Abstract
The objective of this study was to investigate the effects of oxytocin infusion on corpus luteum (CL) function during early to mid-diestrus by measuring luteal size (LS) and luteal blood flow (LBF) along with plasma levels of progesterone (P4) and prostaglandin metabolites (13,14-dihydro-15-keto-prostaglandin F2α, PGFM). On day (D) 7 of the estrus cycle (D1 = ovulation), seven cows received 100 IU of oxytocin (OXY) or placebo (PL) following a Latin square design. LS and LBF increased in both groups over time and no differences were observed between the groups. PGFM did not differ either within the groups over time or between the groups at any time point. P4 of the OXY group was higher compared to that of the the PL group 360 min after the infusion (p = 0.01) and tended to be higher at the time points 450 min, 48 h, and 72 h (all p = 0.08). Results from this study support the hypothesis that OXY is not directly involved in the mechanism(s) governing blood flow of the CL and has no remarkable effects either on luteal size or P4 and PGFM plasma levels. Further investigation is needed to elucidate the role of OXY in CL blood flow during early and late luteal phases.
Recently, transrectal color Doppler sonography has been reported to be a very useful tool for measuring local blood flow in the female bovine reproductive tract [16]. This technique allows estimation of the corpus luteum (CL) physiological status since blood flow on the CL parenchyma reflects its structural and functional development. Moreover, Acosta and Miyamoto [2] hypothesized that in the preovulatory follicle, in the developing and in mature CL in cattle, structural and functional changes of the vasoactive system occur. This highlights the importance of blood flow in the lifespan of ovarian structures [2]. Luteal blood flow (LBF) gradually increases after ovulation along with increases in CL volume and plasma P4 concentrations from days (D) 2 to D5 of the estrus cycle [1]. LBF doubles during the static phase (D8~16) and decreases rapidly during luteal regression (D17~21) [8]. Thus, LBF values may be reliable predictors of luteal status.
The CL is one of the few adult tissues that exhibit regular periods of growth (CL formation), hormonal excretion, and luteolysis (CL regression) [3]. CL growth is predominantly regulated by angiogenic and luteotropic factors such as growth factors (i.e., insulin-like growth factors-1 and -2), oxytocin (OXY), and LH [17,22]. Regression is initiated by luteolytic PGF2α secreted by the endometrium [13] and is promoted by a complex and not well-elucidated cascade of mediators that include OXY. Nevertheless, the role of OXY in luteolysis has been previously studied. It appears that OXY is not a main factor in the initiation of luteolysis in cattle [26] although it can play a supporting and modulating role in early stages of luteolysis, as postulated in sheep [24] and cattle [25]. On the other hand, OXY has a stimulatory effect on progesterone (P4) secretion which is highest on D5~7 of the estrus cycle and declines from D8 to D12 [26]. However, OXY no longer has any stimulatory effect on P4 secretion on D15~18 [26]. Therefore, it was suggested that luteal oxytocin affects the luteal function as a luteotropic or luteolytic autocrine/paracrine factor within the CL depending on the day of the luteal phase. Nevertheless, whether this effect involves changes in LBF has never been determined. The purpose of this study was to investigate the effect of OXY administration on CL function during early to mid-diestrus by measuring luteal size (LS) and blood flow as well as peripheral plasma levels of P4.
This experiment was conducted at the Clinic for Cattle of the University of Veterinary Medicine, Hanover (Germany) in seven non-lactating multiparous Holstein-Friesian cows. The study was approved and conducted in accordance with the German legislation on animal welfare (Lower Saxony Federal State Office for Consumer Protection and Food Safety, Hannover, Germany). The animals were housed in tied stall barns and had ad libitum access to hay and water. They were healthy and examined for any reproductive tract abnormalities before enrollment in the experiment. Afterwards, all cycling cows underwent an ovulation-synchronization (Ovsynch) protocol [19] in which they received a gonadotropin-releasing hormone (GnRH) (2.5 mL; 10 µg, intramuscularly (im); Receptal; Intervet, USA), prostaglandin F2α (PGF2α, 2 mL; 0.526 mg, im; Estrumate; Intervet, USA) 7 days later, and then GnRH (10 µg, im) 48 h after PGF2α administration. At the time of the second GnRH administration, the size of the ovulatory follicle was measured with B-Mode sonography (8 MHz; General Electric, USA). The time of ovulation (D1) was determined by the disappearance of the ovulatory follicle between two consequent examinations performed at 24, 28, and 36 h after the last GnRH injection.
On D7, the cows randomly received OXY (100 IU, 10 mL, Oxykel; Agroscience, Germany) or placebo (PL; 10 mL of 0.9% sodium chloride) diluted in 1,000 mL of 0.9% sodium chloride solution. Infusion via catheter in the jugular vein lasted 60 ± 3 min. Before infusion, the ovaries were evaluated by transrectal sonography to determine that the CL had no cystic cavity. On D10, a second Ovsynch protocol was initiated in all cows with the animals assigned into the different OXY and PL groups receiving the exact reversed treatment protocol, according to a Latin square model.
Size and blood flow of the CL were examined with B-mode and Power Doppler sonography (10 MHz; GE Healthcare, USA), respectively, just prior to and 0.5, 1, 1.5, 2, 3, 5, 12, 24, 36, 48, 60, and 72 h after infusion commencement. LBF was determined by the relative blood flow area (= maximum blood flow area near the maximum cross sectional area of the CL/maximum cross sectional area of the CL). The number of pixels with color was determined as a semiquantitative assessment of LBF using computer assisted image analysis software (PixelFlux; Chameleon Software, Germany). Three images each showing the maximum blood flow area and maximum cross sectional area of the CL were captured. The average from these three images were used for further analyses. Criteria for image selection included ones showing structures of interest that lacked artifacts, had constant color intensity and had intact borders with blood flow.
Blood samples were collected via indwelling jugular catheters just before OXY infusion and every 15 min for two hours after commencing infusion. After this period, blood was collected every 30 min for another 6 h and just after the sonographic examinations. All blood samples were collected in evacuated tubes containing ethylenediaminetetraacetic acid as an anticoagulant (S-Monovette; Sarstedt, Germany). The samples were stored on ice until they were centrifuged (3,500 × g for 15 min at 4℃) within 30 min after collection, and the plasma was stored at -20℃ until hormone analyses. Progesterone and prostaglandin metabolites (13,14-dihydro-15-keto-prostaglandin F2α, PGFM) were measured by an enzyme immunoassay according to Prakash et al. [18] and Mishra et al. [14] (monoclonal anti-P4 antibody, P-1922; Sigma, USA; polyclonal anti-PGFM antibody, provided by Professor Dr. H.H.D. Meyer, Institute for Physiology, Technical University of Munich, Germany; enzymes: 4-pregnen-3, 20-dione-3-O-carboxymethyloxime-horseradish peroxidase and PGFM-horseraddish peroxidase). The intra- and interassay coefficients of variation for P4 were both less than 10%; for PGFM, these coefficients were less than 7% and less than 10%, respectively.
Statistical analyses were conducted using the statistical analysis system (ver. 9.1; SAS, USA). A Shapiro-Wilk test was performed for all outcome variables to determine the underlying distribution of the data. All variables showed a normal distribution. A repeated measures ANOVA using a general linear model within subject effects across time was conducted using proc glm (SAS, USA). Student's t-test was used to evaluate differences within animals at a specific time point using proc univariate (SAS, USA). p-values < 0.05 were considered statistically significant. Data are presented as the mean ± SE.
The luteal size did not differ between the two groups at any time point (Fig. 1). However, the size of the CL increased significantly over time in both groups (p = 0.02 and p = 0.001 for the PL and OXY groups, respectively). Additionally, LBF did not differ between the two groups over time (Fig. 2). Although the LBF increased from 0.3~0.4 cm2 to 0.7 cm2 in both groups, this difference was not significant (Fig. 2). Plasma PGFM was not significantly different within the groups over time or between the groups at any time point (Fig. 3). However, it should be mentioned that the animals in the OXY group showed greater variability compared to the PL group as demonstrated by the substantially larger SE for the OXY group (Fig. 3). OXY infusion had a weak effect on the levels of P4 (Fig. 4). Specifically, the P4 values tended to increase over time in the OXY group (p = 0.07) whereas there was no significant change in the PL group (p = 0.14). Moreover, the P4 levels of the OXY group differed significantly from those of the PL group 360 min after the infusion (4.2 vs. 3.1 ng/mL, p = 0.01) and tended to be higher at 450 min, 48 h, and 72 h (p = 0.08 for all).
Increased blood flow during normal CL development underlines the importance of angiogenesis during this process [8] because nearly every parenchymal cell in the mature CL is in contact with one or more capillaries [20]. The limited number of animals and large variation of the data in the present study were probably the reasons why increases in blood flow we observed did not reach statistical significant. CL growth following ovulation is rapid and comparable to that of tumors [4]. Although LH and PGF2α are two of the primary endocrine factors controlling bovine CL function [29], modulatory or supporting roles of OXY in luteal development and regression have also been reported [10,11]. In the present study OXY administration had no effect on the development of CLs, which continued to increase in size.
D7 of the estrus cycle coincides with the onset of the first follicular wave and a relatively increased concentration of estrogen [21]. It was previously reported that estrogens enhance the formation of endometrial OXY receptors, but P4 reduces the concentration of endometrial OXY receptors during the luteal phase by blocking the estrogen action [7]. On the other hand, a fully functional CL is present at the ovary on D7 and thus P4 production is high [21]. It is also known that the expression of OXY mRNA level is high during the early luteal phase in bovine CLs [9,28]. Additionally, OXY directly stimulates P4 secretion in luteal cells [15]. Based on these findings, continuous infusion of high doses of OXY during the early luteal phase would be expected to alter P4 concentrations. However, only a weak effect was observed in the present study similar to previous findings [27]. The supporting role of OXY to P4 concentration diminishes from D7 onwards of the estrus cycle [26]. This could explain why there was a tendency for P4 concentrations to increase in the present study, since the CLs of some cows were still sensitive to OXY while others were not. Shirasuna et al. [23] recently confirmed this dual role of luteal OXY as luteotropic (during the early luteal and mid-luteal phases) and luteolytic (during the late luteal phase after initiation of the luteolytic cascade), highlighting the modulatory effect of OXY on local secretion of vasoactive substances within the CL.
Infusion of OXY in sheep has been previously reported to evoke a modest increase in PGF2α secretion during the early and late but not the mid-luteal phases [5]. It seems likely that cyclical variation in the ability of OXY to evoke uterine PGF2α secretion is due to cyclical changes in the concentration of endometrial OXY receptors [12]. Gilbert et al. [6] have also reported that there is no increase in PGFM (a stable metabolite of PGF2α) concentration after OXY administration. Results of the present study, showing that OXY administration had no effect on endometrial production of PGF2α (measured as PGFM), support these findings. It is worth mention that large variations in PGFM concentrations were detected within both groups in our study. This could reflect variation of PGF2α secretion as early as D7 in each animal since high or low concentrations were detected in the same animals regardless the group they were assigned to in the Latin square design (data not shown). However such fluctuations are difficult to explain and worth further investigation.
It was previously reported that luteolytic doses of PGF2α affect blood flow in the mature CL [2]. The same effect was noticed in spontaneus luteolysis [16]. In contrast, no change in blood flow was observed in developing CLs (D4) after PGF2α administration since plasma P4 levels and CL volume continues to increase after PGF2α administration [16]. In the present study, OXY administration did not cause any increase in PGFM concentration. Although the CL is sensitive to luteolytic doses of PGF2α during mid-cycle (D7), it seems unaffected by OXY administration, even at relative high doses (100 IU). The possible explanations for this observation are either that there were not enough OXY receptors in the uterus, or there was not enough available PGF2α that could be released from the uterus. Nevertheless, the fact that there were no changes in CL blood flow, which continued to increase during and after infusion, supports the hypothesis, that OXY is not directly involved in the mechanism(s) governing blood flow in the developing CL. Further investigation is needed to elucidate the role of OXY in CL blood flow during different periods of the luteal phase.
Figures and Tables
Fig. 1
Luteal size (cm2) 72 h after oxytocin (OXY, p = 0.02) or placebo (PL, p = 0.001) infusion in Holstein-Friesian cows. Data represent mean ± SE.
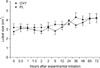
Fig. 2
Luteal blood flow (cm2) 72 h after OXY or PL infusion in Holstein-Friesian cows. Data represent mean ± SE.
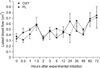
References
1. Acosta TJ, Hayashi KG, Ohtani M, Miyamoto A. Local changes in blood flow within the preovulatory follicle wall and early corpus luteum in cows. Reproduction. 2003. 125:759–767.


2. Acosta TJ, Miyamoto A. Vascular control of ovarian function: ovulation, corpus luteum formation and regression. Anim Reprod Sci. 2004. 82-83:127–140.


4. Fields MJ, Fields PA. Morphological characteristics of the bovine corpus luteum during the estrous cycle and pregnancy. Theriogenology. 1996. 45:1295–1325.


5. Flint APF, Sheldrick E. Continuous infusion of oxytocin prevents induction of uterine oxytocin receptor and blocks luteal regression in cyclic ewes. J Reprod Fertil. 1985. 75:623–631.


6. Gilbert CL, Lamming GE, Parkinson TJ, Flint AP, Wathes DC. Oxytocin infusion from day 10 after oestrus extends the luteal phase in non-pregnant cattle. J Reprod Fertil. 1989. 86:203–210.


7. Grazzini E, Guillon G, Mouillac B, Zingg HH. Inhibition of oxytocin receptor function by direct binding of progesterone. Nature. 1998. 392:509–512.


8. Herzog K, Brockhan-Ludemann M, Kaske M, Beindorff N, Paul V, Niemann H, Bollwein H. Luteal blood flow is a more appropriate indicator for luteal function during the bovine estrous cycle than luteal size. Theriogenology. 2010. 73:691–697.


9. Ivell R, Brackett KH, Fields MJ, Richter D. Ovulation triggers oxytocin gene expression in the bovine ovary. FEBS Lett. 1985. 190:263–267.


10. Jaroszewski J, Kotwica J. Reduction of ovarian oxytocin content from early luteal phase does not affect the corpus luteum secretory function in cattle. Reprod Nutr Dev. 1994. 34:175–182.


11. Kotwica J, Skarzynski D, Jaroszewski J, Williams GL, Bogacki M. Uterine secretion of prostaglandin F2alpha stimulated by different doses of oxytocin and released spontaneously during luteolysis in cattle. Reprod Nutr Dev. 1998. 38:217–226.


12. McCracken JA, Custer EE, Lamsa JC. Luteolysis: a neuroendocrine-mediated event. Physiol Rev. 1999. 79:263–323.


13. Meidan R, Milvae R, Weiss S, Levy N, Friedman A. Intraovarian regulation of luteolysis. J Reprod Fertil Suppl. 1999. 54:217–228.


14. Mishra DP, Meyer HHD, Prakash BS. Validation of a sensitive enzymeimmunoassay for 13,14-dihydro-15-keto-PGF2α in buffalo plasma and its application for reproductive health status monitoring. Anim Reprod Sci. 2003. 78:33–46.


15. Miyamoto A, Schams D. Oxytocin stimulates progesterone release from microdialyzed bovine corpus luteum in vitro. Biol Reprod. 1991. 44:1163–1170.


16. Miyamoto A, Shirasuna K, Hayashi KG, Kamada D, Awashima C, Kaneko E, Acosta TJ, Matsui M. A potential use of color ultrasound as a tool for reproductive management: New observations using color ultrasound scanning that were not possible with imaging only in black and white. J Reprod Dev. 2006. 52:153–160.


17. Niswender GD, Juengel JL, Silva PJ, Rollyson MK, McIntush EW. Mechanisms controlling the function and life span of the corpus luteum. Physiol Rev. 2000. 80:1–29.


18. Prakash BS, Meyer HH, Schallenberger E, van de Wiel DF. Development of a sensitive enzymeimmunoassay (EIA) for progesterone determination in unextracted bovine plasma using the second antibody technique. J Steroid Biochem. 1987. 28:623–627.


19. Pursley JR, Mee MO, Wiltbank MC. Synchronization of ovulation in dairy cows using PGF2α and GnRH. Theriogenology. 1995. 44:915–923.


21. Savio JD, Boland MP, Roche JF. Development of dominant follicles and length of ovarian cycles in post-partum dairy cows. J Reprod Fertil. 1990. 88:581–591.


22. Schams D, Berisha B. Regulation of corpus luteum function in cattle-an overview. Reprod Domest Anim. 2004. 39:241–251.


23. Shirasuna K, Shimizu T, Hayashi KG, Nagai K, Matsui M, Miyamoto A. Positive association, in local release, of luteal oxytocin with endothelin 1 and prostaglandin F2alpha during spontaneous luteolysis in the cow: a possible intermediatory role for luteolytic cascade within the corpus luteum. Biol Reprod. 2007. 76:965–970.


24. Silvia WJ, Raw RE. Regulation of pulsatile secretion of prostaglandin F2α from the ovine uterus by ovarian steroids. J Reprod Fertil. 1993. 98:341–347.


25. Skarzynski DJ, Bogacki M, Kotwica J. Changes in ovarian oxytocin secretion as an indicator of corpus luteum response to prostaglandin F2α treatment in cattle. Theriogenology. 1997. 48:733–742.


26. Skarzynski DJ, Jaroszewski JJ, Okuda K. Luteotropic mechanisms in the bovine corpus luteum: role of oxytocin, prostaglandin F2α, progesterone and noradrenaline. J Reprod Dev. 2001. 47:125–137.


27. Tallam SK, Walton JS, Johnson WH. Effects of oxytocin on cloprostenol-induced luteolysis, follicular growth, ovulation and corpus luteum function in heifers. Theriogenology. 2000. 53:963–979.


28. Wathes DC, Denning-Kendall PA. Control of synthesis and secretion of ovarian oxytocin in ruminants. J Reprod Fertil Suppl. 1992. 45:39–52.