Abstract
The purpose of this study is to investigate the effects of eight weeks high fat intake and regular exercise in skeletal muscle and adipose tissue for Endoplasmic Reticulum (ER) stress in rats. This experiment involved 32 subjects (sprague-dawley rats) divided into four groups as follows: chow group (Chow, n = 8), chow and exercise group (Chow + EX, n = 8), high fat diet-induced hyperlipidemia group (HF, n = 8), and HF and exercise group (HF + EX, n = 8). As a result, there were significant decrease in body weight and abdominal fat, and blood lipid level was significantly improved by exercise for eight weeks (p < .05). There were variables changed about the skeletal muscle and ER stress in GRP78, XBP-1, ATF4, CHOP and JNK mRNA. There increased in mRNA factor by exercise, especially GRP78, and ATF4 mRNA were significantly increased in exercise (p < .05). However, there were increased in adipose tissue by exercise and there were significantly decreased in mRNA factor by high fat diet (p < .05). Consequently, this study suggests that the consistent exercise was more improved of obesity factor, such as dyslipidemia, hyperlipidemia, hyperglycemia, as well as body weight or abdominal fat. The response of ER stress in adipose tissue and skeletal muscle were more sensitive in exercise than high fat diet feed.
Figures and Tables
Fig. 1
The change of body weight during 8 weeks. Chow + EX, CON + exercise; HF, hight fat diet; HF + EX, HF + exercise.
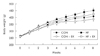
Fig. 3
ER stress gene expression in adipose tissue. a: p < 0.5, significantly different from CON, b: p < 0.5, significantly different from CON + EX, c: p < 0.5, significantly different from HF.
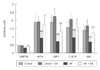
References
1. Calfon M, Zeng H, Urano F, Till JH, Hubbard SR, Harding HP, Clark SG, Ron D. IRE1 couples endoplasmic reticulum load to secretory capacity by processing the XBP-1 mRNA. Nature. 2002. 415(6867):92–96.


2. Hendershot L, Wei J, Gaut J, Melnick J, Aviel S, Argon Y. Inhibition of immunoglobulin folding and secretion by dominant negative BiP ATPase mutants. Proc Natl Acad Sci U S A. 1996. 93(11):5269–5274.


3. Verkhratsky A, Toescu EC. Endoplasmic reticulum Ca (2+) homeostasis and neuronal death. J Cell Mol Med. 2003. 7(4):351–361.
4. Oyadomari S, Koizumi A, Takeda K, Gotoh T, Akira S, Araki E, Mori M. Targeted disruption of the Chop gene delays endoplasmic reticulum stress-mediated diabetes. J Clin Invest. 2002. 109(4):525–532.


5. Mori K. Tripartite management of unfolded proteins in the endoplasmic reticulum. Cell. 2000. 101(5):451–454.


6. Hotamisligil GS. Endoplasmic reticulum stress and the inflammatory basis of metabolic disease. Cell. 2010. 140(6):900–917.


7. Gregor MF, Yang L, Fabbrini E, Mohammed BS, Eagon JC, Hotamisligil GS, Klein S. Endoplasmic reticulum stress is reduced in tissues of obese subjects after weight loss. Diabetes. 2009. 58(3):693–700.


8. Hardie DG. The AMP-activated protein kinase pathway--new players upstream and downstream. J Cell Sci. 2004. 117(Pt 23):5479–5487.


9. Irving BA, Davis CK, Brock DW, Weltman JY, Swift D, Barrett EJ, Gaesser GA, Weltman A. Effect of exercise training intensity on abdominal visceral fat and body composition. Med Sci Sports Exerc. 2008. 40(11):1863–1872.


10. Michishita R, Shono N, Kasahara T, Tsuruta T. Effects of low intensity exercise therapy on early phase insulin secretion in overweight subjects with impaired glucose tolerance and type 2 diabetes mellitus. Diabetes Res Clin Pract. 2008. 82(3):291–297.


11. Holloway GP. Mitochondrial function and dysfunction in exercise and insulin resistance. Appl Physiol Nutr Metab. 2009. 34(3):440–446.


12. Murlasits Z, Lee Y, Powers SK. Short-term exercise does not increase ER stress protein expression in cardiac muscle. Med Sci Sports Exerc. 2007. 39(9):1522–1528.


13. Kim Y, Park M, Boghossian S, York DA. Three weeks voluntary running wheel exercise increases endoplasmic reticulum stress in the brain of mice. Brain Res. 2010. 1317:13–23.


14. Chapados NA, Lavoie JM. Exercise training increases hepatic endoplasmic reticulum (er) stress protein expression in MTP-inhibited high-fat fed rats. Cell Biochem Funct. 2010. 28(3):202–210.


15. Powers SK, Criswell D, Lawler J, Martin D, Lieu FK, Ji LL, Herb RA. Rigorous exercise training increases superoxide dismutase activity in ventricular myocardium. Am J Physiol. 1993. 265(6 Pt 2):H2094–H2098.


16. Jackuliaková D, Vaverková H, Karásek D. Relationship between familial combined hyperlipidemia and insulin resistance. Vnitr Lek. 2008. 54(11):1045–1053.
17. Zanuso S, Jimenez A, Pugliese G, Corigliano G, Balducci S. Exercise for the management of type 2 diabetes: a review of the evidence. Acta Diabetol. 2010. 47(1):15–22.


18. Kirwan JP, Solomon TP, Wojta DM, Staten MA, Holloszy JO. Effects of 7 days of exercise training on insulin sensitivity and responsiveness in type 2 diabetes mellitus. Am J Physiol Endocrinol Metab. 2009. 297(1):E151–E156.


19. González B, Manso R. Induction, modification and accumulation of HSP70s in the rat liver after acute exercise: early and late responses. J Physiol. 2004. 556(Pt 2):369–385.


20. González B, Hernando R, Manso R. Stress proteins of 70 kDa in chronically exercised skeletal muscle. Pflugers Arch. 2000. 440(1):42–49.


21. Um HS, Kang EB, Leem YH, Cho IH, Yang CH, Chae KR, Hwang DY, Cho JY. Exercise training acts as a therapeutic strategy for reduction of the pathogenic phenotypes for Alzheimer’s disease in an NSE/APPsw-transgenic model. Int J Mol Med. 2008. 22(4):529–539.


22. Saito A, Ochiai K, Kondo S, Tsumagari K, Murakami T, Cavener DR, Imaizumi K. Endoplasmic reticulum stress response mediated by the PERK-eIF2 (alpha)-ATF4 pathway is involved in osteoblast differentiation induced by BMP2. J Biol Chem. 2011. 286(6):4809–4818.


23. Rzymski T, Milani M, Singleton DC, Harris AL. Role of ATF4 in regulation of autophagy and resistance to drugs and hypoxia. Cell Cycle. 2009. 8(23):3838–3847.


24. Ye J, Koumenis C. ATF4, an ER stress and hypoxia-inducible transcription factor and its potential role in hypoxia tolerance and tumorigenesis. Curr Mol Med. 2009. 9(4):411–416.


25. Siu F, Bain PJ, LeBlanc-Chaffin R, Chen H, Kilberg MS. ATF4 is a mediator of the nutrient-sensing response pathway that activates the human asparagine synthetase gene. J Biol Chem. 2002. 277(27):24120–24127.


26. Jiang HY, Wek RC. Phosphorylation of the alpha-subunit of the eukaryotic initiation factor-2 (eIF2alpha) reduces protein synthesis and enhances apoptosis in response to proteasome inhibition. J Biol Chem. 2005. 280(14):14189–14202.


27. Wu J, Ruas JL, Estall JL, Rasbach KA, Choi JH, Ye L, Boström P, Tyra HM, Crawford RW, Campbell KP, Rutkowski DT, Kaufman RJ, Spiegelman BM. The unfolded protein response mediates adaptation to exercise in skeletal muscle through a PGC-1α/ATF6α complex. Cell Metab. 2011. 13(2):160–169.


28. Deldicque L, Cani PD, Philp A, Raymackers JM, Meakin PJ, Ashford ML, Delzenne NM, Francaux M, Baar K. The unfolded protein response is activated in skeletal muscle by high-fat feeding: potential role in the downregulation of protein synthesis. Am J Physiol Endocrinol Metab. 2010. 299(5):E695–E705.


29. Deldicque L, Van Proeyen K, Francaux M, Hespel P. The unfolded protein response in human skeletal muscle is not involved in the onset of glucose tolerance impairment induced by a fat-rich diet. Eur J Appl Physiol. 2011. 111(7):1553–1558.


30. Yoshida H, Oku M, Suzuki M, Mori K. pXBP1 (U) encoded in XBP1 pre-mRNA negatively regulates unfolded protein response activator pXBP1 (S) in mammalian ER stress response. J Cell Biol. 2006. 172(4):565–575.


31. Wang H, Kouri G, Wollheim CB. ER stress and SREBP-1 activation are implicated in beta-cell glucolipotoxicity. J Cell Sci. 2005. 118(Pt 17):3905–3915.


32. Urano F, Wang X, Bertolotti A, Zhang Y, Chung P, Harding HP, Ron D. Coupling of stress in the ER to activation of JNK protein kinases by transmembrane protein kinase IRE1. Science. 2000. 287(5453):664–666.


33. Sun W, Bi Y, Liang H, Cai M, Chen X, Zhu Y, Li M, Xu F, Yu Q, He X, Ye J, Weng J. Inhibition of obesity-induced hepatic ER stress by early insulin therapy in obese diabetic rats. Endocrine. 2011. 39(3):235–241.

