Abstract
Background
Carbon monoxide (CO) is a cytoprotective and homeostatic molecule with important signaling capabilities in physiological and pathophysiological situations. CO protects cells/tissues from damage by free radicals or oxidative stress. NAD(P)H:quinone oxidoreductase (NQO1) is a highly inducible enzyme that is regulated by the Kelch-like ECH-associated protein 1 (Keap1)/nuclear factor erythroid 2-related factor 2 (Nrf2)/antioxidant response element (ARE) pathway, which is central to efficient detoxification of reactive metabolites and reactive oxygen species (ROS).
Methods
We generated NQO1 promoter construct. HepG2 cells were treated with CO Releasing Molecules-2 (CORM-2) or CO gas and the gene expressions were measured by RT-PCR, immunoblot, and luciferase assays.
Results
CO induced expression of NQO1 in human hepatocarcinoma cell lines by activation of Nrf2. Exposure of HepG2 cells to CO resulted in significant induction of NQO1 in dose- and time-dependent manners. Analysis of the NQO1 promoter indicated that an antioxidant responsible element (ARE)-containing region was critical for the CO-induced Nrf2-dependent increase of NQO1 gene expression in HepG2 cells.
Carbon monoxide (CO) is a reaction product of the cytoprotective heme oxygenase (HO-1) (1). Endogenous HO-1 has the potential to generate CO, bilirubin and iron (Fe++) at the time of heme breakdown. CO has beneficial effects on many patho-physiological conditions, which mimics the role of HO-1 (2,3). Recently, CO has emerged as a potential therapeutic agent for the treatment of various cardiovascular disorders (2-4). There is an abundance of preclinical evidence in large and small animals showing the beneficial effects of CO, administered as a gas or as a CO Releasing Molecules (CORM), on cardiovascular disease, sepsis and shock, cancer, acute and chronic rejection of a transplanted organ, and acute lung, kidney and liver injuries (5).
NAD(P)H:quinone oxidoreductase (NQO1), a cytosolic flavoprotein that catalyzes quinine detoxification, is induced in response to various agents at the transcriptional level. The NQO1 enzyme is implicated in protection against oxidative stress (6,7) and carcinogenesis, including stabilization of the p53 tumor suppressor (8-10). NQO1-deficient mice show reduced p53 induction and apoptosis, increased susceptibility to chemically induced tumors (11) and impaired NF-κB function (12). The NQO1 gene promoter region contains several essential cis-elements including xenobiotic response element (XRE) and antioxidant response element (ARE) (13). Induction of NQO1 expression by various stimuli has been reported to be regulated by ARE (14-16), which contains several receptors for several nuclear transcription factors such as Nrf2.
The transcription factor Nrf2 (nuclear factor erythroid 2-related factor 2) is a key oxidative stress response modifier that induces transcription of a variety of genes through binding to the antioxidant response element (ARE) in target gene promoters (17-19). Nrf2-dependent activation of ARE-driven gene promoters is generally understood to lead to induction of cytoprotective proteins, which enable cells to combat oxidative insult (18,19).
In unstressed cells, Nrf2 activity is thought to be repressed by Keap1 (Kelch-like ECH-associated protein 1) (20,21), a cytoskeleton-associated protein. Ubiquitin-mediated degradation of Nrf2 is induced by its binding to Keap1. In one model for the activation of Nrf2 in stressed cells, electrophile- or reactive oxygen species-induced release of Nrf2 is proposed to involve covalent modifications of Keap1 and/or Nrf2 in the cytoplasm. In addition, microarray results suggest that more than 200 gene products including an antioxidative enzyme NQO1 are under the transcriptional control for Nrf2 (17).
In this study, we demonstrate that CO induces NQO1 gene expression through the Nrf2-dependent pathway.
The human hepatocarcinoma cell lines (HepG2) were cultured in DMEM (Gibco, NY). All media was supplemented with 10% fetal bovine serum and a 100 units/ml penicillin-streptomycin mixture (Gibco, NY). Cell lines were incubated at 37℃ with 100% humidity in 5% CO2 and passaged using standard cell culture techniques. Ticharbonyl dichlororuthenium (II) dimer (CORM-2) was from Sigma-Aldrich (St. Louis, MO).
The sequence of the human NQO1 genomic locus at 16q22.1 (GenBank accession number NM_000016.9) was used to engineer PCR cloning primers. A 618 base pair (bp) genomic fragment containing the 5'-flanking region of the NQO1 gene was isolated by PCR amplification from human genomic DNA. Construct pGL3/NQO1p-501 contains the -501 bp promoter region of the human NQO1 gene up to nucleotide+ 117 bp (i.e., downstream from the NQO1 mRNA capsite) inserted into the SacI and XhoI sites of the pGL3 basic vector (Promega). Oligonucleotides for construct pGL3/NOQ1p-ARE were synthesized at Bioneer Corporation. The oligonucleotides were ligated into the SacI and XhoI sites of the pGL3 basic vector. Nucleotide sequences of the primers were as follows: pGL3/NQO1p-501: 5'-GAGCTCGTGCAGAATCTGAATCTTGCAAAG-3' (sense) and 5'-CTCGAGGCTCTGGTGCAGTCCGGGGCGCT-3' (antisense). pGL3/NQO1p-ARE: 5'-CCTCAGCCTTCCAAATCCGCAGTCACAGTGACTCAGCAGAA TCC-3' (sense), TCGAGGATTCTGCTGAGTCACTGTGACTGCGGATTTGGAAGGCTGAGGAGCT-3' (antisense). The mammalian expression plasmid pcDNA3 bearing human Nrf2 was obtained from Addgene (Cambridge, MA).
Small interfering RNAs (siRNAs) against human Nrf2 (Nrf2-siRNA) (sc37030), human PERK (PERK-siRNA) (sc-36213) and control siRNA (scRNA) (sc-37007) were purchased from Santa Cruz Biotechnology (Santa Cruz, CA).
HepG2 cells were plated in 24-well flat bottom plates on a day prior to transfection. Cells were then co-transfected with pGL3/NQO1p-501-luciferase reporter construct, pcDNA3-mycNrf2 and pRL-SV40 Renilla luciferase construct using Lipofectamine reagent (Invitrogen, CA). Forty eight hours after transfection, cells were lysed, and both firefly and Renilla luciferase activities were measured with Dual-Luciferase reporter assay system (Promega, WI), according to the manufacturer's instructions. The luciferase activities were measured on a SpectraMax L (M. Devices). Each experiment was carried out at least 3 times with triplicate samples.
HepG2 cells were treated with the indicated concentration of CORM-2. At the indicated time after CORM-2 treatment, total RNA was prepared using Trizol reagent (Invitrogen, CA). Two microgram of total RNA was used to synthesize the first-strand cDNA by using oligo-dT primers (QIAGEN, CA) and M-MLV reverse transcriptase (Promega, WI) according to the manufacturer's instructions. The synthesized cDNA was subjected to the PCR-based amplification. Oligonucleotide primer sets used were as follows: NQO1, 5'-GTATCCTGCCGAGTCTGTT-3' (sense) and 5'-GATCCCTTGCAGAGAGTACA-3' (antisense); HO-1, 5'-GGAACTTTCAGAAGGGCCAG-3' (sense) and 5'-GTCCTTGGTGTCATGGGTCA-3' (antisense); GAPDH, 5'-CCACCCATGGCAAATTCCATGGCA-3' (sense) and 5'-TCTAGACGGCAGGTCAGGTCCACC-3' (antisense). The expression of GAPDH was measured as an internal control. The PCR products were subjected to agarose gel electorphoresis and visualized by ethidium bromide staining.
For immunoblot, cells were lysed in a lysis buffer containing 25 mM Tris-HCl, pH 7.5, 137 mM NaCl, 2.7 mM KCl, 1% Triton X-100, and Halt™ Protease and Phosphatase inhibitor cocktail (Thermo Scientific, IL), and spun to separate insoluble debris from the clear lysates. Equal amounts of cell lysates were separated by SDS-PAGE and transferred onto Immobilon-P membranes (Millipore, Bedford, MA). The transferred membranes were incubated with anti-NQO1 (Cell Signaling, DE), polyclonal anti-HO-1 (Enzo Life Sciences, NY), anti-actin (Santa Cruz Biotechnology, CA) antibody. Immunoreactivity was detected using the ECL detection system (GE Healthcare Bio-Sciences Corp. NJ).
CO, a reaction product of HO-1 activity, has been shown to have potent anti-inflammatory, anti-proliferative, and anti-apoptotic effects. In our previous study, we revealed that CO induces Nrf2 activation and HO-1 expression in the liver cells (22). Furthermore, activated Nrf2 might induce various proteins which play a role of cytoprotection. Because NQO1 as one of the most cytoprotective molecules which is induced by Nrf2 in various stress conditions, we examined whether CO could increase NQO1 gene expression. As shown in Fig. 1A, NQO1 expression was increased by CO in a dose-dependent manner up to 50 µM of CORM-2. To confirm the effect of metal carrier of CORM-2, cells were treated with RuCl2 in a dose dependent manner. However, treatment of cells with equivalent molar concentrations of ruthenium chloride (RuCl2) did not induce NQO1 expression (Fig. 1B). As shown in Fig. 2A, we also found that NQO1 expression was increased in a time-dependent manner. Compared with un-stimulated cells, an increase in NQO1 expression was observed in cells exposed to CO for 6 h. We also found that NQO1 was induced by CO gas at 6 h (Fig. 2B). NQO1 protein expression, as well as NQO1 mRNA expression, was also increased by CORM-2 in a dose dependent manner (Fig. 3). We report for the first time that exogenous CO induces NQO1 expression in HepG2 cells.
Our previous studies showed that CO induces Nrf2 activation (22). Moreover, it has been reported that the importance of transcription factor Nrf2 in regulating ARE-dependent the transcription of ARE-dependent NQO1 gene (23). We confirmed that NQO1 expression was increased by CO at RNA levels in a dose-dependent manner (Fig. 1 and 2). To confirm whether the expression of NQO1 mRNA induced by CORM-2 was due to transcriptional regulation, we performed a promoter activity assay on the NQO1 gene. First, we constructed the NQO1 promoter-luc plasmid (pGL3/NQO1p-501) by subcloning NQO1 promoter region into the pGL3 basic vector for luciferase assay. After transfecting the indicated promoter constructs, cells were treated with CORM-2 for 6 h and luciferase activity was measured. As expected, treatment of CORM with pGL3/NQO1p-501 transfected cells resulted in a 1.4 fold increase in NQO1 promoter activity when compared to pGL3/NQO1p-501 transfected cells (Fig. 4). These results indicate that CORM-2 also induces NQO1 promoter activity at the transcriptional level.
Nrf2 is a major transcription factor involved in cellular protection against oxidative stress through ARE-mediated induction of several phase II detoxifying and antioxidant enzymes (24). It is known that Nrf2 regulates the antioxidant response element-mediated expression of NQO1 gene (25). To further evaluate the involvement of Nrf2 in CO-induced NQO1 expression, we constructed the plasmid containing antioxidant response element (pGL3/NQO1p-ARE) within the NQO1 promoter that might be involved in Nrf2-mediated induction of the gene. Co-transfection studies were performed using Nrf2 expression plasmids and the pGL3/NQO1p-ARE luciferase promoter construct. As shown in Fig. 5, Nrf2-overexpression increased pGL3/NQO1p-ARE promoter activity by ~5-fold compared with pcDNA3 mock vector transfected cells. In addition, transfection of Nrf2 followed by CO treatment resulted in ~1.4 fold increase in the induction of pGL3/NQO1p-ARE promoter activity. Based on these results, we suggest that Nrf2-mediated ARE activation is involved in CORM-2-induced NQO1 gene expression.
Cobalt protoporphyrin (CoPP) is a well-known inducer of HO-1 in many cell types. Curcumin also activates Nrf-2/HO-1 pathway (26). Because endogenous HO-1 has the potential to generate CO, we wanted to know whether CoPP or curcumin also induce NQO1 expression through CO generated by HO-1. After treatment with CoPP or curcumin, we analyzed HO-1 expression by semi-quantitative RT-PCR at different times. HO-1 expression was induced by CoPP or curcumin in a time-dependent manner (Fig. 6). In a similar fashion, CoPP or curcumin also caused a significant increase in NQO1 expression (Fig. 6). Collectively, these data support that expression of NQO1 is induced by CoPP or curcumin via HO-1 induction.
As shown in Fig. 4, CORM-2 treatment of HepG2 cells was able to significantly stimulate the NQO1 promoter at 6 h after the incubation. To confirm the involvement of Nrf2 for CO-induced NQO1 promoter activity, we inhibited Nrf2 expression by siRNA against Nrf2. Down-regulation of Nrf2 by siRNA abrogated the CO-induced NQO1 promoter activity (Fig. 7). These data indicate that the NQO1 promoter activity is under regulation of CO and Nrf2 in HepG2 cells. In addition, our previous result showed that CO up-regulates Nrf2-dependent HO-1 expression via PERK activation (27). Similar to the reduction of NQO1 promoter activity by siRNA against Nrf2, CO-induced NQO1 promoter activities were abrogated by PERK siRNA suggesting that induction of NQO1 promoter activity by CORM-2 is mediated via a PERK pathway. Thus, our data suggest that NQO1 expression is induced by CO through PERK-Nrf2 pathway.
CO has shown the impact as a potential therapeutic molecule. There is an abundance of preclinical evidence in animal models of cardiovascular disease, inflammatory disorders and organ transplantation showing the beneficial effects of CO, administered as a gas or as a CORM-2 (28). NQO1 is highly inducible, and its induction protected animals and their cells against the toxic and oxidative stress. Here we show for the first time that CO can induce NQO1 gene transcription in Nrf2-dependent pathway, corroborating our previous report (22) that CO can up-regulate Nrf2-mediated HO-1 expression in liver cells. Nrf2 is responsible for mediating binding to the antioxidant responsible element (ARE) (20,29). AREs containing 12-O-tetradecanoylphorbol-13-acetate response element (TRE) (perfect AP1) elements has been reported to mediate high basal transcription of the human NQO1 and its induction in response to various stimuli such as polyphenol, xenobiotics, and oxidative stress (30,31).
In this study, we hypothesized that CO, a reaction product of the cytoprotective heme oxygenase (HO)-1, up-regulates the inducible NQO1 gene expression by enhancing the Nrf2 signaling pathway. To address this hypothesis, we first confirmed that CO induced NQO1 mRNA expression (Fig. 1). And also, CORM-2 was capable of causing a significant induction of NQO1 at the mRNA and protein levels in HepG2 cells in dose-dependent manner.
Furthermore, we cloned a 618-bp NQO1 promoter (pGL3/NQO1p-501) that ranges from -501 to +117 relative to the transcriptional start site. When the cells were treated with only CORM-2 or CO gas, NQO1 gene expression and its promoter activity were significantly induced (Fig. 2 and 4). It is possible that CO-mediated transcription factor can bind to this region of NQO1 promoter. It has been shown that NQO1 promoter contains antioxidant response element (ARE), a basal element, and AP-2 element (32). Because ARE is essentially required for expression and coordinated induction of NQO1, we measured promoter activity using pGL3/NQO1p-ARE construct containing only ARE. Additionally, it was reported that ARE-dependent transcriptional activation of the NQO1 gene requires the Nrf2 activation, which could consequently bind to ARE and subsequently activated NQO1 transcription (33,34). As shown in Fig. 5, overexpression of Nrf2 resulted in an induction of pGL3/NQO1p-ARE promoter activation and Nrf2-mediated NQO1 transcriptional induction was significantly enhanced by CO. In addition, knockdown of Nrf2 and PERK resulted in a reduction of NQO1 promoter activity. These results indicate a possibility that NQO1 expression is induced by CO activated PERK-Nrf2 axis.
CoPP is well-known to induce HO-1. Curcumin was also reported to increase HO-1 expression via Nrf2 to protect oxidative stress (26). We demonstrated that HO-1 induced by CoPP or curcumin would significantly increase the expression of NQO1. These studies show a possibility that NQO1 expression is induced by endogenous CO generated by CoPP- or curcumin-induced HO-1.
Several studies reported that NQO1 has various physiological functions. For example, NQO1 affects the TNF-mediated pathway to apoptosis (35). Furthermore, ethanol-mediated up-regulation of HO-1 and NQO1 in THP-1 cells led to significant decrease of inflammatory cytokines production, such as TNF-α and IL-1β (36). NQO1 has protective effect against the development of Alzheimer's disease (37) as well as binds to p53 and inhibits p53 degradation in an ubiquitin-independent pathway via the 20S proteasome (11). Especially, CO gas and CORM exhibit a wide range of biological effects that encompass inflammation, apoptosis and cellular proliferation (38-40). Therefore, we have investigated the effects of CO on the various physiological functions of NQO1.
Consequently, we found that CO-induced Nrf2 activation leads to up-regulation of NQO1. Because CO has a beneficial effect in animal model of disease, regulation of CO-mediated NQO1 expression through Nrf2 activation may be beneficial in preventing disease caused by various stimuli.
Figures and Tables
Figure 1
Dose-dependent NQO1 mRNA induction by exogenous CO. (A, B) HepG2 cells were treated with various concentrations of CORM-2 as exogenous CO donor or Rucl2 as control for 6 h and the cells were harvested for semi-quantitative RT-PCR. The mRNA expression of NQO1 was determined by semi-quantitative RT-PCR. GAPDH was used as internal controls.
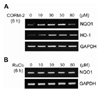
Figure 2
Time dependent NQO1 mRNA induction by exogenous CO. (A, B) HepG2 cells were treated with 50 uM CORM-2 or 250 ppm CO gas for indicated time points and analyzed. The mRNA expression of NQO1 was determined by semi-quantitative RT-PCR as described under Material and methods. GAPDH was used as internal controls.
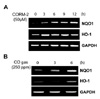
Figure 3
Effects of exogenous CO on the NQO1 and HO-1 protein induction. HepG2 cells were treated with various concentrations of CORM-2 as exogenous CO donor for 6 h and the cell lysates were used for immunoblot with antibody against NQO1, HO-1, and β-actin.
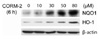
Figure 4
Effects of CORM-2 on the NQO1 promoter activity. HepG2 cells were transfected with hNQO1p-501 containing the NQO1 promoter (-501 to +117). After 24 h cells were treated with various concentrations of CORM-2. At 6 h post-treatment, the level of firefly luciferase activity was normalized to the Renilla luciferase activity. The relative luciferase activities are presented as a fold increase over no-treated cells. Each bar represents the mean±S.D. of three independent experiments (**p<0.01).
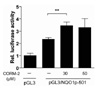
Figure 5
Effects of CORM-2 on Nrf2-mediated NQO1 promoter activity. HepG2 cells were co-transfected with pGL3/NQO1p-ARE and pcDNA3-Nrf2. After 24 h, cells were treated with 30 uM CORM-2 and at 6 h post-treatment, luciferase activity was determined. The levels of firefly luciferase activity were normalized to the Renilla luciferase activity. The relative luciferase activities are presented as a fold increase over no-treated cells. Each bar represents the mean±S.D. of three independent experiments (*p<0.05, ***p<0.001).
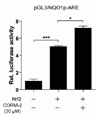
Figure 6
Effects of CoPP and curcumin on NQO1 expression. (A, B) HepG2 cells were treated with 10 µM CoPP and 10 µM curcumin for the indicated time period. Total RNA were extracted and expression of NQO1 and GAPDH mRNA was detected by semiquantitative RT-PCR.
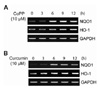
Figure 7
Effects of silencing of Nrf2 on CO-induced NQO1 promoter activity HepG2 cells were transfected with pGL3/NQO1p-501 containing the NQO1 promoter (-501 to +117), Nrf2 specific (siNRf2), and PERK specific (siPERK) or scrambled siRNA (scRNA). After 24 h cells were treated with various concentrations of CORM-2. At 6 h post-treatment, the level of firefly luciferase activity was normalized to the Renilla luciferase activity. The relative luciferase activities are presented as a fold increase over no-treated cells. Each bar represents the mean±S.D. of three independent experiments (*p<0.05, **p<0.01).
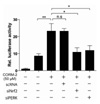
ACKNOWLEDGEMENTS
This work is supported by Grant from Korea Research Foundation Grant funded by the Korean Government (MOEHRD) (BRL 2010-0001199).
References
1. Tenhunen R, Marver HS, Schmid R. The enzymatic conversion of heme to bilirubin by microsomal heme oxygenase. Proc Natl Acad Sci U S A. 1968. 61:748–755.


2. Otterbein LE, Soares MP, Yamashita K, Bach FH. Heme oxygenase-1: unleashing the protective properties of heme. Trends Immunol. 2003. 24:449–455.


3. Otterbein LE, Zuckerbraun BS, Haga M, Liu F, Song R, Usheva A, Stachulak C, Bodyak N, Smith RN, Csizmadia E, Tyagi S, Akamatsu Y, Flavell RJ, Billiar TR, Tzeng E, Bach FH, Choi AM, Soares MP. Carbon monoxide suppresses arteriosclerotic lesions associated with chronic graft rejection and with balloon injury. Nat Med. 2003. 9:183–190.


4. Fujimoto H, Ohno M, Ayabe S, Kobayashi H, Ishizaka N, Kimura H, Yoshida K, Nagai R. Carbon monoxide protects against cardiac ischemia--reperfusion injury in vivo via MAPK and Akt--eNOS pathways. Arterioscler Thromb Vasc Biol. 2004. 24:1848–1853.


5. Bauer I, Pannen BH. Bench-to-bedside review: Carbon monoxide--from mitochondrial poisoning to therapeutic use. Crit Care. 2009. 13:220.


6. Siegel D, Gustafson DL, Dehn DL, Han JY, Boonchoong P, Berliner LJ, Ross D. NAD(P)H:quinone oxidoreductase 1: role as a superoxide scavenger. Mol Pharmacol. 2004. 65:1238–1247.


7. Siegel D, Bolton EM, Burr JA, Liebler DC, Ross D. The reduction of alpha-tocopherolquinone by human NAD(P)H: quinone oxidoreductase: the role of alpha-tocopherolhydroquinone as a cellular antioxidant. Mol Pharmacol. 1997. 52:300–305.


8. Asher G, Lotem J, Cohen B, Sachs L, Shaul Y. Regulation of p53 stability and p53-dependent apoptosis by NADH quinone oxidoreductase 1. Proc Natl Acad Sci U S A. 2001. 98:1188–1193.


9. Asher G, Lotem J, Kama R, Sachs L, Shaul Y. NQO1 stabilizes p53 through a distinct pathway. Proc Natl Acad Sci U S A. 2002. 99:3099–3104.


10. Asher G, Lotem J, Sachs L, Kahana C, Shaul Y. Mdm-2 and ubiquitin-independent p53 proteasomal degradation regulated by NQO1. Proc Natl Acad Sci U S A. 2002. 99:13125–13130.


11. Iskander K, Gaikwad A, Paquet M, Long DJ 2nd, Brayton C, Barrios R, Jaiswal AK. Lower induction of p53 and decreased apoptosis in NQO1-null mice lead to increased sensitivity to chemical-induced skin carcinogenesis. Cancer Res. 2005. 65:2054–2058.


12. Ahn KS, Sethi G, Jain AK, Jaiswal AK, Aggarwal BB. Genetic deletion of NAD(P)H:quinone oxidoreductase 1 abrogates activation of nuclear factor-kappaB, IkappaBalpha kinase, c-Jun N-terminal kinase, Akt, p38, and p44/42 mitogen-activated protein kinases and potentiates apoptosis. J Biol Chem. 2006. 281:19798–19808.


14. Dhakshinamoorthy S, Jaiswal AK. Functional characterization and role of INrf2 in antioxidant response element-mediated expression and antioxidant induction of NAD(P)H:quinone oxidoreductase1 gene. Oncogene. 2001. 20:3906–3917.


15. Nioi P, Hayes JD. Contribution of NAD(P)H:quinone oxidoreductase 1 to protection against carcinogenesis, and regulation of its gene by the Nrf2 basic-region leucine zipper and the arylhydrocarbon receptor basic helix-loop-helix transcription factors. Mutat Res. 2004. 555:149–171.


16. Jaiswal AK. Nrf2 signaling in coordinated activation of antioxidant gene expression. Free Radic Biol Med. 2004. 36:1199–1207.


17. Kwak MK, Wakabayashi N, Itoh K, Motohashi H, Yamamoto M, Kensler TW. Modulation of gene expression by cancer chemopreventive dithiolethiones through the Keap1-Nrf2 pathway. Identification of novel gene clusters for cell survival. J Biol Chem. 2003. 278:8135–8145.


18. Nguyen T, Sherratt PJ, Pickett CB. Regulatory mechanisms controlling gene expression mediated by the antioxidant response element. Annu Rev Pharmacol Toxicol. 2003. 43:233–260.


19. Motohashi H, Yamamoto M. Nrf2-Keap1 defines a physiologically important stress response mechanism. Trends Mol Med. 2004. 10:549–557.


20. Itoh K, Wakabayashi N, Katoh Y, Ishii T, Igarashi K, Engel JD, Yamamoto M. Keap1 represses nuclear activation of antioxidant responsive elements by Nrf2 through binding to the amino-terminal Neh2 domain. Genes Dev. 1999. 13:76–86.


21. Kang MI, Kobayashi A, Wakabayashi N, Kim SG, Yamamoto M. Scaffolding of Keap1 to the actin cytoskeleton controls the function of Nrf2 as key regulator of cytoprotective phase 2 genes. Proc Natl Acad Sci U S A. 2004. 101:2046–2051.


22. Lee BS, Heo J, Kim YM, Shim SM, Pae HO, Kim YM, Chung HT. Carbon monoxide mediates heme oxygenase 1 induction via Nrf2 activation in hepatoma cells. Biochem Biophys Res Commun. 2006. 343:965–972.


23. Rushworth SA, MacEwan DJ, O'Connell MA. Lipopolysaccharide-induced expression of NAD(P)H:quinone oxidoreductase 1 and heme oxygenase-1 protects against excessive inflammatory responses in human monocytes. J Immunol. 2008. 181:6730–6737.


24. Cho HY, Reddy SP, Debiase A, Yamamoto M, Kleeberger SR. Gene expression profiling of NRF2-mediated protection against oxidative injury. Free Radic Biol Med. 2005. 38:325–343.


25. Venugopal R, Jaiswal AK. Nrf1 and Nrf2 positively and c-Fos and Fra1 negatively regulate the human antioxidant response element-mediated expression of NAD(P)H:quinone oxidoreductase1 gene. Proc Natl Acad Sci U S A. 1996. 93:14960–14965.


26. Balogun E, Hoque M, Gong P, Killeen E, Green CJ, Foresti R, Alam J, Motterlini R. Curcumin activates the haem oxygenase-1 gene via regulation of Nrf2 and the antioxidant-responsive element. Biochem J. 2003. 371:887–895.


27. Kim KM, Pae HO, Zheng M, Park R, Kim YM, Chung HT. Carbon monoxide induces heme oxygenase-1 via activation of protein kinase R-like endoplasmic reticulum kinase and inhibits endothelial cell apoptosis triggered by endoplasmic reticulum stress. Circ Res. 2007. 101:919–927.


28. Motterlini R, Otterbein LE. The therapeutic potential of carbon monoxide. Nat Rev Drug Discov. 2010. 9:728–743.


29. Motohashi H, Yamamoto M. Nrf2-Keap1 defines a physiologically important stress response mechanism. Trends Mol Med. 2004. 10:549–557.


30. Tanigawa S, Fujii M, Hou DX. Action of Nrf2 and Keap1 in ARE-mediated NQO1 expression by quercetin. Free Radic Biol Med. 2007. 42:1690–1703.


31. Xie T, Belinsky M, Xu Y, Jaiswal AK. ARE- and TRE-mediated regulation of gene expression. Response to xenobiotics and antioxidants. J Biol Chem. 1995. 270:6894–6900.
32. Li Y, Jaiswal AK. Regulation of human NAD(P)H:quinone oxidoreductase gene. Role of AP1 binding site contained within human antioxidant response element. J Biol Chem. 1992. 267:15097–15104.


33. Miao W, Hu L, Scrivens PJ, Batist G. Transcriptional regulation of NF-E2 p45-related factor (NRF2) expression by the aryl hydrocarbon receptor-xenobiotic response element signaling pathway: direct cross-talk between phase I and II drug-metabolizing enzymes. J Biol Chem. 2005. 280:20340–20348.


34. Itoh K, Chiba T, Takahashi S, Ishii T, Igarashi K, Katoh Y, Oyake T, Hayashi N, Satoh K, Hatayama I, Yamamoto M, Nabeshima Y. An Nrf2/small Maf heterodimer mediates the induction of phase II detoxifying enzyme genes through antioxidant response elements. Biochem Biophys Res Commun. 1997. 236:313–322.


35. Siemankowski LM, Morreale J, Butts BD, Briehl MM. Increased tumor necrosis factor-alpha sensitivity of MCF-7 cells transfected with NAD(P)H:quinone reductase. Cancer Res. 2000. 60:3638–3644.
36. Yeligar SM, Machida K, Kalra VK. Ethanol-induced HO-1 and NQO1 are differentially regulated by HIF-1alpha and Nrf2 to attenuate inflammatory cytokine expression. J Biol Chem. 2010. 285:35359–35373.


37. Bian JT, Zhao HL, Zhang ZX, Bi XH, Zhang JW. Association of NAD(P)H:quinone oxidoreductase 1 polymorphism and Alzheimer's disease in Chinese. J Mol Neurosci. 2008. 34:235–240.


38. Ryter SW, Otterbein LE, Morse D, Choi AM. Heme oxygenase/carbon monoxide signaling pathways: regulation and functional significance. Mol Cell Biochem. 2002. 234-235:249–263.

