohepatitis development in diet-induced mouse model through tumor necrosis factor-alpha production. J Biol Chem. 2012; 287:40161–40172.


30. Dong J, Zhang X, Zhang L, Bian HX, Xu N, Bao B, Liu J. Quercetin reduces obesity-associated ATM infiltration and inflammation in mice: a mechanism including AMPKalpha1/SIRT1. J Lipid Res. 2014; 55:363–374.


31. Matsuzawa N, Takamura T, Kurita S, Misu H, Ota T, Ando H, Yokoyama M, Honda M, Zen Y, Nakanuma Y, Miyamoto K, Kaneko S. Lipid-induced oxidative stress causes steatohepatitis in mice fed an atherogenic diet. Hepatology. 2007; 46:1392–1403.


32. Kim CS, Kwon Y, Choe SY, Hong SM, Yoo H, Goto T, Kawada T, Choi HS, Joe Y, Chung HT, Yu R. Quercetin reduces obesity-induced hepatosteatosis by enhancing mitochondrial oxidative metabolism via heme oxygenase-1. Nutr Metab (Lond). 2015; 12:33.


33. Endale M, Park SC, Kim S, Kim SH, Yang Y, Cho JY, Rhee MH. Quercetin disrupts tyrosine-phosphorylated phosphatidylinositol 3-kinase and myeloid differentiation factor-88 association, and inhibits MAPK/AP-1 and IKK/NF-kappaB-induced inflammatory mediators production in RAW 264.7 cells. Immunobiology. 2013; 218:1452–1467.

