Abstract
High-resolution electrogastrography (HR-EGG) presents a new paradigm in diagnosing and treating functional gastroduodenal disorders. Unlike traditional electrogastrography, HR-EGG allows for a more precise analysis of the gastric electrical activity, offering improved diagnostic accuracy. Recent studies have revealed the clinical potential of HR-EGG, particularly in detecting abnormal electrical patterns in patients with functional dyspepsia and gastroparesis, supporting the development of novel therapeutic strategies. The non-invasive HR-EGG method has shown promise in identifying new biomarkers. Moreover, further integration of artificial intelligence, is expected to enhance diagnostic efficiency and develop more refined treatment models for functional gastrointestinal disorders.
기능성 위십이지장질환은 명확한 기질적 원인이 없이 발생하는 질환이며 임상적으로 흔히 볼 수 있다. 로마 IV 기준에 따르면, 기능성 위십이지장질환은 기능성 소화불량, 만성 메스꺼움 및 구토 장애, 트림 및 반추 장애 등을 포함한다.1 기능성 위십이지장질환을 진단하려면, 환자가 증상을 호소할 때 기질적, 전신적, 또는 대사적 질환의 증거가 없어야 하며, 증상에 근거한 진단 기준을 충족해야 한다.1 위마비의 경우, 기질적 원인이 존재하더라도 기능성 메스꺼움 및 구토 장애와 유사한 임상 증상이 나타날 수 있다. 이로 인해 증상이 중복되며, 두 질환의 병태생리에 대한 이해가 부족할 경우 명확한 구별이 어려워질 수 있다.2
기능성 위십이지장질환의 진단은 주로 환자의 증상과 병력을 기반으로 이루어진다. 이를 위해 환자가 겪는 증상을 평가하며, 이러한 증상들이 기질적, 전신적, 또는 대사적 원인에 의한 것이 아닌지를 확인하기 위해 다양한 검사를 시행한다. 위내시경, 복부전산화단층촬영, 혈액 검사, 대변 검사 등이 주요 진단 도구로 활용되며, 이 과정에서 기질적 질환이 배제되면 기능성 위십이지장질환으로 진단하게 된다. 그러나 임상 병력과 검사만으로는 종종 기능적 질환과 기질적 질환을 구별하기에 충분하지 않으며,3,4 지속적인 증상을 보이는 환자들은 종종 다양한 질환을 배제하기 위해 광범위하고 고비용의 검사를 반복적으로 받는 경우가 많다.5,6
기능성 위십이지장질환 진단에서 핵심적인 문제 중 하나는, 이를 명확히 규명할 수 있는 구체적이고 객관적인 바이오마커가 여전히 부족하다는 점이다.4 위 신티그래피나 호흡을 이용한 위 배출 검사가 위 기능을 평가하는 주요 방법이지만, 최근 연구에 따르면 이 검사들이 증상과 어느 정도 연관이 있음을 보여주면서도 치료 반응과의 명확한 상관관계는 부족하여, 바이오마커로서의 임상적 유용성에 대한 의문이 제기되고 있다.7,8 일부 의료 기관에서는 십이지장 내압 측정이나 무선 운동성 캡슐 등 다양한 위 기능 검사를 시행하고 있지만, 이러한 검사들의 유용성은 제한적이며, 기존의 위 신티그래피 검사보다 뛰어난 정확도를 보이지는 못하고 있다.9-13 따라서 기능성 위십이지장질환을 보다 정확하고 객관적으로 진단하고 구별할 수 있는 새로운 접근 방식이 필요하다. 이상적인 진단 방법은 비침습적일 뿐만 아니라 대규모 인구에 쉽게 적용할 수 있으며, 임상적으로 신뢰할 수 있을 정도로 민감하고 특이적이어야 한다.
위의 전기 활동은 위 운동에 중요한 역할을 하며, 특히 서파(slow wave)는 위장관 근육 수축을 유도하는 전기 신호를 전달한다. 이러한 위장관의 전기 활동은 1922년 알바레즈가 위전도검사(electrogastrography)를 처음 설명한 이후, 잠재적인 임상 바이오마커로 오랫동안 주목받아 왔다.14 전통적인 위전도검사는 비침습적이고 임상에서 손쉽게 적용할 수 있는 검사 방법으로, 개선된 위전도 지표와 기능성 위십이지장질환 간에 흥미로운 연관성이 발견되면서 임상적 유용성에 대한 큰 기대를 모았다.15-17 그러나 광범위한 연구에도 불구하고 고식적 위전도검사는 여전히 일부 전문 센터에서만 임상적으로 활용되고 있는데 이는 위전도가 위의 병태생리를 명확히 규명하는 데 필요한 민감도와 특이성이 부족하다는 평가를 받으며, 여러 노이즈의 영향을 완전히 배제하지 못하고 증상과 일관된 연관성을 보여주지 못했기 때문이고 이로 인해 임상 진단 및 치료에서 확실한 역할을 갖고 있지 못한 상태이다.17-22
본고에서는 위의 전기생리학적 평가와 관련된 기존 연구와 최신 동향을 검토하고, 고식적 위전도 검사의 주요 한계에 대한 최근의 이해를 분석하고 위의 전기적 신호의 탐지 및 분석 방식을 혁신적으로 개선하는 새로운 접근법을 소개하고자 한다.
위장관의 연동운동(peristalsis)은 음식물이 소화관을 따라 이동하도록 돕는 일련의 근육 수축과 이완의 과정이다. 위장관의 근육 수축은 자율신경계와 내장 신경계의 복잡한 상호작용, 그리고 전기적 활동을 전달하는 서파에 의해 조절된다(Fig. 1).23 위 연동운동의 수축과정은 평활근 세포의 탈분극에 의해 시작된다. 장의 평활근 내에 존재하는 카할간질세포(interstitial cells of Cajal)는 서파를 생성함으로써 연동운동의 리듬을 조절하며, 이를 통해 위장 근육이 리드미컬하게 수축하게 되어 음식물이 한 방향으로 이동할 수 있게 된다. 이후 서파가 전파되면서 인접한 평활근 세포 네트워크의 탈분극에 의해 수축이 발생하고, 이 수축은 체액성, 기계적, 외부 신경 자극과 결합해 임계값을 넘는 탈분극을 유도하여 위장관의 연동 운동을 형성한다.23-25
카할간질세포는 위에서 서파의 생성과 전파를 지원하는 전기적 구조를 형성한다.15 카할간질세포는 자연적으로 탈분극되어 서파를 생성하는 고유한 주파수를 가지고 있으며 보통 위장관에서 카할간질세포는 가장 높은 고유 주파수를 가진 부위(주로 위장관의 중상부 체부에서 발견됨)를 중심으로 다른 카할간질세포들을 해당 주파수에 맞춰 동조화 시키는데 이 부위를 '페이스메이커 영역'이라고 한다.15
카할간질세포의 기능손실과 다양한 위장관 질환과의 관계가 보고된 바 있다.26 예를 들어, 당뇨병성 위마비(gastroparesis) 환자에서는 카할간질세포의 손상이 자주 나타나며, 이 손상은 서파의 비정상적인 전도와 밀접한 관련이 있다.27-29 또한, Chagas 병과 같은 특정 질환에서도 카할간질세포의 감소가 관찰되며, 이는 위장관 운동의 비정상적인 변화를 초래할 수 있다.30
위 서파와 기능성 소화불량증 환자의 연관성을 분석한 이전 연구에서 환자들은 건강한 대조군에 비해 공복 상태와 식사 후 상태 모두에서 정상적인 주파수(frequency)의 서파 비율이 유의하게 낮았고, 건강한 대조군에서는 식사 후 위전도의 주파수가 유의하게 증가한 반면, 환자군에서는 이러한 증가가 관찰되지 않았다.31
서파와 관련된 세포 외 전기적 전위는 이상적인 양상(biphasic)으로 전기적 추적에서 구분되며, 이는 파동의 앞부분에서 감지되는 양의 전위와 뒷부분에서 감지되는 음의 전위로 구성된다. 이 두 전위 사이의 가장 가파른 기울기는 파면이 전극 바로 아래를 통과하는 순간을 나타내며, 이를 활성화 시간(activation time)이라고 한다.32,33 전극 위치에서 연속적인 활성화 시간 간의 시간 차이는 주파수 계산에 사용되며, 피크와 트로프 사이의 전압 차이(peak to trough voltage)는 진폭(amplitude)을 나타낸다. 신체 표면에서 서파의 활성화를 프로파일링하는 것은 '원거리장'(far-field) 측정으로, 이 과정에서 넓은 활성화 영역이 합산되어 더 큰 전위 진동이 생성된다. 이는 탈분극과 재분극의 쌍극자(depolarization and repolarization dipoles)를 반영한다.34,35
위전도검사(conventional electrogastrography)는 피부 표면 전극(cutaneous electrode)을 이용하여 위의 전기적 활동을 기록하는 방법으로, 임상증상을 보이는 환자에서 비정상적인 위 전기 활동이 발견되면서 기초 생리학 연구와 임상 적용 양측에서 많은 관심을 받게 되었다. 위전도검사는 다양한 방법으로 측정할 수 있는데 복부에서 피부 표면 전극을 이용해 측정하거나, 점막 전극(mucosal electrode) 또는 장막 전극(serosal electrode)을 사용해 측정할 수 있다. 그러나 장막 전극을 이용한 위전도 측정은 전극을 배치하기 위해 외과적 수술이 필요하므로 임상에서 널리 사용되지 않았으며, 주로 피부 표면 전극을 통한 위전도 측정이 시행되었다.
위전도는 상복부에 피부 표면 전극을 붙여 측정한다(Fig. 2). 보통 자극이 적은 음식을 제공한 후, 식사 전과 후에 전기 신호를 기록한다. 주로 위장의 서파 주파수와 전력(power metrics)을 측정하며, 이를 분석하기 위해 컴퓨터를 이용한 스펙트럼 분석(computerized spectral analysis)을 사용한다.
이후 위전도 측정을 위한 다양한 전극 구성이 도입되었으며, 초기에는 주로 단일 전극 쌍을 사용하는 방식이 많이 활용되었으나14 1990–2000년대에 다중 채널 방식이 도입되면서, 더욱 정밀한 분석이 가능해졌다.36,37 이 방법에서는 일반적으로 위축을 따라 4개의 전극을 배치하고, 검상돌기 부위에 기준 전극, 왼쪽 늑골 가장자리에는 접지 전극을 부착한다. 다중 채널 방식은 위의 서파 전파를 재현성 있게 분석할 수 있으며, 한 연구에서는 이 방법을 통해 건강한 대조군과 소화불량 환자 사이에서 서파의 차이가 나타날 수 있음을 보여주었다.38
위전도검사는 연구에서는 비교적 유용하게 활용되었으나,실제 임상상황에서는 그 기술적 한계가 오랫동안 지적되어 왔다.
위전도검사 시 전극을 보통 위의 전정부에 위치시키려 하지만 사람마다 체형과 위의 위치가 다양하여 정확한 위치를 찾는 것이 어렵다.18,39,40 이로 인해 일반적인 위전도검사의 다중 채널 전극 배열이 위에 정확히 맞지 않는 경우가 발생한다. 또한 복벽을 통해서 측정되는 위의 전기 신호는 전극이 위에 가까이 위치하더라도 그 진폭이 상당히 약한데(~50–300 μV peak to peak)18,19 이 전기 신호는 위에서 거리가 멀어질수록 급격히 감소하여41 신뢰할 수 있는 기준 이하로 줄어들게 된다. 한 연구에서는 위전도검사 전극의 위치가 위에서 조금만 벗어나도 부정확한 데이터가 나올 수 있다는 것을 보여주었다.42
또한, 고식적 위전도검사의 스펙트럼 분석에서는 서파의 주파수를 중요한 지표로 사용했지만, 이 주파수만으로는 신뢰할 수 있는 임상진단 관련 결과를 얻기 어렵다(예: '위장 서맥' 은 분당 2.5회 미만(cpm), '위장 빈맥'은 분당 3.75회 이상, 정상 범위는 그 사이).17
위 서파의 전파 패턴과 방향을 더 정확히 파악하기 위해 밀집된 전극 배열을 사용하여 위마비 환자(당뇨, 특발성, 수술 후 원인) 및 기능성 소화불량 환자 등에서 위 전기신호를 측정하는 여러 고해상도 위전도검사 연구에 따르면, 서파의 주파수 지표만으로는 임상적으로 의미 있는 위전도의 이상신호를 감지하기에는 충분하지 않다는 것이 제시되었다.18,43-48 복강경 수술이나 복강경 검사를 통해 위의 장막 표면에서 직접 위의 전기신호를 측정한 연구들은 보다 정확한 데이터를 제공하며, 기능성 위십이지장질환 환자들에서 정상 주파수 범위 내에서도 다양한 율동 부정의 형태가 나타날 수 있음을 보여주었다. 따라서, 전극이 최적의 위치에 있더라도 고식적 위전도 스펙트럼 분석만으로는 이러한 위전도의 이상신호를 감지하지 못할 가능성이 있다.
Lammers 등49이 개발한 고해상도 위전도검사(high-resolution electrogastrography, HR-EGG)의 등장은 위장관 전기 생리학 분야에 획기적인 발전을 가져왔다. 고해상도 위전도검사는 위의 전기 활동을 보다 정밀하고 상세하게 측정하는 방법으로 많은 수의 전극을 밀집하게 배치하여, 위장관에서 발생하는 서파의 전기적 전도를 보다 정확하게 측정할 수 있다. 고해상도 위전도검사는 현재 임상에서 널리 사용되고 있는 뇌파 검사와 심장 생리 검사와 같은 기술을 기반으로 개발되었다(Fig. 3).
고해상도 위전도검사를 위해 여러 종류의 전극이 개발되었고, 다양한 동물 실험과 임상 실험이 진행되었다. 실험실에서 사용하는 전극뿐만 아니라, 개복수술이 필요한 장막 전극, 복강경 장치를 이용한 전극, 그리고 위장관 내시경에 사용하는 전극 등 다양한 형태의 전극이 사용되었다.50-52 이 연구들을 통해 다양한 위전도 측정 방식이 시험 적용되었고, 위전도 신호 처리, 분석, 시각화 방법이 발전하였다.53
고해상도 위전도검사는 인체 위장관의 전기 신호에 대한 더 깊은 이해를 제공하며, 이를 통해 비정상적 신호와 정상 신호를 비교할 수 있는 기준을 설정할 수 있게 되었다. 또한, 위 전기 신호가 신체 표면으로 전달되는 과정을 이해하는 데 기여하며, 이 데이터를 다층 계산 모델에 통합함으로써 위장관의 생리학적 지식을 확장하는 데 유용할 수 있다.54,55
고해상도 위전도검사는 기존의 주파수와 진폭 중심의 접근이 아닌, 서파의 전파 패턴을 기반으로 한 위장관 율동부정의새로운 분류 체계를 제안한다.43 이 체계는 기존 심장 전기생리검사 방식을 기반으로, 위전도의 비정상적인 신호의 시작과 전도 이상을 구분할 수 있다.15 한 연구에서는 중증 위마비 환자와 원인 불명의 만성 오심 및 구토 환자에서 고해상도 위전도검사를 통해 비정상적인 신호 패턴을 확인했으며, 고식적 위전도보다 더 우수한 임상적 연관성을 보여줄 가능성을 확인했다.43,44
그러나 고해상도 위전도검사를 위해서는 침습적인 과정이 필요하며, 임상적 효용성을 평가하기 위한 데이터가 부족해 아직 임상적으로 널리 적용되기에는 어려움이 있다.
현재까지 진행된 위전도검사 연구 결과에 따르면, 고식적 위전도검사를 개선하기 위한 유망한 전략 중 하나는 고해상도 위전도검사와 고식적 위전도검사 방식을 결합하는 것이다. 이를 통해 비침습적 시스템과 침습적 시스템의 한계를 상호 보완할 수 있다.56 이 통합된 접근 방식은 다양한 지표, 특히 위 서파의 전파 양상을 기반으로 위장관 운동과 관련된 전기적 이상을 더욱 정밀하게 규명할 수 있는 가능성을 제시한다. 또한, 비침습적 시스템 기반의 더 정밀한 진단과 맞춤형 치료를 가능하게 할 것이다.
비침습적 시스템은 고식적 위전도검사처럼 위에서 멀리 떨어진 복벽에서 위장관 전기 신호를 측정하기 때문에 정밀한 데이터를 얻는 데는 여전히 기술적인 난관이 존재한다. 여기에는 위장의 해부학적 변동성을 고려하여 전극과 피부 간의 적절한 접촉을 유지할 수 있는 고밀도 전극 패널 구축, 관련 배선의 효율적인 관리, 그리고 위장관 전기 신호를 안정적으로 수집할 수 있는 확장 가능한 다채널 신호 수신 시스템의 확보가 포함된다. 현재까지 이러한 요구를 충족하는 적절한 대역폭과 높은 충실도의 다채널 신호 수신 시스템은 매우 고가이거나 임상 적용이 어렵다는 한계가 있었다.53 최근에는 다채널 디지털 생체전기 신호 증폭기를 위장관에 적용하려는 시도가 이루어지면서, 더 저렴한 비용으로 기기를 사용할 수 있는 방안이 제시되고 있다.57,58
복벽에서 위장관의 전기 신호를 정밀하게 측정하기 위해 사용되는 고밀도 전극 패널은, 여전히 복벽을 통과하는 동안 서파의 감쇠 현상에 영향을 받을 수 있다. 이를 해결하려면, 신호가 약해지거나 잡음이 섞인 상황에서도 정확한 데이터를 얻을 수 있도록 신호 대 잡음비를 개선하고, 여러 신호가 합쳐지는 것을 처리할 수 있는 정교한 알고리즘이 필요하다.54 최근 Gharibans 등18은 경피 고해상도 위전도검사(cutaneous high-resolution electrogastrography) 기술의 임상적 유용성을 보여주며, 비침습적인 방법으로 위장관의 전기 신호를 시공간적 데이터로 성공적으로 구현하였다. 이 연구에서는 모두 32명의 환자(위마비 환자 18명, 정상 위배출능의 기능성 소화불량 환자 7명, 대조군 7명)를 대상으로 경피 고해상도 위전도검사를 시행하였다. 95 mm² 크기의 5×5 배열 신생아용 심장 전극을 사용하여(Fig. 4) 식사 전 30분과 식사 후 60분 동안 위장관 전기 신호를 측정했다. 위의 해부학적 변동성을 보완하기 위해 연구자들은 환자의 복부 전산화단층촬영 결과를 참고해 전극 배열을 위 끝부분 근처에 정확하게 배치했다. 또한, 새로운 알고리즘을 활용해 불필요한 신호를 제거하고 서파의 방향과 속도를 분석하였다. 연구결과 증상이 있는 환자의 44%에서 서파의 비정상적인 역방향 전파나 지속적인 파동이 발견되었고, 이를 통해 증상이 있는 환자와 대조군을 명확히 구분할 수 있었다. 특히, 비정상적인 느린 파동의 방향은 복부 팽창과 조기 포만감 같은 위장 증상과 밀접하게 관련된 것으로 나타났습니다. 반면, 고식적 위전도 지표는 이러한 연관성을 보여주지 못했다.
위장관 전기생리학 분야는 최근 몇 년간 상당한 발전을 이루었지만, 여전히 해결해야 할 과제가 많이 남아있다. 특정 전기 신호 이상이 특정 위장관 질환과 어떻게 연관되는지, 그리고 이러한 신호가 질환 진단에 얼마나 유용한지를 명확히 규명할 필요가 있다. 더 나아가, 위장관 전기 신호에 대한 검사가 실제 치료에 어떤 영향을 미칠 수 있는지 확인하는 것도 중요하다.
고해상도 위전도검사는 위장관의 전기적 활동을 보다 정밀하게 공간적으로 분석할 수 있는 새로운 방법을 제시했으며, 최근에는 이를 통해 비침습적인 진단 가능성을 입증하고 있다. 또한, 고해상도 위전도 검사에서 수집된 방대한 데이터는 추후 인공지능을 활용한 질환 예측 모델 개발에 중요한 역할을 할 것으로 추측되며 이는 기능성 위십이지장질환의 진단과 맞춤형 치료에 큰 기여를 할 수 있을 것으로 기대된다.55
약물치료, 심리적 치료, 말초 신경 자극과 같은 다양한 자극이 위장관의 전기적 신호에 미치는 영향을 연구하는 것도 중요하며 이는 복잡한 위장관 기능의 문제를 더 깊이 이해하고, 각 자극이 치료에 어떤 영향을 미치는지 평가하는 데 중요한 역할을 할 것이다.
위 전기생리학의 발전이 새로운 치료법으로 이어질 수 있을지는 궁금한 부분이다. 아직 그 답은 명확하지 않지만, 많은 연구가 그 기반을 다지고 있다.59 고해상도 위전도검사에 결합된 전기 활동을 조정하는 장치와60-62 위 신경 자극 기술의 임상적용63,64이 연구되고 있다. 또한, 위의 비정상적인 전도 부분의 고주파적 제거 방법도65 동물 연구에서 시행된 바 있다.
고해상도 위전도검사를 기반으로 한 새로운 치료법 적용에 대한 연구는 지속적으로 이루어지고 있으며, 기존의 심장 전기생리학적 접근을 위장관 분야에 적용할 수 있는 방법 또한 적극적으로 연구될 필요가 있다. 이러한 융합적 연구는 기능성 위십이지장질환의 진단과 치료에 중요한 발전을 이끌어낼 것이다.
Notes
REFERENCES
1. Stanghellini V, Chan FK, Hasler WL, et al. 2016; Gastroduodenal disorders. Gastroenterology. 150:1380–1392. DOI: 10.1053/j.gastro.2016.02.011. PMID: 27147122.
2. Harer KN, Pasricha PJ. 2016; Chronic unexplained nausea and vomiting or gastric neuromuscular dysfunction (GND)? An update on nomenclature, pathophysiology and treatment, and relationship to gastroparesis. Curr Treat Options Gastroenterol. 14:410–419. DOI: 10.1007/s11938-016-0113-z. PMID: 27722801. PMCID: PMC5102760.
3. Sood R, Ford AC. 2016; Diagnosis: Rome IV criteria for FGIDs - an improvement or more of the same? Nat Rev Gastroenterol Hepatol. 13:501–502. DOI: 10.1038/nrgastro.2016.110. PMID: 27407043.
4. Camilleri M, Chedid V. 2020; Actionable biomarkers: the key to resolving disorders of gastrointestinal function. Gut. 69:1730–1737. DOI: 10.1136/gutjnl-2019-320325. PMID: 32269066.
5. Linedale EC, Shahzad MA, Kellie AR, Mikocka-Walus A, Gibson PR, Andrews JM. 2017; Referrals to a tertiary hospital: A window into clinical management issues in functional gastrointestinal disorders. JGH Open. 1:84–91. DOI: 10.1002/jgh3.12015. PMID: 30483542. PMCID: PMC6207011.
6. Linedale EC, Chur-Hansen A, Mikocka-Walus A, Gibson PR, Andrews JM. 2016; Uncertain diagnostic language affects further studies, endoscopies, and repeat consultations for patients with functional gastrointestinal disorders. Clin Gastroenterol Hepatol. 14:1735–1741.e1. DOI: 10.1016/j.cgh.2016.06.030. PMID: 27404968.
7. Vijayvargiya P, Jameie-Oskooei S, Camilleri M, Chedid V, Erwin PJ, Murad MH. 2019; Association between delayed gastric emptying and upper gastrointestinal symptoms: a systematic review and meta-analysis. Gut. 68:804–813. DOI: 10.1136/gutjnl-2018-316405. PMID: 29860241.
8. Janssen P, Harris MS, Jones M, et al. 2013; The relation between symptom improvement and gastric emptying in the treatment of diabetic and idiopathic gastroparesis. Am J Gastroenterol. 108:1382–1391. DOI: 10.1038/ajg.2013.118. PMID: 24005344.
9. Phillips SF, Camilleri M. 1992; Antroduodenal manometry. Dig Dis Sci. 37:1305–1308. DOI: 10.1007/BF01296580. PMID: 1499459.
10. Camilleri M, Hasler WL, Parkman HP, Quigley EM, Soffer E. 1998; Measurement of gastrointestinal motility in the GI laboratory. Gastroenterology. 115:747–762. DOI: 10.1016/S0016-5085(98)70155-6. PMID: 9721173.
11. Braden B, Adams S, Duan LP, et al. 1995; The [13C]acetate breath test accurately reflects gastric emptying of liquids in both liquid and semisolid test meals. Gastroenterology. 108:1048–1055. DOI: 10.1016/0016-5085(95)90202-3. PMID: 7698571.
12. Maes BD, Ghoos YF, Rutgeerts PJ, Hiele MI, Geypens B, Vantrappen G. 1994; [*C]octanoic acid breath test to measure gastric emptying rate of solids. Dig Dis Sci. 39(12 Suppl):104S–106S. DOI: 10.1007/BF02300385. PMID: 7995200.
13. Szarka LA, Camilleri M, Vella A, et al. 2008; A stable isotope breath test with a standard meal for abnormal gastric emptying of solids in the clinic and in research. Clin Gastroenterol Hepatol. 6:635–643.e1. DOI: 10.1016/j.cgh.2008.01.009. PMID: 18406670. PMCID: PMC3739971.
14. Alverez W. 1922; The electrogastrogram and what it shows. J Am Med Assoc. 78:1116–1119. DOI: 10.1001/jama.1922.02640680020008.
15. O'Grady G, Wang TH, Du P, Angeli T, Lammers WJ, Cheng LK. 2014; Recent progress in gastric arrhythmia: pathophysiology, clinical significance and future horizons. Clin Exp Pharmacol Physiol. 41:854–862. DOI: 10.1111/1440-1681.12288. PMID: 25115692. PMCID: PMC4359928.
16. Riezzo G, Russo F, Indrio F. 2013; Electrogastrography in adults and children: the strength, pitfalls, and clinical significance of the cutaneous recording of the gastric electrical activity. Biomed Res Int. 2013:282757. DOI: 10.1155/2013/282757. PMID: 23762836. PMCID: PMC3677658.
17. Parkman HP, Hasler WL, Barnett JL, Eaker EY. American Motility Society Clinical GI Motility Testing Task Force. 2003; Electrogastrography: a document prepared by the gastric section of the American Motility Society Clinical GI Motility Testing Task Force. Neurogastroenterol Motil. 15:89–102. DOI: 10.1046/j.1365-2982.2003.00396.x. PMID: 12680908.
18. Gharibans AA, Coleman TP, Mousa H, Kunkel DC. 2019; Spatial patterns from high-resolution electrogastrography correlate with severity of symptoms in patients with functional dyspepsia and gastroparesis. Clin Gastroenterol Hepatol. 17:2668–2677. DOI: 10.1016/j.cgh.2019.04.039. PMID: 31009794.
19. Gharibans AA, Smarr BL, Kunkel DC, Kriegsfeld LJ, Mousa HM, Coleman TP. 2018; Artifact rejection methodology enables continuous, noninvasive measurement of gastric myoelectric activity in ambulatory subjects. Sci Rep. 8:5019. DOI: 10.1038/s41598-018-23302-9. PMID: 29568042. PMCID: PMC5864836.
20. Abid S, Lindberg G. 2007; Electrogastrography: poor correlation with antro-duodenal manometry and doubtful clinical usefulness in adults. World J Gastroenterol. 13:5101–5107. DOI: 10.3748/wjg.v13.i38.5101. PMID: 17876876. PMCID: PMC4434640.
21. Chang FY. 2005; Electrogastrography: basic knowledge, recording, processing and its clinical applications. J Gastroenterol Hepatol. 20:502–516. DOI: 10.1111/j.1440-1746.2004.03751.x. PMID: 15836697.
22. Tabor S, Furgała A, Laskiewicz J, Thor PJ. 2002; Electrogastrography and manometry of the stomach in patients with disturbances of basic electrical rhythm. Folia Med Cracov. 43:29–41. PMID: 12815796.
23. Huizinga JD, Lammers WJ. 2009; Gut peristalsis is governed by a multitude of cooperating mechanisms. Am J Physiol Gastrointest Liver Physiol. 296:G1–8. DOI: 10.1152/ajpgi.90380.2008. PMID: 18988693.
24. Owyang C, Hasler WL. 2002; Physiology and pathophysiology of the interstitial cells of Cajal: from bench to bedside. VI. Pathogenesis and therapeutic approaches to human gastric dysrhythmias. Am J Physiol Gastrointest Liver Physiol. 283:G8–15. DOI: 10.1152/ajpgi.00095.2002. PMID: 12065286.
25. Chen JD, Qian L, Ouyang H, Yin J. 2003; Gastric electrical stimulation with short pulses reduces vomiting but not dysrhythmias in dogs. Gastroenterology. 124:401–409. DOI: 10.1053/gast.2003.50048.
26. Choi EL, Taheri N, Tan E, Matsumoto K, Hayashi Y. 2023; The crucial role of the interstitial cells of Cajal in neurointestinal diseases. Biomolecules. 13:1358. DOI: 10.3390/biom13091358. PMID: 37759758. PMCID: PMC10526372.
27. Southwell BR. 2008; Loss of interstitial cells of Cajal may be central to poor intestinal motility in diabetes mellitus. J Gastroenterol Hepatol. 23:505–507. DOI: 10.1111/j.1440-1746.2008.05363.x. PMID: 18397479.
28. Yamamoto T, Watabe K, Nakahara M, et al. 2008; Disturbed gastrointestinal motility and decreased interstitial cells of Cajal in diabetic db/db mice. J Gastroenterol Hepatol. 23:660–667. DOI: 10.1111/j.1440-1746.2008.05326.x. PMID: 18341539.
29. Ordög T. 2008; Interstitial cells of Cajal in diabetic gastroenteropathy. Neurogastroenterol Motil. 20:8–18. DOI: 10.1111/j.1365-2982.2007.01056.x. PMID: 18173559.
30. Jabari S, da Silveira AB, de Oliveira EC, et al. 2013; Interstitial cells of Cajal: crucial for the development of megacolon in human Chagas' disease? Colorectal Dis. 15:e592–e598. DOI: 10.1111/codi.12331. PMID: 23810202.
31. Lin Z, Eaker EY, Sarosiek I, McCallum RW. 1999; Gastric myoelectrical activity and gastric emptying in patients with functional dyspepsia. Am J Gastroenterol. 94:2384–2389. DOI: 10.1111/j.1572-0241.1999.01362.x. PMID: 10483996.
32. Angeli TR, Du P, Paskaranandavadivel N, et al. 2013; The bioelectrical basis and validity of gastrointestinal extracellular slow wave recordings. J Physiol. 591:4567–4579. DOI: 10.1113/jphysiol.2013.254292. PMID: 23713030. PMCID: PMC3784199.
33. Bayguinov O, Hennig GW, Sanders KM. 2011; Movement based artifacts may contaminate extracellular electrical recordings from GI muscles. Neurogastroenterol Motil. 23:1029–1042. e498. DOI: 10.1111/j.1365-2982.2011.01784.x. PMID: 21951699. PMCID: PMC4793914.
34. Smout AJ, van der Schee EJ, Grashuis JL. 1980; What is measured in electrogastrography? Dig Dis Sci. 25:179–187. DOI: 10.1007/BF01308136. PMID: 7371462.
35. Kajimoto T. 1995; Relation between electrogastrography and gastric electromyogram, mechanical activity. J Smooth Muscle Res. 31:93–107. DOI: 10.1540/jsmr.31.93. PMID: 8563060.
36. Yin J, Chen JD. 2013; Electrogastrography: methodology, validation and applications. J Neurogastroenterol Motil. 19:5–17. DOI: 10.5056/jnm.2013.19.1.5. PMID: 23350042. PMCID: PMC3548127.
37. Simonian HP, Panganamamula K, Chen JZ, Fisher RS, Parkman HP. 2004; Multichannel electrogastrography (EGG) in symptomatic patients: a single center study. Am J Gastroenterol. 99:478–485. DOI: 10.1111/j.1572-0241.2004.04103.x. PMID: 15056089.
38. Lin X, Chen JZ. 2001; Abnormal gastric slow waves in patients with functional dyspepsia assessed by multichannel electrogastrography. Am J Physiol Gastrointest Liver Physiol. 280:G1370–G1375. DOI: 10.1152/ajpgi.2001.280.6.G1370. PMID: 11352832.
39. Calder S, O'Grady G, Cheng LK, Du P. 2020; A simulated anatomically accurate investigation into the effects of biodiversity on electrogastrography. IEEE Trans Biomed Eng. 67:868–875. DOI: 10.1109/TBME.2019.2922449. PMID: 31199250.
40. Lamart S, Imran R, Simon SL, et al. 2013; Prediction of the location and size of the stomach using patient characteristics for retrospective radiation dose estimation following radiotherapy. Phys Med Biol. 58:8739–8753. DOI: 10.1088/0031-9155/58/24/8739. PMID: 24301086. PMCID: PMC4160803.
41. He B, Cohen RJ. 1992; Body surface Laplacian ECG mapping. IEEE Trans Biomed Eng. 39:1179–1191. DOI: 10.1109/10.168684. PMID: 1487281.
42. Gharibans AA, Coleman T, Mousa H, Kunkel D. 2018; High-density multichannel electrode Array improves the accuracy of cutaneous electrogastrography across subjects with wide-ranging BMI: 1235. Am J Gastroenterol. 113:S708. DOI: 10.14309/00000434-201810001-01235.
43. Angeli TR, Cheng LK, Du P, et al. 2015; Loss of interstitial cells of Cajal and patterns of gastric dysrhythmia in patients with chronic unexplained nausea and vomiting. Gastroenterology. 149:56–66.e5. DOI: 10.1053/j.gastro.2015.04.003. PMID: 25863217. PMCID: PMC4617790.
44. O'Grady G, Angeli TR, Du P, et al. 2012; Abnormal initiation and conduction of slow-wave activity in gastroparesis, defined by highresolution electrical mapping. Gastroenterology. 143:589–598.e3. DOI: 10.1053/j.gastro.2012.05.036. PMID: 22643349. PMCID: PMC3429650.
45. Berry R, Cheng LK, Du P, et al. 2017; Patterns of abnormal gastric pacemaking after sleeve gastrectomy defined by laparoscopic high-resolution electrical mapping. Obes Surg. 27:1929–1937. DOI: 10.1007/s11695-017-2597-6. PMID: 28213666.
46. Wang TH, Angeli TR, Beban G, et al. 2019; Slow-wave coupling across a gastroduodenal anastomosis as a mechanism for postsurgical gastric dysfunction: evidence for a "gastrointestinal aberrant pathway". Am J Physiol Gastrointest Liver Physiol. 317:G141–G146. DOI: 10.1152/ajpgi.00002.2019. PMID: 31169993. PMCID: PMC6734376.
47. Ray K. 2012; Motility: Mapping gastric dysrhythmias in gastroparesis--a slow wave of electrical activity. Nat Rev Gastroenterol Hepatol. 9:363. DOI: 10.1038/nrgastro.2012.116. PMID: 22710570.
48. Chen JD, Lin Z, McCALLUM RW. 1998; Gastric electrical stimulation in patients with gastroparesis. J Gastroenterol Hepatol. 13:S232–S236. DOI: 10.1111/j.1440-1746.1998.tb01883.x. PMID: 28976676.
49. Lammers WJ, Stephen B, Arafat K, Manefield GW. 1996; High resolution electrical mapping in the gastrointestinal system: initial results. Neurogastroenterol Motil. 8:207–216. DOI: 10.1111/j.1365-2982.1996.tb00259.x. PMID: 8878080.
50. Du P, O'Grady G, Egbuji JU, et al. 2009; High-resolution mapping of in vivo gastrointestinal slow wave activity using flexible printed circuit board electrodes: methodology and validation. Ann Biomed Eng. 37:839–846. DOI: 10.1007/s10439-009-9654-9. PMID: 19224368. PMCID: PMC4090363.
51. O'Grady G, Du P, Egbuji JU, et al. 2009; A novel laparoscopic device for measuring gastrointestinal slow-wave activity. Surg Endosc. 23:2842–2848. DOI: 10.1007/s00464-009-0515-2. PMID: 19466491. PMCID: PMC4106912.
52. Angeli TR, Du P, Paskaranandavadivel N, et al. High-resolution electrical mapping of porcine gastric slow-wave propagation from the mucosal surface. Neurogastroenterol Motil. 2017; May. doi: 10.1111/nmo.13010. DOI: 10.1111/nmo.13010. PMID: 28035728. PMCID: PMC5393964.
53. O'Grady G, Angeli TR, Paskaranandavadivel N, et al. 2019; Methods for high-resolution electrical mapping in the gastrointestinal tract. IEEE Rev Biomed Eng. 12:287–302. DOI: 10.1109/RBME.2018.2867555. PMID: 30176605.
54. Du P, O'Grady G, Cheng LK, Pullan AJ. 2010; A multiscale model of the electrophysiological basis of the human electrogastrogram. Biophys J. 99:2784–2792. DOI: 10.1016/j.bpj.2010.08.067. PMID: 21044575. PMCID: PMC2965998.
55. Calder S, O'Grady G, Cheng LK, Peng Du. 2017; A theoretical analysis of electrogastrography (EGG) signatures associated with gastric dysrhythmias. IEEE Trans Biomed Eng. 64:1592–1601. DOI: 10.1109/TBME.2016.2614277. PMID: 28113227.
56. Du P, O'Grady G, Gao J, Sathar S, Cheng LK. 2013; Toward the virtual stomach: progress in multiscale modeling of gastric electrophysiology and motility. Wiley Interdiscip Rev Syst Biol Med. 5:481–493. DOI: 10.1002/wsbm.1218. PMID: 23463750. PMCID: PMC3681930.
57. Erickson JC, Hayes JA, Bustamante M, et al. 2018; Intsy: a low-cost, open-source, wireless multi-channel bioamplifier system. Physiol Meas. 39:035008. DOI: 10.1088/1361-6579/aaad51. PMID: 29406314.
58. Erickson JC, Reed B, Wharton J, Thapa U, Robey J, Shrestha R. 2020; Open-source 128-channel bioamplifier module for ambulatory monitoring of gastrointestinal electrical activity. Annu Int Conf IEEE Eng Med Biol Soc. 2020:4429–4432. DOI: 10.1109/EMBC44109.2020.9175582. PMID: 33018977.
59. Kim SH, Kim HB, Chun HJ, et al. 2020; Minimally invasive gastric electrical stimulation using a newly developed wireless gastrostimulator: A pilot animal study. J Neurogastroenterol Motil. 26:410–416. DOI: 10.5056/jnm20063. PMID: 32606261. PMCID: PMC7329147.
60. McCallum RW, Chen JD, Lin Z, Schirmer BD, Williams RD, Ross RA. 1998; Gastric pacing improves emptying and symptoms in patients with gastroparesis. Gastroenterology. 114:456–461. DOI: 10.1016/S0016-5085(98)70528-1. PMID: 9496935.
61. Alighaleh S, Cheng LK, Angeli TR, et al. 2019; A novel gastric pacing device to modulate slow waves and assessment by high-resolution mapping. IEEE Trans Biomed Eng. 66:2823–2830. DOI: 10.1109/TBME.2019.2896624. PMID: 30735980.
62. O'Grady G, Du P, Lammers WJ, et al. 2010; High-resolution entrainment mapping of gastric pacing: a new analytical tool. Am J Physiol Gastrointest Liver Physiol. 298:G314–G321. DOI: 10.1152/ajpgi.00389.2009. PMID: 19926815. PMCID: PMC2822498.
63. Lal N, Livemore S, Dunne D, Khan I. 2015; Gastric electrical stimulation with the enterra system: A systematic review. Gastroenterol Res Pract. 2015:762972. DOI: 10.1155/2015/762972. PMID: 26246804. PMCID: PMC4515290.
64. Yin J, Abell TD, McCallum RW, Chen JD. 2012; Gastric neuromodulation with Enterra system for nausea and vomiting in patients with gastroparesis. Neuromodulation. 15:224–231. discussion 231DOI: 10.1111/j.1525-1403.2012.00429.x. PMID: 22364275.
65. Aghababaie Z, Chan CA, Paskaranandavadivel N, et al. 2019; Feasibility of high-resolution electrical mapping for characterizing conduction blocks created by gastric ablation. Annu Int Conf IEEE Eng Med Biol Soc. 2019:170–173. DOI: 10.1109/EMBC.2019.8856406. PMID: 31945871.
66. Blair PJ, Rhee PL, Sanders KM, Ward SM. 2014; The significance of interstitial cells in neurogastroenterology. J Neurogastroenterol Motil. 20:294–317. DOI: 10.5056/jnm14060. PMID: 24948131. PMCID: PMC4102150.
67. Komorowski D, Pietraszek S, Tkacz E, Provaznik I. 2015; The extraction of the new components from electrogastrogram (EGG), using both adaptive filtering and electrocardiographic (ECG) derived respiration signal. Biomed Eng Online. 14:60. DOI: 10.1186/s12938-015-0054-0. PMID: 26099312. PMCID: PMC4477495.
68. Carson DA, Robertson S, Wang TH, et al. 2023; The impact and clinical implications of gastric surgery on the gastric conduction system. Foregut. 3:29–44. DOI: 10.1177/26345161221130117.
Fig. 1
Slow waves observed in the gastric antrum. Phase 0 is the resting membrane potential, Phase 1 is rapid depolarization due to calcium ion influx, Phase 2 is the peak representing maximum depolarization, Phase 3 is the start of repolarization as potassium ions exit, and Phase 4 is gradual repolarization, returning to the resting potential. Adapted from Blair et al.66 with permission from the Korean Society of Neurogastroenterology and Motility.
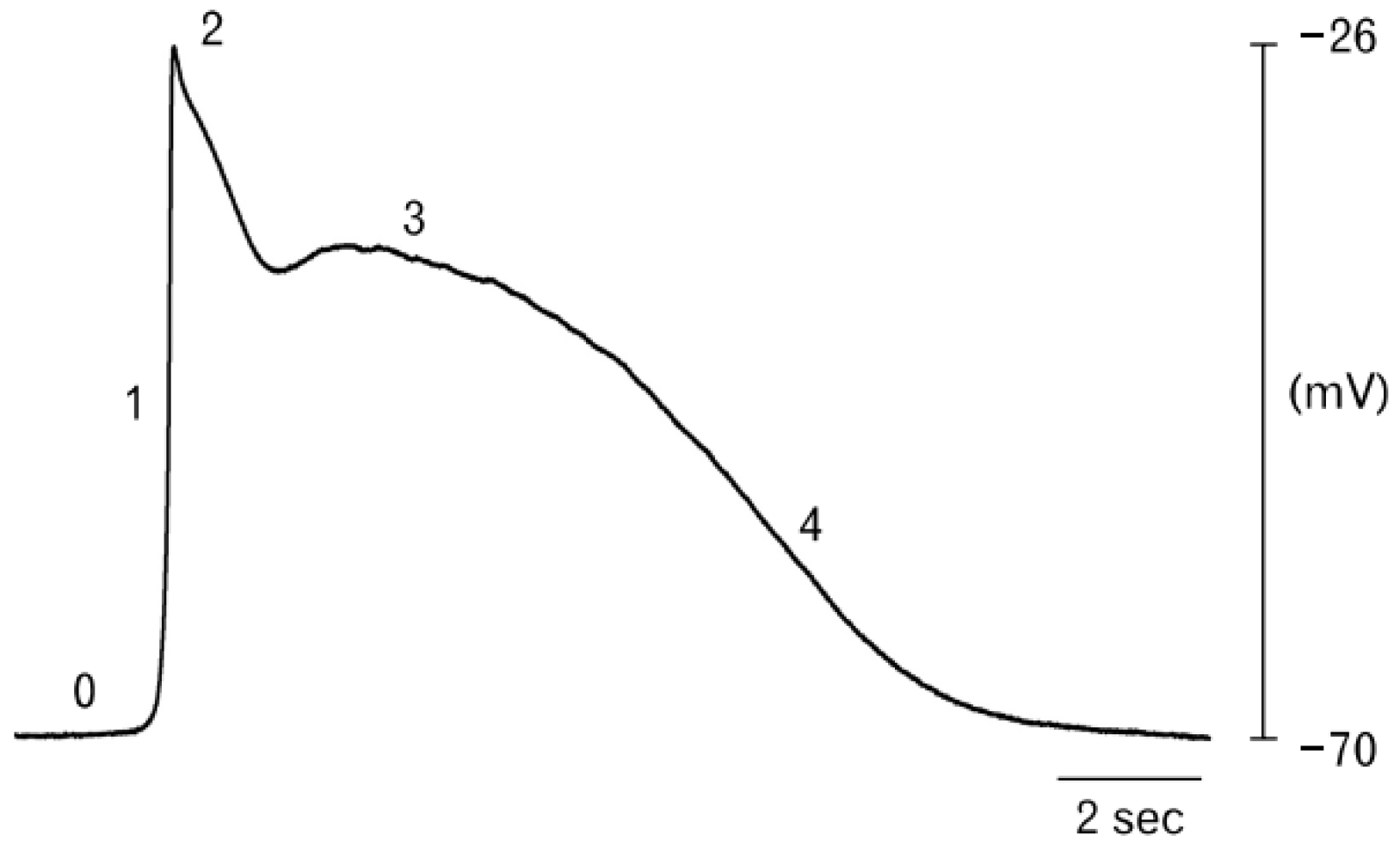
Fig. 2
Standard placement of electrogastrography electrodes. Four electrodes (A1, A2, A3, A4) are placed along the stomach's axis, with a reference electrode (R) near the xiphoid process and a ground electrode (U) on the left costal margin. Adapted from Komorowski et al.67 with permission from Springer Nature.
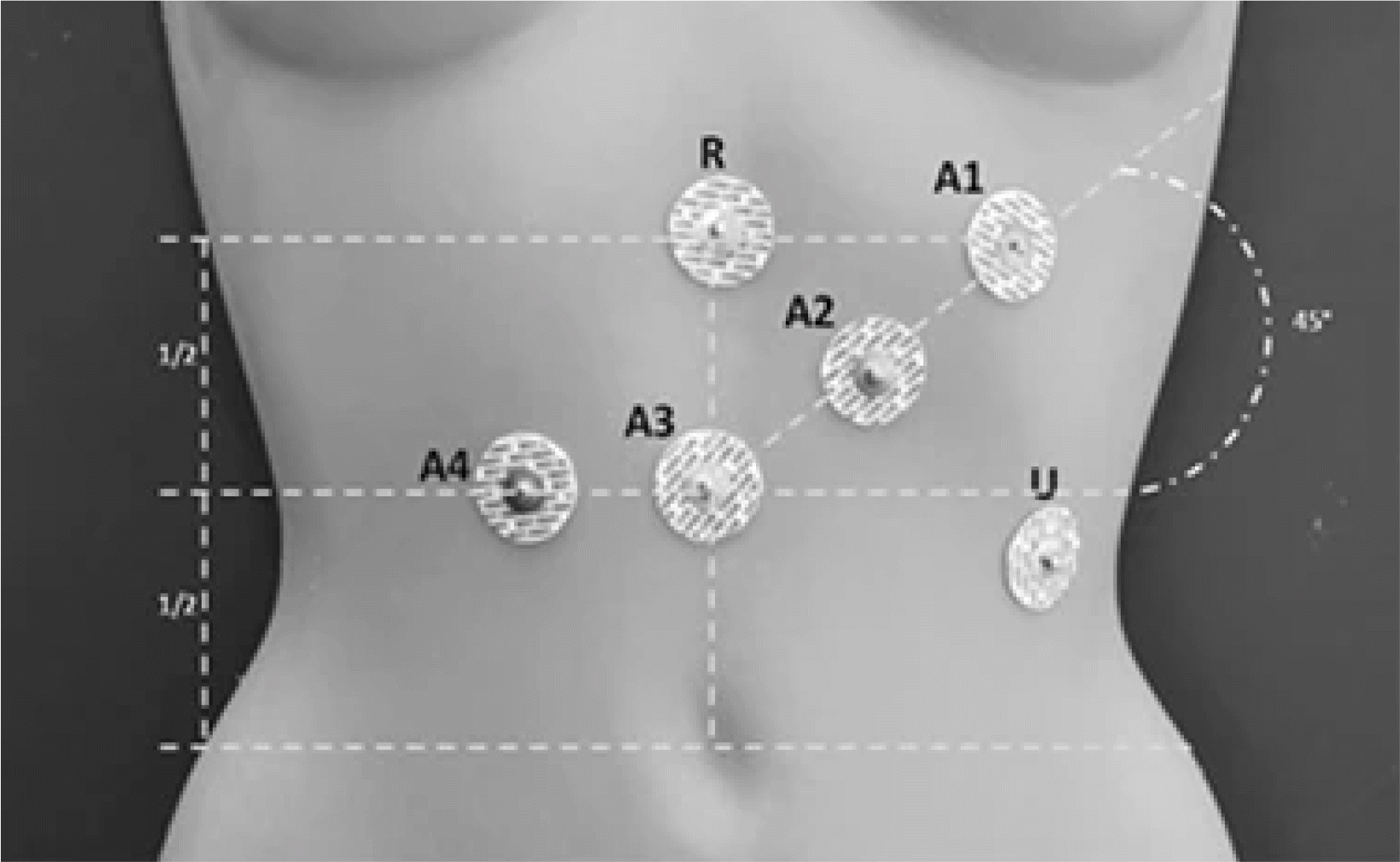
Fig. 3
High-resolution electrogastrography. Multi-electrode array is used to measure gastric electrical signals simultaneously at various locations on the stomach and represent the spatial propagation of gastric electrical activity. Adapted from Carson et al.68 with permission from Wiley.
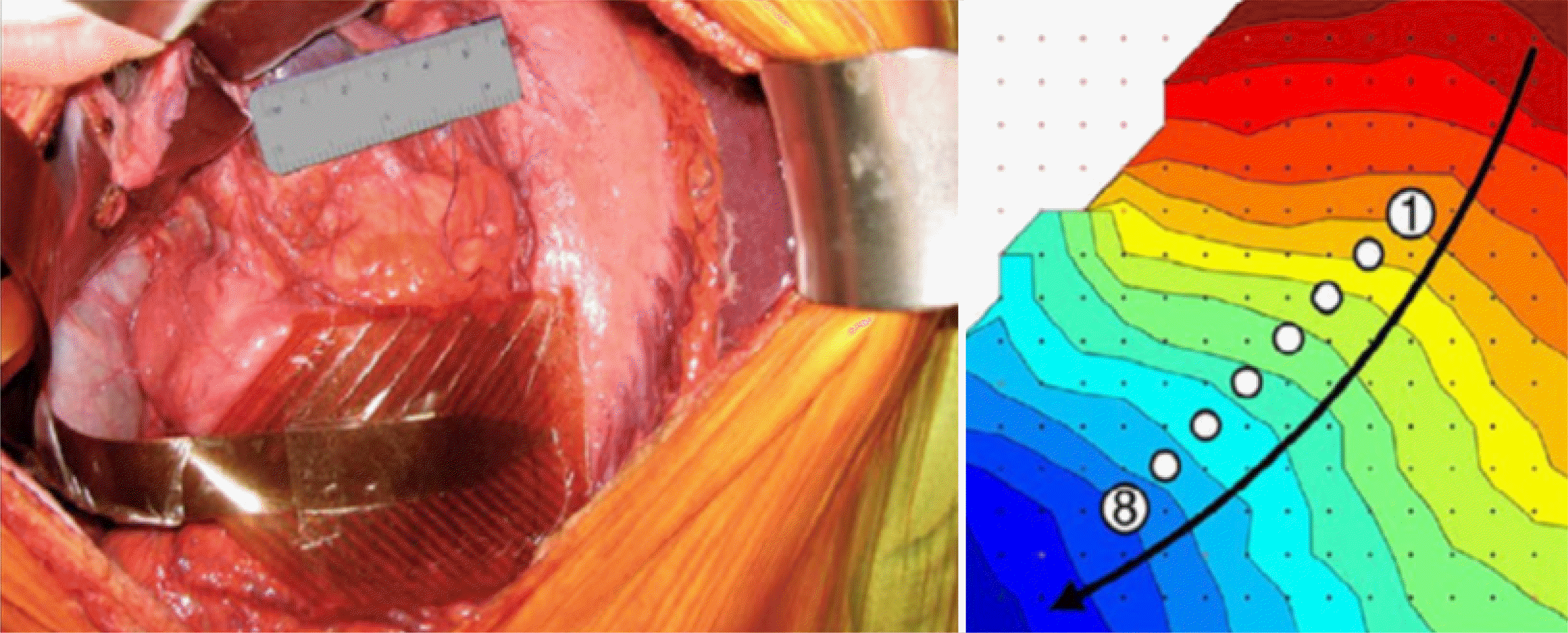
Fig. 4
Cutaneous high-resolution electrogastrography. Electrode array attached to the abdominal surface, and a circular diagram illustrating correlations between various variables. Adapted from Carson et al.68 with permission from Wiley.
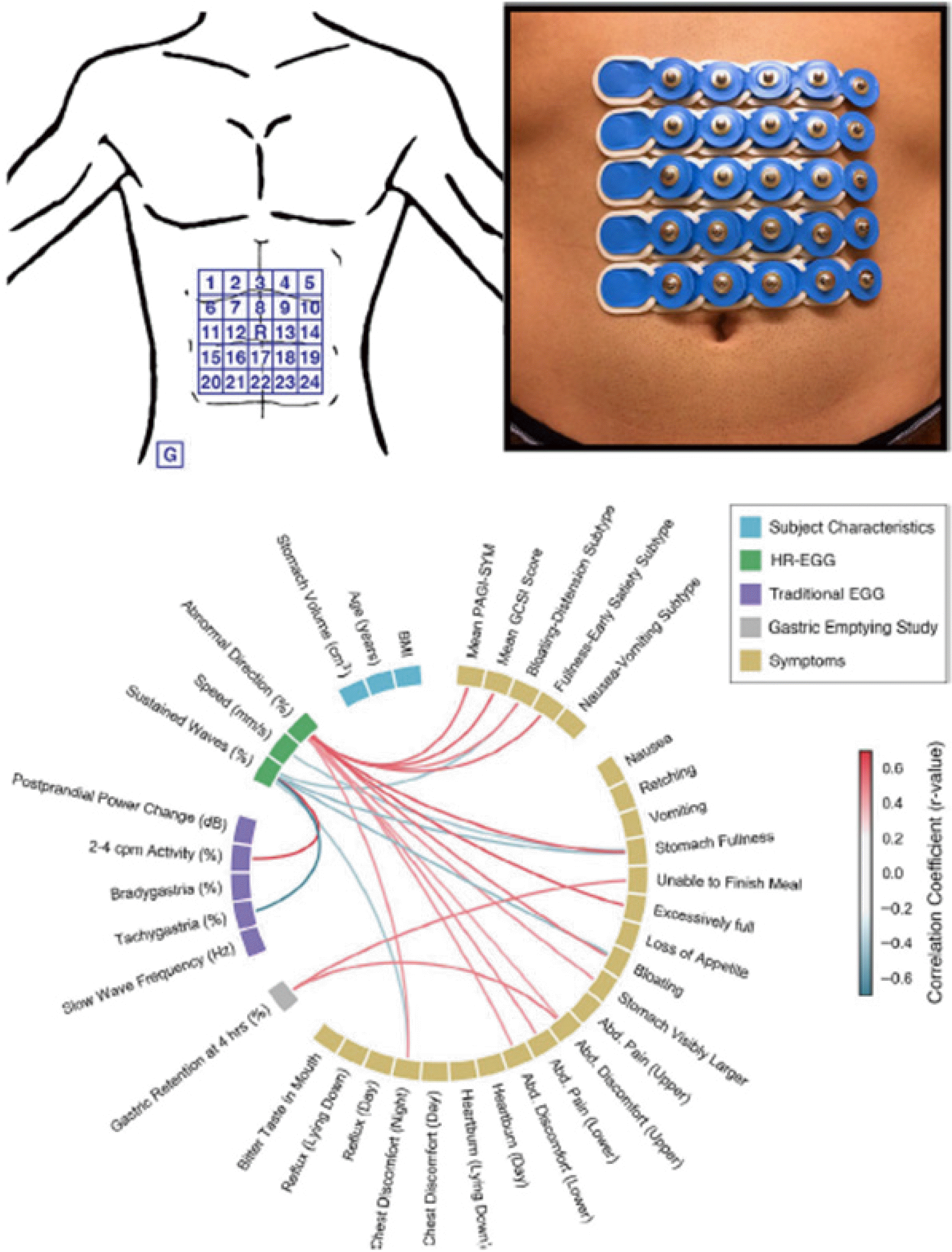