Dear Sir:
Intracranial atherosclerotic disease (ICAD) is a major cause of ischemic stroke. In patients with symptomatic ICAD, ICAD plaque ulceration as revealed through three-dimensional rotational angiography (3DRA) was associated with repetitive infarctions downstream, indicating possibly repetitive embolization [1]. Hence, plaque ulceration represents a vulnerable plaque type in ICAD, and it is imperative to study the contributing factors.
Previous studies have revealed the effects of carotid bifurcation geometry, in addition to systemic risk factors, on atherosclerosis in proximal internal carotid artery (ICA) [2,3]. Regarding ICAD, there is some evidence of the association between proximal artery geometry and early-stage atherosclerosis (e.g., non-stenotic plaque) in middle cerebral artery (MCA) [4]. However, it would be more clinically relevant to study such associations in patients with advanced ICAD (e.g., high-grade, symptomatic lesions), which bear a much higher stroke risk. In this study, we therefore aimed to investigate the association between terminal ICA bifurcation geometry and MCA-M1 plaque ulceration in patients with symptomatic, high-grade, atherosclerotic MCA-M1 stenosis.
In a prospective, cross-sectional study, patients with ischemic stroke or transient ischemic attack within 1 month, attributed to high-grade (60%–99%), atherosclerotic MCA-M1 stenosis confirmed through 3DRA, were recruited. Patients with an ipsilateral hypoplastic anterior cerebral artery (ACA) were excluded. More inclusion/exclusion criteria are provided in Supplementary Methods. This study was approved by the local Ethics Committee (Reference No. 2011.021), and all patients provided written informed consent.
Ulcerative MCA-M1 plaque was defined as the invagination of contrast beneath the endoluminal lining or a >90˚ slope at the upstream or downstream plaque shoulder [1]. Non-ulcerative plaques were defined as lesions with a smooth or irregular plaque surface [1]. We measured the distance of the plaque from the MCA origin and assessed the geometric features at the terminal ICA bifurcation in 3DRA, including the diameters at the MCA origin, ACA origin, and terminal ICA, and MCA-ACA angle (Figure 1 and Supplementary Methods). We calculated the area ratios of MCA versus ACA origin, MCA origin versus terminal ICA, and MCA+ACA origins versus terminal ICA.
Continuous and categorical variables were respectively described using median (interquartile range, IQR) and numbers (percentage) and compared between patients with ulcerative and non-ulcerative plaques, using Wilcoxon rank sum tests and chi-square or Fisher’s exact tests. The geometric features of ICA bifurcation with a P<0.05 in univariate analyses were analyzed using multivariate logistic regression for their independent associations with ulcerative MCA-M1 plaque, adjusting for age, sex in Model 1, plus cardiovascular risk factors in Model 2, and plus percent luminal stenosis in MCA-M1 in Model 3. All analyses were conducted using IBM SPSS Statistics v26.0 (IBM Corp., Armonk, NY, USA), with a two-sided P<0.05 considered to represent statistical significance.
A flowchart is provided in Supplementary Figure 1. Among the 154 patients (median age 59 years; 65.6% males) recruited, 50 (32.5%), 84 (54.5%), and 20 (13.0%), respectively, had smooth, irregular, and ulcerative MCA-M1 plaques. Of the 20 patients with ulcerative plaques, the ulceration was located at the proximal, middle, and distal one-thirds of the plaques in 7, 7, and 6 patients, respectively. The median MCA-M1 luminal stenosis was 75% (IQR 71%–84%). The age, sex, common cardiovascular risk factors, prior ischemic stroke or transient ischemic attack, fasting blood test results (glycosylated hemoglobin, glucose, lipids), and MCA-M1 luminal stenosis were similar between patients with ulcerative and non-ulcerative MCA-M1 plaques (Supplementary Table 1).
Diameters of terminal ICA (medians 3.3 vs. 3.3 mm), MCA origin (2.5 vs. 2.6 mm), and ACA origin (2.1 vs. 2.0 mm); (MCA+ ACA)/ICA area ratio (0.94 vs. 1.03); or distance of the plaque from MCA origin (4.4 vs. 4.7 mm) were not significantly different between patients with ulcerative and non-ulcerative MCA-M1 plaques (all P>0.05) (Supplementary Table 2). However, the MCA/ACA (1.36 vs. 1.71; P=0.007) and MCA/ICA area ratios (0.51 vs. 0.65; P=0.029), and MCA-ACA angle (103.1° vs. 113.2°; P=0.046) were smaller in patients with ulcerative MCA-M1 plaques than in those with non-ulcerative MCA-M1 plaques (Supplementary Table 2 and Supplementary Figure 2).
Smaller MCA/ACA and MCA/ICA area ratios were independently associated with ulcerative MCA-M1 plaques (P<0.05 in all multivariate logistic regression models) (Table 1). The association between a smaller MCA-ACA angle and ulcerative MCA-M1 plaque was attenuated in multivariate regression (0.05<P<0.1) (Table 1). Figure 1 illustrates two cases with ulcerative and non-ulcerative MCA-M1 plaques, with different geometric features at the terminal ICA bifurcation.
In summary, in this study, using 3DRA with a high spatial resolution for depicting intracranial arteries and ICAD lesions, we established an association between smaller MCA caliber relative to ACA and ICA and smaller MCA-ACA angle with MCA-M1 plaque ulceration, in patients with symptomatic, high-grade MCA-M1 stenosis, independent of systemic confounders and MCA-M1 luminal stenosis. These findings are partially consistent with a carotid bifurcation study, wherein smaller proximal ICA caliber relative to distal common carotid artery was associated with more severe ICA stenosis [2]. However, the same study also associated a larger carotid bifurcation angle with more severe ICA stenosis [2], contrary to our findings. The differences in the anatomical features at carotid bifurcation and distal ICA bifurcation may partly explain the inconsistent findings among these two studies and other previous studies when proximal ICA contains the bulb area (with focally enlarged vessel lumen) and the distal resistance in external carotid artery differs from ICA. Another reason could be the different stages of atherosclerosis investigated in individual studies. For instance, a larger bifurcation angle would generally increase the area of low and oscillatory wall shear stress (WSS), which is atherogenic in the early stages [5]. In contrast, with a sudden, significant decrease in the vessel caliber from distal ICA to proximal MCA and a smaller degree of deviation in the flow direction (i.e., smaller MCA-ACA angle), there could be relatively high flow velocities and velocity gradient and hence high WSS in MCA-M1. This may increase plaque vulnerability and lead to plaque rupture (i.e., ulceration) in advanced atherosclerosis, which could partly explain the findings in the current study. Indeed, in our previous studies using a computational fluid dynamics model to simulate blood flow and quantify WSS across symptomatic ICAD of 50%–99% stenosis based on computed tomography angiography, we did observe significant associations of elevated WSS with multiple cortical or territorial infarcts at the index stroke and a higher risk of recurrent stroke with similar infarct patterns. Such infarct patterns suggest artery-to-artery embolism as a stroke mechanism that may be caused by plaque rupture [6,7]. Another study using high-resolution vessel wall MRI to depict MCA plaque characteristics and four-dimensional MRI to quantify focal WSS corroborated our previous findings. It showed that plaque enhancement was associated with the highest WSS, located mostly at the upstream shoulder, in MCA plaques with downstream infarct patterns related to artery-to-artery embolism [8].
In conclusion, the geometry at the terminal ICA bifurcation was associated with MCA-M1 plaque ulceration, independent of cardiovascular risk factors and MCA-M1 luminal stenosis. This may help identify patients at a high risk of plaque ulceration. Further longitudinal, rheological studies across populations and subjects with different stages of ICAD may reveal the hemodynamic mechanisms and the possible effects on the risk of stroke recurrence. Vessel wall imaging for plaque components could also aid in understanding the mechanisms underlying such associations.
Supplementary materials
Supplementary materials related to this article can be found online at https://doi.org/10.5853/jos.2024.00129.
Supplementary Table 1.
Demographics, cardiovascular risk factors, and MCA-M1 luminal stenosis in patients with non-ulcerative versus ulcerative MCA-M1 plaques
Supplementary Table 2.
Geometric characteristics at terminal ICA bifurcation in patients with non-ulcerative versus ulcerative MCA-M1 plaques
Supplementary Figure 1.
Flowchart for patient screening. ICAD, intracranial atherosclerotic disease; 3DRA, three-dimensional rotational angiography; MCA, middle cerebral artery.
Supplementary Figure 2.
Scatterplots showing MCA/ACA and MCA/ICA area ratios and MCA-ACA angle in patients with non-ulcerative and ulcerative MCA-M1 plaques. Smaller MCA/ACA (medians 1.36 vs. 1.71; P=0.007) and MCA/ICA area ratios (0.51 vs. 0.65; P=0.029) and MCA-ACA angle (103.1° vs. 113.2°; P=0.046) were observed in patients with ulcerative MCA-M1 plaques than in those with non-ulcerative MCA-M1 plaques. MCA, middle cerebral artery; ACA, anterior cerebral artery; ICA, internal carotid artery.
Notes
ACKNOWLEDGMENTS
We thank the participants of the study, and all clinical and research staff who contributed to patient recruitment.
References
1. Leung TW, Wang L, Zou X, Soo Y, Pu Y, Ip HL, et al. Plaque morphology in acute symptomatic intracranial atherosclerotic disease. J Neurol Neurosurg Psychiatry. 2020; 92:370–376.


2. Phan TG, Beare RJ, Jolley D, Das G, Ren M, Wong K, et al. Carotid artery anatomy and geometry as risk factors for carotid atherosclerotic disease. Stroke. 2012; 43:1596–1601.


3. Jiang P, Chen Z, Hippe DS, Watase H, Sun B, Lin R, et al. Association between carotid bifurcation geometry and atherosclerotic plaque vulnerability: a Chinese atherosclerosis risk evaluation study. Arterioscler Thromb Vasc Biol. 2020; 40:1383–1391.
4. Liu YT, Zhang ZM, Li ML, Gao S, Feng F, Xu WH. Association of carotid artery geometries with middle cerebral artery atherosclerosis. Atherosclerosis. 2022; 352:27–34.


5. Spanos K, Petrocheilou G, Karathanos C, Labropoulos N, Mikhailidis D, Giannoukas A. Carotid bifurcation geometry and atherosclerosis. Angiology. 2017; 68:757–764.


6. Leng X, Lan L, Ip HL, Abrigo J, Scalzo F, Liu H, et al. Hemodynamics and stroke risk in intracranial atherosclerotic disease. Ann Neurol. 2019; 85:752–764.


7. Feng X, Fang H, Ip BYM, Chan KL, Li S, Tian X, et al. Cerebral hemodynamics underlying artery-to-artery embolism in symptomatic intracranial atherosclerotic disease. Transl Stroke Res. 2024; 15:572–579.


8. Woo HG, Kim HG, Lee KM, Ha SH, Jo H, Heo SH, et al. Wall shear stress associated with stroke occurrence and mechanisms in middle cerebral artery atherosclerosis. J Stroke. 2023; 25:132–140.
Figure 1.
Illustration of terminal ICA bifurcation geometry in 2 patients with ulcerative and non-ulcerative MCA-M1 plaques. (A) In a patient with an ulcerative (black arrow) MCA-M1 plaque of 83% luminal stenosis, the diameters of MCA origin, ACA origin, and terminal ICA were 2.04, 2.13, and 3.64 mm, respectively, and the MCA/ACA and MCA/ICA area ratios were 0.92 and 0.31, respectively, with an MCA-ACA angle of 87.7°. (B) In a patient with a non-ulcerative MCA-M1 plaque of 88% luminal stenosis, the diameters of MCA origin, ACA origin, and terminal ICA were 2.71, 2.20, and 3.25 mm, respectively, and the MCA/ACA and MCA/ICA area ratios were 1.52 and 0.70, respectively, with an MCA-ACA angle of 120.5°. ICA, internal carotid artery; MCA, middle cerebral artery; ACA, anterior cerebral artery.
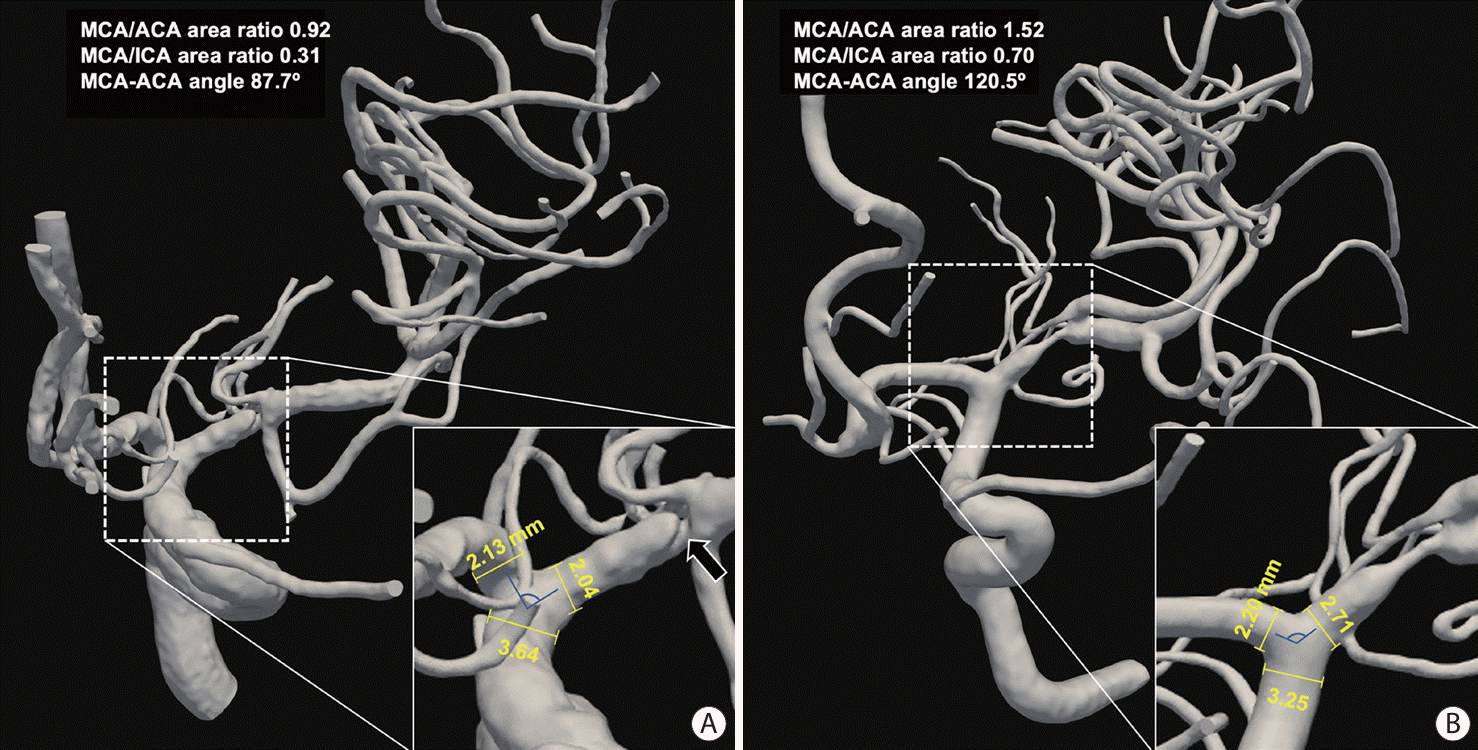
Table 1.
Multivariate logistic regression analyses of the independent associations between three geometric metrics and MCA-M1 plaque ulceration