Abstract
BACKGROUND/OBJECTIVES
Bone formation and bone resorption continuously occur in bone tissue to prevent the accumulation of old bone, this being called bone remodeling. Osteoblasts especially play a crucial role in bone formation through the differentiation and proliferation. Therefore, in this study, we investigated the effects of Scytosiphon lomentaria extract (SLE) on osteoblastic proliferation and differentiation in MC3T3-E1 cells.
MATERIALS/METHODS
A cell proliferation assay, alkaline phosphatase (ALP) activity assay, alizarin red staining and protein expression analysis of osteoblastic genes were carried out to assess the osteoblastic proliferation and differentiation.
RESULTS
The results indicated that treatment of SLE promoted the proliferation of MC3T3-E1 cells and improved ALP activity. And, SLE treatment significantly promoted mineralized nodule formation compared with control. In addition, cells treated with SLE significantly upregulated protein expression of ALP, type 1 collagen, bone morphogenetic protein 2, runt-related transcription factor 2, osterix, and osteoprotegerin.
Our skeleton is continually renewed at the rate of about 10% per year [1]. Osteoporosis is a disease resulting from decreased renewal of bone, which leads to less dense, lose strength and break more easily of skeleton. The etiology of osteoporosis is complex and fracture risk is influenced both by the familial effects and by environmental factors, with lifestyle becoming more important for bone mass and osteoporotic fractures with increasing age [12]. With increasing age, there has been a significant reduction in bone mass due to tipping of this delicate balance towards increased resorption combined with decreased formation. Also, this can lead to net bone loss in the aging peoples that ultimately induces as osteoporosis [3].
The balance between bone formation and bone resorption is essential for maintaining bone homeostasis [4]. Recent therapy for osteoporosis has mainly been focused on inhibiting osteoclast activity by using bisphosphonates and selective estrogen receptor modulators to prevent further bone loss [5]. But, many patients have already lost bone mass before receiving any treatment. Bone formation therefore needs to be accelerated to treat osteoporosis. Since new bone formation is primarily a function of the osteoblast, agents that act by either increasing the proliferation of cells of the osteoblastic lineage or inducing differentiation of the osteoblasts can enhance bone formation [6]. Osteoblast differentiation, an important process for its function, confers marked rigidity and strength to the bone while still maintaining some degree of elasticity. It is regulated by the action of key transcription factors, including runt-related transcription factor 2 (Runx2) and osterix (Osx), accompanied by the increased expression of bone matrix proteins such as alkaline phosphatase (ALP) and type 1 collagen (Col 1). These osteoblast differentiation factors stimulate mineralization and lead to bone formation [7]. In addition, bone morphogenetic protein (BMP) signaling pathways cooperatively control the osteogenesis and osteoblast differentiation [8].
Recently, there has been an increasing interest in the utilization of natural products in the treatment of diseases since they are basically safe and inexpensive. Moreover, numerous edible natural products are showing tremendous potential for the treatment of bone-related diseases. The brown alga Scytosiphon lomentaria is plentifully produced on southern sea coast in Korea. Also, S. lomentaria contains fucoxanthin, which has anti-inflammation and the antioxidant activity [9]. One study found that fucoxanthin content is greater in brown algae than that in Rhodophyta or Chlorophyta [10]. However, effects of Scytosiphon lomentaria extract (SLE) on proliferation and differentiation of osteoblastic cells have not yet to be determined. Thus, in this study we investigated whether SLE regulates the proliferation and osteogenic differentiation of MC3T3-E1 cells.
Alpha modification of Eagle's minimum essential medium (α-MEM), fetal bovine serum (FBS) and tissue culture reagents were purchased from Gibco BRL (Carlsbad, USA). All other reagents were of analytical grade and purchased from Sigma Chemicals Co. (St. Louis, USA). Antibody against Col 1, BMP-2, and Osx were purchased from Abcam (Cambridge, USA). Antibody against ALP, OPG, RANKL, Runx2, and β-actin were purchased from Cell signaling Technology (Beverly, USA).
Scytosiphon lomentaria was purchased from Parajeju Inc. (Seoul, Korea). The sample was washed three times with tap water to remove the salt, epiphytes, and sand attached to the surface, then carefully rinsed with fresh water and maintained in a deep freezer at -80℃. Thereafter, the frozen samples were lyophilized and homogenized with a grinder prior to extraction. Scytosiphon lomentaria (100 g) was extracted with twenty volumes of distilled water for 4 h three times at 60℃. The filtrates were concentrated by rotary vacuum evaporation (Buchi, Zurich, Switzerland) and then lyophilized with a freeze dryer (Ilshin BioBase, Seoul, Korea). The SLE was thoroughly dried for complete removal of solvent and stored in a deep freezer (-80℃).
MC3T3-E1 cells were obtained from the American Type Culture Collection (ATCC, Manassas, USA). The cells were maintained in an α-MEM supplemented with 10% heat-inactivated fetal bovine serum, 1% penicillin at 37℃ in a humidified 5% CO2 incubator. To induce differentiation, MC3T3-E1 cells were seeded into plates or dishes and cultured in minimal medium containing 50 µg/ml L-ascorbic acid along with 5 mM β-glycerophosphate.
Cell proliferation was determined by the MTT method as described previously [11]. In brief, the cells were seeded (5 × 103 cells/96-well), at confluence, with culture medium containing 50 µg/ml L-ascorbic acid and 5 mM β-glycerophosphate to initiate differentiation. After 7 days, the cells were incubated in culture medium containing various concentrations (10, 50 and 100 µg/ml) of SLE for 48 h. After treatment, the medium was discarded and fresh medium containing 5 mg/mL MTT was added for another 4 h. Formazan salts were dissolved in DMSO (100 µL) and absorbance was measured at 550 nm using the ELISA reader.
ALP activity was measured as described previously [12]. Specifically, the cells were grown in 6-well plates in osteogenic medium (minimal medium containing 50 µg/ml L-ascorbic acid along with 5 mM β-glycerophosphate). After 7 days, the cells were incubated in culture medium containing various concentrations (10-100 µg/ml) of SLE for 48 h. After treatment, the cells were harvested, washed twice with PBS, and then lysed with 0.2% Triton X-100. This mixture was then centrifuged at 12,000 rpm for 10 min at 4℃. The supernatants were collected to determine ALP activity and protein concentration. A 100 µl aliquot of lysate supernatant was incubated with 100 µl pNPP (Sigma, St. Louis, USA) and 0.1 N glycine for 30 min at 37℃. The pNPP reaction was stopped with 1 M NaOH and measured at 405 nm using a microplate reader (Bio-Rad, Hercules, USA). Relative ALP activity was normalized to the protein concentration of the samples assayed by the BCA method, and then to the control without treatment.
Alizarin red S staining was performed to analyze calcium deposits. In brief, the cells were grown in 6-well plates in osteogenic medium. After 14 days, the cells were incubated in culture medium containing various concentrations (10-100 µg/ml) of SLE for 48 h. Cells were washed with PBS twice and then fixed with 70% ethanol for 1 h. The cells were stained with 40 mM of Alizarin red S solution (pH 4.2) for 10 min at room temperature and rinsed with distilled water to exclude non-specific staining. For quantitation, cells stained with Alizarin red were destained with ethylpyridimium chloride and then the extracted stain was transferred to a 96-well plate, and the absorbance at 562 nm was measured using a microplate reader [13].
The cells were grown in 10 mm dishes in osteogenic medium. After 7 days, the cells were incubated in culture medium containing various concentrations (10-100 µg/ml) of SLE for 48 h. Cells were homogenized with ice-cold lysis buffer containing 250 mM NaCl, 25 mM Tris-HCl (pH 7.5), 1% v/v NP-40, 1 mM dithiothreitol (DTT), 1 mM phenylmethylsulfonyl fluoride (PMSF), and protein inhibitor cocktail (10 µg/ml aprotinin, 1 µg/ml leupeptin). The cells were then centrifuged at 20,000 g for 15 min at 4℃. The supernatants were used as total protein extracts. The total protein contents were determined by the Bio-Rad protein kit with BSA as the standard. The lysate containing 30-50 µg of protein was subjected to electrophoresis on 10% sodium dodecyl sulfate-polyacrylamide gel (SDS-PAGE). Separated proteins were transferred electrophoretically to a pure nitrocellulose membrane, blocked with 5% skimmed milk solution for 1 h, and then incubated with primary antibodies overnight at 4℃. After washing, the blots were incubated with goat anti-rabbit or goat anti-mouse IgG horseradish peroxidase-conjugated (HRP-conjugated) secondary antibody for 1 h at room temperature. Each antigen-antibody complex was visualized using ECL Western Blotting Detection Reagents and detected by chemiluminescence with LAS-1000 plus (FUJIFLIM, Tokyo, Japan). Band densities were determined by an image analyzer (FUJIFLIM, Valhalla, USA) and normalized to β-actin for total protein and nuclear protein.
The data are represented as the mean ± standard deviation (SD) of triplicate experiments. Statistical analyses were performed using SAS 9.1 software (SAS Institute Inc., Cary, USA). The treatment groups were compared by one-way analysis of variance (ANOVA) followed by post hoc Duncan's multiple range tests, and p-values of less than 0.05 were considered statistically significant.
The effect of SLE on the cell proliferation was determined by a MTT assay. Compared to control, the SLE showed stimulatory effects on osteoblastic cell proliferation at all tested concentrations from 10 to 100 µg/ml (Fig. 1). In particular, treatment with 100 µg/ml of SLE treatment resulted in a significant increase in cell proliferation, to 126.7%. Thus, these results indicated that SLE promoted osteoblastic cell proliferation.
We examined the effect of SLE on ALP activity as a marker of osteoblast differentiation. ALP is a well-recognized marker of osteoblast differentiation. As shown in Fig. 2, SLE treatment significantly increased ALP activity in a dose-dependent manner. The maximal effect was observed at 100 µg/ml of SLE, which increased ALP activity by 34.3%, as compared to the control. These findings suggest that SLE stimulates osteoblast differentiation.
Mineralized bone nodule formation is an osteoblast maturation marker [14]. The effect of SLE on mineralization in cells was determined by alizarin red S staining and a microscopic analysis. Alizarin red S staining of the cells demonstrated a mineralized extracellular matrix as a calcified deposit. The addition of SLE significantly and concentration-dependently enhanced bone nodule formation in MC3T3-E1 cells when compared with that in the control observed (Fig. 3). At 100 µg/ml of SLE the mineralization level was significantly increased by 24.8%, as compared to the control (Fig. 3B). Thus, SLE effectively promoted markers of osteoblast maturation, which resulted in stimulated osteoblast differentiation in MC3T3-E1 cells.
The osteoblast-associated molecules ALP and Col 1 are considered osteoblast differentiation markers, as their expression levels increased during osteoblast differentiation [1516]. To investigate the effect of SLE on osteoblast differentiation in MC3T3-E1 cells, protein levels of ALP and Col 1 were examined by western blot. The protein expression levels of ALP and Col 1 were significantly increased by SLE treatment in a dose-dependent manner (Fig. 4). These results suggest that SLE promotes mineralization and osteogenesis by increasing markers of osteoblast differentiation.
Osteoblasts can secrete OPG to protect the skeleton from excessive bone resorption by binding to RANKL and preventing it from binding to its receptor, RANK. Thus, the expression of OPG/RANKL ratio is an informative marker for evaluation of effects on bone resorption and bone formation [17]. SLE significantly increased OPG protein levels at a concentration of 50 and 100 µg/ml, when compared to the control (Fig. 5). On the other hand, SLE treatment simultaneously decreased the protein expression of RANKL. Consequently, SLE treatment increases the expression of OPG/RANKL ratio, which can result in inhibition of osteoclastogenesis.
The BMP signaling pathway promotes the differentiation of mesenchymal cells into an osteoblastic lineage, as suggested by its ability to stimulate the expression of osteoblast differentiation markers [18]. SLE treatment significantly activated the protein expression of genes in the BMP signaling pathway-related regulator, including BMP2, Runx2, and Osx (Fig. 6). SLE treatment effectively regulated the expression of the BMP signaling pathway components, leading to stimulation of osteoblast differentiation.
Osteoporosis is a systemic skeletal disease characterized by decreased bone mass and loss of bone tissue that may lead to weak and fragile bones [19]. The current clinical treatment regimens for osteoporosis are anti-resorptive drugs, which maintain bone mass by inhibiting osteoclast function. However, the effects of these drugs on bone mineral loss and recovery is comparatively minor by about 2% per year [20]. Because new bone formation is primarily mediated by osteoblasts, agents that act to either increasing osteoblast proliferation or inducing osteoblast differentiation enhance bone formation [21]. A wide variety of natural materials with therapeutic effects on bone formation and skeleton construction have been reported and several enhance osteogenic differentiation [22]. Marine algae are known to contain an abundance of bioactive compounds with great pharmaceutical, nutritive, and biomedical potential. Among them, Scytosiphon lomentaria is a potential candidate for promoting bone formation [23]. But, effects of Scytosiphon lomentaria extract on proliferation and differentiation of osteoblastic cells have not yet to be determined. Therefore, in the present study, we investigated whether SLE stimulates the proliferation and osteogenic differentiation of MC3T3-E1 cells.
A decrease in osteoblastic proliferation is an important factor in the pathogenesis of osteoporosis [24]. The effect of SLE on cell proliferation was assayed by using MTT assay. As shown in figure 1, 10-100 µg/mL of the SLE increased in MC3T3-E1 cell proliferation dose dependently. Thus, these results indicated that SLE stimulated cell proliferation and had no cytotoxicity toward the cells.
The process of bone formation includes initial osteoblast proliferation followed by increased ALP activity, development and maturation of the extracellular matrix and mineralization [25]. ALP is a phenotypic marker and essential enzyme, which produces and up-regulates specific osteoblast differentiation genes, including Col 1 and osteopontin (OPN) [26]. Also, Col 1 is the most abundant protein synthesized by the active osteoblasts and its expression represents the begining of the osteoblasts differentiation [27]. ALP activities in SLE-treated cells were promoted significantly, and this effect was concentration dependent (Fig. 2). In addition, the protein expression levels of ALP and Col 1 were significantly increased by SLE treatment in a dose-dependent manner (Fig. 4). These findings demonstrate that SLE enhances the osteoblast differentiation process from the early to the late stage, up-regulating differentiation in MC3T3-E1 cells.
In addition to osteoblast proliferation and differentiation, such as ALP activity, extracellular matrix mineralization is also one of the major parts of osteogenesis, and we assessed whether SLE treatment to MC3T3-E1 cells stimulated matrix mineralization. Mineralization of extracellular matrix as Ca deposits for mineralized nodule formation was assessed by Alizarin red staining which combines with Ca ions. SLE significantly promoted mineralization in a dose-dependent manner (Fig. 3). These results indicated that SLE-treated cells showed increased Ca deposits as compared to the controls, which indicate stimulation of matrix mineralization.
The unbalance between OPG and RANKL has been indicated as a crucial mechanism responsible for bone loss [28]. RANKL, expressed by osteoblasts, plays a pivotal role in activation of osteoclasts through binding to its receptor RANK. OPG, a decoy receptor for the RANKL, is expressed by osteoblasts. The secretion of OPG by osteoblasts prevents RANKL-RANK interaction and RANK activation, resulting in inhibition of bone resorption [1429]. Thus, the OPG/RANKL ratio is critical to the coupling of bone resorption to bone remodeling. We found that OPG protein expression could be increased significantly and RANKL protein expression could be decreased significantly when MC3T3-E1 cells were exposed to various concentrations of SLE (10, 50 and 100 µg/ml). So, SLE increased the protein expression of OPG/RANKL ratio in MC3T3-E1 cells (Fig. 5), indicating that SLE inhibited osteoclastogenesis by regulating the balance of OPG/RANKL.
The BMP signaling pathway induces bone formation and bone remodeling by stimulating the differentiation of osteoblast [30]. As shown Fig. 6, SLE increased the protein expression of BMP signaling pathway components, such as BMP2, Runx2, and Osx. BMPs are one of the factors that play a major role in the growth and differentiation of osteoblastic cells, BMPs being potent stimulators of bone formation in various animal models [31]. Runx2 is one of the osteogenic master transcription factors, and its activity is elevated by BMP2 signaling [32]. Osx is also an essential transcription factor for osteoblast differentiation. Consequently, SLE stimulates osteoblast differentiation through activation of the BMP signaling pathway, increasing the expression of the down-stream regulators, Runx2 and Osx.
Various bioactive components in brown algae, such as polysaccharides, carotenoids, and polyphenols, have been suggested to be involved in indicating biological activities. Also, some studies reported that polyphenols delayed onset or reduction in the progression of osteoporosis [3334]. According to O'Gorman et al. [35], the marine-derived, multi-mineral formula, Aquamin enhanced mineralization of osteoblast cells. Taken together, these results suggest that polyphenol-rich SLE is involved in the anti-osteoporosis effects.
In conclusion, SLE promoted osteoblast differentiation and mineralization by regulating osteoblast differentiation factors and the balance of OPG/RANKL by activating the BMP signaling pathways. Therefore, our findings demonstrate that SLE can stimulate osteoblast differentiation, and could be developed as a promising drug with little toxicity to regulate bone metabolism.
Figures and Tables
Fig. 1
Effect of SLE on the proliferation in MC3T3-E1 cells.
Cells were treated with SLE at 10, 50 and 100 µg/ml for 2 days. Cell proliferation was measured using the MTT assay. Data are expressed as percentage of control. Each value is expressed as mean ± SD (n = 3). a-dValues with different letters were significantly different at P < 0.05, as analyzed by Duncan's multiple range test. SLE: Scytosiphon lomentaria extract.
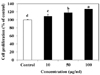
Fig. 2
Effect of SLE on alkaline phosphatase (ALP) activity in MC3T3-E1 cells.
Cells were treated with SLE at 10, 50 and 100 µg/ml for 2 days. Data are expressed as percentage of control. Each value is expressed as mean ± SD (n = 3). a-cValues with different letters were significantly different at P < 0.05, as analyzed by Duncan's multiple range test. SLE: Scytosiphon lomentaria extract.
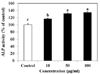
Fig. 3
Effect of SLE on mineralization in MC3T3-E1 cells.
(A) The mineralized matrix was stained with Alizarin red S. (B) Quantitation of mineralization using Alizarin red staining, as described in the materials and methods section. Each value is expressed as mean ± SD (n = 3). a-cValues with different letters were significantly different at P < 0.05, as analyzed by Duncan's multiple range test. SLE: Scytosiphon lomentaria extract.
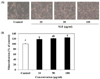
Fig. 4
Effects of SLE on osteoblast differentiation in MC3T3-E1 cells.
Cells were treated with SLE at 10, 50 and 100 µg/ml for 2 days. (A) The protein expression of osteoblast differentiation factors, such as ALP, and Col 1 was detected by western blot. (B) Relative expression was quantified by densitometry using the Multi Gauge V3.1 and calculated according to the reference bands of β-actin. Each value is expressed as mean ± SD (n = 3). a-dValues with different letters were significantly different at P < 0.05, as analyzed by Duncan's multiple range test. SLE: Scytosiphon lomentaria extract.
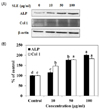
Fig. 5
Effects of SLE on the OPG/RANKL ratio in MC3T3-E1 cells.
Cells were treated with SLE at 10, 50 and 100 µg/ml for 2 days. (A) The protein expression of OPG/RANKL ratio, such as OPG and RANKL was detected by western blot. (B) Relative expression was quantified by densitometry using the Multi Gauge V3.1 and calculated according to the reference bands of β-actin. Each value is expressed as mean ± SD (n = 3). a-cValues with different letters were significantly different at P < 0.05, as analyzed by Duncan's multiple range test. SLE: Scytosiphon lomentaria extract.
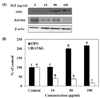
Fig. 6
Effects of SLE on the BMP signaling pathway in MC3T3-E1 cells.
Cells were treated with SLE at 10, 50 and 100 µg/ml for 2 days. (A): The protein expression of the BMP signaling pathway related markers, such as BMP-2, Runx2, and Osx was detected by western blot. (B): Relative expression was quantified by densitometry using the Multi Gauge V3.1 and calculated according to the reference bands of β-actin. Each value is expressed as mean ± SD (n = 3). a-dValues with different letters were significantly different at P < 0.05, as analyzed by Duncan's multiple range test. SLE: Scytosiphon lomentaria extract.
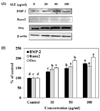
References
1. Michaëlsson K, Melhus H, Ferm H, Ahlbom A, Pedersen NL. Genetic liability to fractures in the elderly. Arch Intern Med. 2005; 165:1825–1830.


2. Slemenda CW, Christian JC, Williams CJ, Norton JA, Johnston CC Jr. Genetic determinants of bone mass in adult women: a reevaluation of the twin model and the potential importance of gene interaction on heritability estimates. J Bone Miner Res. 1991; 6:561–567.


3. Riggs BL, Khosla S, Melton LJ 3rd. Sex steroids and the construction and conservation of the adult skeleton. Endocr Rev. 2002; 23:279–302.


4. Isomura H, Fujie K, Shibata K, Inoue N, Iizuka T, Takebe G, Takahashi K, Nishihira J, Izumi H, Sakamoto W. Bone metabolism and oxidative stress in postmenopausal rats with iron overload. Toxicology. 2004; 197:93–100.


5. Yonezawa T, Hasegawa S, Asai M, Ninomiya T, Sasaki T, Cha BY, Teruya T, Ozawa H, Yagasaki K, Nagai K, Woo JT. Harmine, a β-carboline alkaloid, inhibits osteoclast differentiation and bone resorption in vitro and in vivo. Eur J Pharmacol. 2011; 650:511–518.


6. Lane NE, Kelman A. A review of anabolic therapies for osteoporosis. Arthritis Res Ther. 2003; 5:214–222.
7. Yoon HJ, Seo CR, Kim M, Kim YJ, Song NJ, Jang WS, Kim BJ, Lee J, Hong JW, Nho CW, Park KW. Dichloromethane extracts of Sophora japonica L. stimulate osteoblast differentiation in mesenchymal stem cells. Nutr Res. 2013; 33:1053–1062.


8. Zhang R, Oyajobi BO, Harris SE, Chen D, Tsao C, Deng HW, Zhao M. Wnt/β-catenin signaling activates bone morphogenetic protein 2 expression in osteoblasts. Bone. 2013; 52:145–156.


9. Demirel Z, Yilmaz-Koz FF, Karabay-Yavasoglu UN, Ozdemir G, Sukatar A. Antimicrobial and antioxidant activity of brown algae from the Aegean sea. J Serb Chem Soc. 2009; 74:619–628.


10. Nakamura T, Nagayama K, Kawaguchi S. High tocopherol content in a brown alga Ishige okamurae. Fish Sci. 1994; 60:793–794.
11. Hill PA, Tumber A, Meikle MC. Multiple extracellular signals promote osteoblast survival and apoptosis. Endocrinology. 1997; 138:3849–3858.


12. Tietz NW, Burtis CA, Duncan P, Ervin K, Petitclerc CJ, Rinker AD, Shuey D, Zygowicz ER. A reference method for measurement of alkaline phosphatase activity in human serum. Clin Chem. 1983; 29:751–761.


13. Whyte MP. Hypophosphatasia and the role of alkaline phosphatase in skeletal mineralization. Endocr Rev. 1994; 15:439–461.


14. Niu YB, Li YH, Kong XH, Zhang R, Sun Y, Li Q, Li C, Liu L, Wang J, Mei QB. The beneficial effect of Radix Dipsaci total saponins on bone metabolism in vitro and in vivo and the possible mechanisms of action. Osteoporos Int. 2012; 23:2649–2660.


15. Musiał K, Fornalczyk K, Zwolińska D. [Osteopontin (OPN), PDGF-BB (platelet-derived growth factor) and BMP-7 (bone morphogenetic protein) as markers of atherogenesis in children with chronic kidney disease (CKD) treated conservatively--preliminary results]. Pol Merkur Lekarski. 2008; 24:Suppl 4. 25–27.
16. W W. Glial line-derived neurotrophic factor (GDNF): biological activities. Folia Morphol (Warsz). 1999; 58:155–159.
17. Li F, Yang Y, Zhu P, Chen W, Qi D, Shi X, Zhang C, Yang Z, Li P. Echinacoside promotes bone regeneration by increasing OPG/RANKL ratio in MC3T3-E1 cells. Fitoterapia. 2012; 83:1443–1450.


18. Lee HS, Jung EY, Bae SH, Kwon KH, Kim JM, Suh HJ. Stimulation of osteoblastic differentiation and mineralization in MC3T3-E1 cells by yeast hydrolysate. Phytother Res. 2011; 25:716–723.


19. Christiansen C. Consensus development conference: diagnosis, prophylaxis, and treatment of osteoporosis. Am J Med. 1993; 94:646–650.


21. Ducy P, Schinke T, Karsenty G. The osteoblast: a sophisticated fibroblast under central surveillance. Science. 2000; 289:1501–1504.


22. Hsieh TP, Sheu SY, Sun JS, Chen MH, Liu MH. Icariin isolated from Epimedium pubescens regulates osteoblasts anabolism through BMP-2, SMAD4, and Cbfa1 expression. Phytomedicine. 2010; 17:414–423.


23. Chandini SK, Ganesan P, Bhaskar N. In vitro antioxidant activities of three selected brown seaweeds of India. Food Chem. 2008; 107:707–713.


24. Bai XC, Lu D, Bai J, Zheng H, Ke ZY, Li XM, Luo SQ. Oxidative stress inhibits osteoblastic differentiation of bone cells by ERK and NF-kappaB. Biochem Biophys Res Commun. 2004; 314:197–207.


25. Neve A, Corrado A, Cantatore FP. Osteoblast physiology in normal and pathological conditions. Cell Tissue Res. 2011; 343:289–302.


26. Bellows CG, Aubin JE, Heersche JN. Initiation and progression of mineralization of bone nodules formed in vitro: the role of alkaline phosphatase and organic phosphate. Bone Miner. 1991; 14:27–40.


27. Horiguchi Y, Nakai T, Kume K. Effects of Bordetella bronchiseptica dermonecrotic toxin on the structure and function of osteoblastic clone MC3T3-E1 cells. Infect Immun. 1991; 59:1112–1116.


28. Kühn MC, Willenberg HS, Schott M, Papewalis C, Stumpf U, Flohé S, Scherbaum WA, Schinner S. Adipocyte-secreted factors increase osteoblast proliferation and the OPG/RANKL ratio to influence osteoclast formation. Mol Cell Endocrinol. 2012; 349:180–188.


29. Simonet WS, Lacey DL, Dunstan CR, Kelley M, Chang MS, Lüthy R, Nguyen HQ, Wooden S, Bennett L, Boone T, Shimamoto G, DeRose M, Elliott R, Colombero A, Tan HL, Trail G, Sullivan J, Davy E, Bucay N, Renshaw-Gegg L, Hughes TM, Hill D, Pattison W, Campbell P, Sander S, Van G, Tarpley J, Derby P, Lee R, Boyle WJ. Osteoprotegerin: a novel secreted protein involved in the regulation of bone density. Cell. 1997; 89:309–319.


30. Park KH, Kang JW, Lee EM, Kim JS, Rhee YH, Kim M, Jeong SJ, Park YG, Kim SH. Melatonin promotes osteoblastic differentiation through the BMP/ERK/Wnt signaling pathways. J Pineal Res. 2011; 51:187–194.


31. Huang W, Rudkin GH, Carlsen B, Ishida K, Ghasri P, Anvar B, Yamaguchi DT, Miller TA. Overexpression of BMP-2 modulates morphology, growth, and gene expression in osteoblastic cells. Exp Cell Res. 2002; 274:226–234.


32. Phimphilai M, Zhao Z, Boules H, Roca H, Franceschi RT. BMP signaling is required for RUNX2-dependent induction of the osteoblast phenotype. J Bone Miner Res. 2006; 21:637–646.


33. Liu ZP, Li WX, Yu B, Huang J, Sun J, Huo JS, Liu CX. Effects of trans-resveratrol from Polygonum cuspidatum on bone loss using the ovariectomized rat model. J Med Food. 2005; 8:14–19.


34. Rassi CM, Lieberherr M, Chaumaz G, Pointillart A, Cournot G. Down-regulation of osteoclast differentiation by daidzein via caspase 3. J Bone Miner Res. 2002; 17:630–638.


35. O'Gorman DM, Tierney CM, Brennan O, O'Brien FJ. The marinederived, multi-mineral formula, Aquamin, enhances mineralisation of osteoblast cells in vitro. Phytother Res. 2012; 26:375–380.