Abstract
Background
Anesthetic agents are potential modifiable factors that can mitigate chronic postsurgical pain (CPSP) development. This study aimed to investigate the association between propofol-based total intravenous anesthesia (TIVA) and the occurrence of CPSP following video-assisted thoracoscopic surgery (VATS) for lung cancer resection.
Methods
This single-center retrospective cohort study included adult patients with lung cancer who underwent elective VATS between January 2018 and December 2022. Patients were divided based on the maintenance anesthetic used (propofol vs. sevoflurane). The primary outcome was the presence of CPSP, defined as any level of surgical site pain recorded within 3–6 months postoperatively. The authors investigated the association between anesthetic agents and CPSP using propensity score matching with stabilized inverse probability of treatment weighting (sIPTW) to adjust for confounders. Additionally, multivariable logistic regression was used to further adjust for intraoperative opioid use that sIPTW could not account for. The robustness of these associations was evaluated using the E-value.
Results
Of the 833 patients analyzed, 461 received propofol and 372 sevoflurane. The overall incidence of CPSP was 43.3%. After sIPTW, the use of TIVA was significantly associated with a lower incidence of CPSP (odds ratio [OR] 0.75, 95% confidence interval [CI] 0.57–0.99, P = 0.041), and remained significant after adjusting for intraoperative remifentanil equivalent dose (OR 0.73, 95% CI 0.55–0.96, P = 0.026). The E-values were 1.08 and 1.17, respectively.
Chronic postsurgical pain (CPSP) is a highly prevalent postoperative complication that profoundly affects patients' quality of life by causing functional and emotional disabilities and imposing substantial economic burdens [1,2]. Additionally, CPSP is associated with persistent opioid use, potentially contributing to the opioid epidemic [3]. Despite its significance, effective treatment modalities for CPSP remain elusive, highlighting the critical need for its prevention [4]. Thus, several preventive strategies have been proposed, including preemptive analgesia, regional analgesia, pharmacological interventions, psychoeducational support, and the modification of surgical techniques [5].
Anesthetic agents are potential modifiable factors that can mitigate the development of CPSP. In particular, propofol has been suggested to alleviate postoperative pain through positive modulation of inhibitory functions, inhibition of central sensitization, and antioxidant, anti-inflammatory, and nerve hyperexcitability inhibitory effects [6]. In previous studies in human volunteers, propofol administration reduced hyperalgesia and allodynia and diminished the anti-analgesic effects observed when remifentanil infusion was discontinued [7,8]. In contrast, another widely used group of anesthetics, the inhalational agents, may contribute to the development of CPSP due to their pronociceptive effect through the activation of peripheral ion channels transmitting nociceptive signals [9].
Video-assisted thoracoscopic surgery (VATS) has become the standard surgical approach for lung cancer owing to its minimally invasive nature and comparable oncological outcomes to those of open thoracotomy [10]. However, it remains controversial whether VATS can reduce CPSP compared to thoracotomy, despite its less invasive nature [11,12]. Moreover, the importance of CPSP in patients undergoing VATS is underscored by the high prevalence of lung cancer and the adoption of low-dose computed tomography for early detection [13]. To the best of the authors’ knowledge, no study to date has investigated the impact of anesthetic agents on CPSP in patients undergoing VATS.
Therefore, this study aimed to retrospectively investigate the association between the type of general anesthesia and the incidence of CPSP in patients who underwent VATS using propensity score analysis with inverse probability of treatment weighting (IPTW). Specifically, the authors sought to investigate the association between two major anesthetic techniques, namely propofol-based total intravenous anesthesia (TIVA) and sevoflurane-based anesthesia, and the incidence of CPSP after VATS for lung cancer.
This was a single-center, retrospective cohort study. This study was approved by the Institutional Review Board (IRB) of the Seoul National University Hospital (IRB No. H-2312-069-1491). The requirement for written informed consent from the patients was waived because no personalized data were included. This study was conducted in accordance with the Declaration of Helsinki and adhered to the Strengthening the Reporting of Observational Studies in Epidemiology (STROBE) reporting guidelines [14].
We retrospectively reviewed data from adult patients who were diagnosed with lung cancer and underwent elective VATS between 2018 and 2022. The study included patients who were assessed for surgical site pain in the outpatient clinic between 3 and 6 months after the initial elective VATS and who did not undergo any other thoracic surgery within 6 months after the index surgery. The following patients were excluded: those who received neoadjuvant or adjuvant chemotherapy or radiation therapy between 1 year before and 6 months after surgery; those who were included in the authors’ previous randomized controlled trials (RCTs) on multimodal analgesia [15,16]; those whose anesthesia was maintained with an agent other than sevoflurane or propofol; those who underwent thoracic epidural analgesia; those who received combined operation such as chest wall resection or sternotomy; and those who required reoperation after the index surgery.
The data utilized in this study were obtained through a retrospective review of electronic medical records using the Seoul National University Hospital Patient Research Environment (SUPREME). Demographic data was collected, such as age, sex, weight, height, body mass index (BMI), history of smoking, and American Society of Anesthesiologists (ASA) physical status. The preoperative data included history of analgesic and neuropsychiatric medication use and history of prior thoracic surgery on the same side. In the assessment of the history of analgesic use, tramadol, classified as a weak opioid, was treated as an opioid. In the perioperative phase, the authors collected the name of the surgery, type of anesthetic used, duration of anesthesia (hour), regional analgesia, and intraoperative opioids used. Regional analgesia encompassed the paravertebral block, intercostal nerve block, or wound infiltration. During the study period, intravenous (IV) fentanyl and remifentanil were the only opioids used for intraoperative analgesia at the authors’ institution. Referring to a previous study that converted two opioids into equivalent doses, the total amount was calculated by adding the respective amounts of both opioids administered [17]. This was then divided by the patient's weight and anesthesia duration to get the amount (µg) per minute and body weight. In addition, d the maximum pain intensity assessed using an 11-point numeric rating scale recorded on the day of discharge after surgery, postoperative day of chest tube removal, length of hospital stay, prescription of strong opioids 3 months after discharge from surgery, and records of surgical site pain assessed at the outpatient clinic. No imputation for missing values were performed, nor did the authors rectify any outliers for the variables analyzed.
No pre-medications are routinely administered at the authors’ institution. The attending anesthesiologists determined that the type of general anesthesia administered during the study period was either inhalational anesthesia or propofol-based TIVA. In patients undergoing inhalation anesthesia, a bolus dose of 1.5 to 2 mg/kg of propofol, 2 to 4 µg/kg of fentanyl or target-controlled infusion (TCI) of remifentanil, and 0.6 to 1 mg/kg of rocuronium was administered for anesthetic induction. In patients undergoing propofol-based TIVA, both propofol and remifentanil were administered via TCI for anesthesia induction, followed by a bolus of rocuronium. Anesthesia was maintained with sevoflurane or propofol according to the anesthetic technique employed, under the guidance of an electroencephalogram-based anesthetic depth monitor. Intraoperative analgesic control was achieved by titration of the remifentanil concentration or an additional injection of fentanyl. Based on the judgment of the attending anesthesiologists, IV ketorolac, nefopam, or acetaminophen was administered for postoperative analgesia prior to completion of the surgery.
Fentanyl-based intravenous patient-controlled analgesia (IV-PCA) was employed for postoperative analgesia. Since mid-2019, the authors’ institution has discontinued the use of basal infusion for IV-PCA [18]. Other protocols related to postoperative pain management were consistently applied to all patients included in this study. Water intake and ward ambulation were permitted 6 hours postoperatively, and an oral extended-release 75 mg tramadol/650 mg acetaminophen combination tablet or a 400 mg ibuprofen tablet was routinely administered upon the resumption of water intake. Additionally, IV fentanyl and oral oxycodone were administered as rescue analgesics.
The primary outcome measure was the presence of CPSP, which was assessed by reviewing outpatient electronic medical records between 3 and 6 months after surgery. A patient was considered to have CPSP if they complained of surgical site pain with any level of pain intensity at least once during the observational period. Patients were considered not to have CPSP if all outpatient records during the observational period indicated the absence of surgical site pain. If the surgical site pain was not assessed at any time point, the patient was excluded from the study.
Secondary outcomes included postoperative length of hospital stay and post-discharge opioid prescriptions in outpatient settings. Post-discharge opioid prescriptions were defined as outpatient prescriptions for morphine, oxycodone, hydromorphone, buprenorphine, fentanyl, tapentadol, or tramadol between 3 and 6 months postoperatively.
Continuous variables are presented as means with standard deviations or medians with interquartile ranges, depending on normality, which was evaluated using the Shapiro–Wilk test. Categorical variables are presented as frequencies with percentages. The main statistical approaches for evaluating the association between the type of anesthesia and the occurrence of CPSP included the following analyses.
First, to investigate the association between the type of anesthesia (propofol-based TIVA vs. sevoflurane) and the primary and secondary outcomes, a logistic regression was conducted with adjustment for confounders using stabilized IPTW (sIPTW). The propensity score for each patient was calculated using a logistic regression model that predicted the likelihood of receiving propofol-based TIVA vs. sevoflurane anesthesia, based on the following confounders: sex, age, BMI, ASA physical status, history of analgesic use, history of neuropsychiatric medication use, history of prior thoracic surgery on the same side, duration of anesthesia (hour), extent of surgery (non-anatomical resection such as wedge resection and metastatectomy, segmentectomy, lobectomy, and more extensive procedures such as sleeve lobectomy, bilobectomy, and pneumonectomy), regional analgesia, prolonged chest tube placement, and maximum pain score on discharge day. Prolonged chest tube placement was defined as maintaining the tube for ≥ 4 days postoperatively, based on a previous study that identified it as a risk factor for CPSP after thoracic surgery [19]. Due to significant imbalances between the two anesthesia types, the intraoperative remifentanil equivalent dose could not be included as a confounder in the sIPTW. The weights were calculated as 1/(probability of receiving TIVA) for patients who underwent TIVA and 1/(1-probability of receiving TIVA) for those who did not [20]. These weights were stabilized by multiplying by the proportion of patients in each respective group. Patients with a probability of 0 or 1 for receiving TIVA were excluded based on the positivity assumption. Extreme weights below the 1st percentile or above the 99th percentile were adjusted to the 1st and 99th percentile values, respectively [20]. The balance of variables between the two groups before and after sIPTW was assessed using the standardized mean difference (SMD), with an SMD < 0.10 for all covariates indicating well-balanced groups. Subsequently, a binary logistic regression and t-tests were conducted to investigate the association between TIVA and both primary and secondary outcomes before and after applying sIPTW. Additionally, to consider the imbalance in the intraoperative remifentanil equivalent dose during sIPTW, multivariable logistic regression was conducted, including both the type of anesthesia and a high-dose intraoperative remifentanil equivalent dose (≥ 0.2 µg/kg/min) after applying sIPTW. The cutoff value of high-dose remifentanil (≥ 0.2 µg/kg/min) was determined according to previous studies that reported an association between opioid-induced hyperalgesia and CPSP [21,22].
Second, a sensitivity analysis was performed using a multivariable binary logistic regression analysis for the occurrence of CPSP after VATS. This analysis incorporated the variables used in the propensity score calculation along with the type of anesthesia and high-dose intraoperative remifentanil equivalent dose (≥ 0.2 µg/kg/min). Variables that showed a P value of less than 0.2 in the univariable logistic regression analyses were selected for inclusion in the multivariable analyses. A multivariable regression model was constructed using the selected variables and optimized using backward stepwise selection. The variance inflation factor was used to assess multicollinearity among the variables included in the logistic regression analyses. The Hosmer–Lemeshow test was employed to assess the model's goodness of fit.
Third, interaction analysis was conducted between propofol and female sex, high-dose remifentanil (≥ 0.2 µg/kg/min), maximal pain intensity on the day of discharge, and attending surgeon to investigate how these variables influenced the association between propofol and the occurrence of CPSP.
Finally, to assess the potential effect of unmeasured confounders on the observed association, E-values were estimated. The E-value quantifies the minimum association strength that an unmeasured confounder would need with both treatment and outcome to entirely negate a specific observed association between the treatment and outcome, assuming that all measured covariates are accounted for [23].
Additionally, the authors aimed to investigate the selection bias caused by the exclusion of patients due to the absence of pain records. During the revision period, they conducted a comparative analysis of the baseline characteristics between patients who were excluded from the analysis due to the absence of pain records in the EMRs and those who were included. They also considered the potential influence of attending surgeons on the results, expecting variations in pain documentation at postoperative outpatient clinics.
In the statistical analysis, all tests were carried out using a two-tailed approach, and P values less than 0.05 were deemed to be statistically significant. All statistical analyses were performed using the R language and environment for statistical computing (ver. 4.3.3; R Foundation for Statistical Computing). Additional packages including ‘tableone’ (ver. 0.13.2) and ‘survey’ (4.2.1) were used for conducting sIPTW analysis [24,25], and ‘ggplot2’ (ver. 3.5.0) for generating figures.
Of the 2,068 eligible patients, 496 were excluded based on the exclusion criteria and 739 did not have an outpatient record of surgical site pain assessment. Finally, 833 were divided into two groups. Accordingly, 461 and 372 patients were assigned to the propofol and sevoflurane groups, respectively (Fig. 1).
The baseline characteristics and perioperative variables compared between patients included in the analysis and those without an outpatient record on surgical pain assessment are presented in Table 1. The proportion of excluded patients was lower in the propofol group, and there was a significant difference in the composition of attending surgeons. Additionally, the two groups showed differences in age, the extent of surgery, duration of anesthesia, use of intraoperative opioids, regional analgesia, and the timing of chest tube removal; however, the clinical significance of these differences was not substantial.
Table 2 presents the baseline characteristics and perioperative variables before and after sIPTW in the propofol and sevoflurane groups. After sIPTW, all variables except intraoperative opioids showed an SMD of less than 0.1, indicating that they were adequately balanced (Fig. 2). Table 3 shows the odds ratio (ORs) or mean differences between the two groups for primary and secondary outcomes before and after sIPTW. The incidence of CPSP in the total cohort was 43.3% (n = 361), with rates of 47.6% (n = 177) and 39.9% (n = 184) in the sevoflurane and propofol groups, respectively. After sIPTW, the use of propofol was significantly associated with a lower incidence of CPSP (OR: 0.75, 95% confidence interval [CI]: 0.57–0.99, P = 0.041). The E-value for this association was 1.08. After adjusting for the high-dose intraoperative remifentanil equivalent dose, propofol use was significantly associated with a lower incidence of CPSP (OR: 0.73, 95% CI: 0.55–0.96, P = 0.026). The E-value for this association was 1.17. No secondary outcomes showed statistically significant differences between the groups before and after the sIPTW.
In the sensitivity analysis, the final model of multivariable logistic regression indicated that female sex, longer duration of anesthesia, and higher pain scores at discharge were associated with increased risk of CPSP, while propofol-based TIVA (OR: 0.67, 95% CI: 0.50–0.89, P = 0.006) was associated with lower risk of CPSP (Table 4). The Hosmer–Lemeshow test indicated that the model showed no evidence of a lack of fit (χ2 = 5.196, P = 0.736). The E-value for the association between propofol-based TIVA and CPSP in this model was 1.31. Fig. 3 shows the results of different logistic regression models on the association between propofol-based TIVA and the risk of CPSP.
Interactions between propofol-based TIVA and female sex, high-dose remifentanil use, pain intensity on the day of discharge, and attending surgeon were not significant (Table 5).
This study investigated the association between anesthesia type and CPSP in patients who underwent VATS for lung cancer. The incidence of CPSP was significantly lower in the propofol group than in the sevoflurane group. However, the robustness of this association, assessed using the E-value, was not strong, indicating that caution is required when interpreting the results of this study.
Previous studies on the impact of the type of anesthesia on the occurrence of CPSP have reported conflicting results. In a RCT conducted in patients who underwent thoracotomy, the incidence of CPSP at 3 and 6 months postoperatively was significantly lower with propofol-based TIVA than with sevoflurane anesthesia [26]. In both an RCT conducted in patients undergoing open hysterectomy and a retrospective study in patients who underwent breast cancer surgery, propofol-based TIVA showed more favorable results in reducing the incidence of CPSP than those for sevoflurane anesthesia [27,28]. However, subsequent reports from a RCT conducted in patients who underwent cardiac surgery via sternotomy and a prospective cohort study in patients who underwent breast cancer surgery showed no significant difference in the incidence of CPSP between the propofol-based TIVA and inhalation anesthesia [29,30]. Furthermore, an RCT involving patients with preoperative chronic pain undergoing total knee arthroplasty revealed that, at 3 months postoperatively, the proportion of patients with no reported pain was higher among patients who were administered sevoflurane anesthesia compared to patients who received propofol-based TIVA [31]. Given the conflicting results, the impact of anesthetic choice on CPSP may not significantly influence the selection of general anesthetics. According to a survey of Australasian anesthesiologists regarding the choice between propofol-based TIVA and inhalation anesthesia, only 7.3% responded that they 'somewhat agree' or 'strongly agree' to the question of whether propofol-based TIVA reduces the risk of CPSP, whereas 73.5% stated that the risk of CPSP is identical for both propofol-based TIVA and inhalation anesthesia [32].
The main finding of this study was that propofol-based TIVA reduced the risk of developing CPSP. Given that general anesthesia is necessary for most VATS procedures, these findings could have a significant clinical impact on the incidence of CPSP. Surgical noxious stimuli and opioid use can contribute to postoperative hypersensitivity, increasing the risk of CPSP [33,34]. Although the administration of propofol during surgery lasts only a few hours, this period is characterized by the most intense noxious stimuli and the highest concentration of opioid administration. As a result, propofol could play a significant role in reducing CPSP by preventing hypersensitivity induced by noxious stimuli and opioids [35]. Consequently, the potential to influence long-term outcomes through anesthetic choice highlights the importance of propofol-based TIVA as a strategic approach for VATS procedures.
However, although the results showed a significant association between propofol-based TIVA and a reduction in the incidence of CPSP after VATS, even after adjusting for multiple confounders, the robustness of this association, as assessed by the E-value, was not substantial. Some confounders, such as preoperative psychological problems [36,37], perioperative analgesic method [38], and postoperative complications [39] that could not be adequately considered in this study owing to its retrospective nature, may have influenced the results. Therefore, prospective studies that account for all these confounding variables are needed to further investigate these associations.
The authors also explored the interactions between various risk factors and the use of propofol regarding the occurrence of CPSP and found no significant interactions, warranting further investigation. First, high doses of intraoperative remifentanil are associated with increased postoperative opioid use and a broader area of hyperalgesia [21]. This effect was evident in clinical studies of patients undergoing VATS surgery, where those who received remifentanil at doses above 0.2 µg/kg/min experienced more chronic pain after 1 year [22]. However, the present study did not find a significant association between high-dose remifentanil equivalents and the development of CPSP, possibly due to the interaction between high-dose remifentanil and different general anesthetics. In a previous RCT of patients with breast cancer, differences in postoperative pain between the sevoflurane and propofol groups were only observed under high-dose remifentanil, with notably more severe pain 24 hours after surgery in the group using high-dose remifentanil combined with sevoflurane [40]. Additionally, in the aforementioned RCT, while the difference in pain intensity between the two anesthetics was pronounced immediately after surgery, the pain had nearly disappeared by 24 hours postoperatively [40]. Therefore, the high intraoperative opioid use in the present study likely did not influence the occurrence of CPSP. Second, acute postoperative pain is a consistent risk factor for CPSP, and propofol administration can affect the intensity of acute postoperative pain. The authors explored the interaction between acute postoperative pain and propofol-based TIVA on CPSP occurrence but found no significant associations. Previous meta-analyses have shown that, although propofol-based TIVA reduces acute postoperative pain intensity significantly more than inhalational anesthesia, the reduction is clinically marginal, suggesting a minimal effect on the results of the present study [41,42]. Lastly, while female sex has been proposed as a significant risk factor for CPSP, the interaction between propofol-based TIVA and sex was not significant in this study. Previous studies on exclusively female patients, such as those with breast cancer and gynecological surgeries, have shown that propofol-based TIVA use is associated with a reduced incidence of CPSP [27,28]. However, to the authors’ knowledge, no previous study has investigated the effect of the type of anesthesia on CPSP in male patients alone. Further research is required to corroborate the findings of the present study.
Further limitations should be considered. First, the single-center, retrospective design of this study is susceptible to inherent biases, which represents a significant limitation. To mitigate the impact of potential biases, sIPTW and multivariable logistic regression analyses were conducted. However, it is possible that unmeasured confounding factors may still be present. The calculated E-value, used to assess the impact of unmeasured variables numerically, was insufficient to guarantee the robustness of the results. In addition, a significant number of patients were excluded due to the absence of pain documentation in the outpatient setting, which was taken exclusively by the attending surgeons. Consequently, the surgeons’ individual approaches could have introduced a selection bias, potentially affecting the study outcomes. Second, this study evaluated CPSP based on the presence of pain at the surgical site, as documented in the outpatient clinical records. This approach constrained the assessment of various pain characteristics, including pain intensity, temporal characteristics, referred pain, and neuropathic features. Nevertheless, the incidence of CPSP in the present study is similar to the results of prospective telephone interviews in the authors’ previous studies, supporting the reliability of this study’s findings [15,16]. Lastly, this study did not analyze postoperative analgesic use profiles. This was because the total amount of IV-PCA administered to each patient was not recorded, and the administration of rescue analgesics varied across the attending physicians. However, a recent meta-analysis reported that the impact of analgesics on CPSP is minimal and has uncertain clinical relevance [43], suggesting that the effect of this limitation on the outcomes of the present study would be minor. Instead, the pain intensity on the day of discharge was examined as an alternative measure for assessing the overall effectiveness of acute postoperative pain management.
In conclusion, in patients undergoing elective VATS for lung cancer, propofol-based TIVA was associated with a lower CPSP risk than that of sevoflurane anesthesia, between 3 and 6 months after surgery. This association was significant after adjusting for covariates related to CPSP. Nevertheless, considering the potential for inherent bias from the study design and the limited robustness of the results, further prospective studies are required to help anesthesiologists select the appropriate anesthetic for VATS where CPSP is prevalent.
Notes
DATA AVAILABILITY
The datasets generated and analyzed during the current study are available from the corresponding author upon reasonable request.
REFERENCES
1. Reddi D. 2016; Preventing chronic postoperative pain. Anaesthesia. 71(Suppl 1):64–71. DOI: 10.1111/anae.13306. PMID: 26620149.
2. Guertin JR, Pagé MG, Tarride JÉ, Talbot D, Watt-Watson J, Choinière M. 2018; Just how much does it cost? A cost study of chronic pain following cardiac surgery. J Pain Res. 11:2741–59. DOI: 10.2147/JPR.S175090. PMID: 30519078. PMCID: PMC6235323.
3. Glare P, Aubrey KR, Myles PS. 2019; Transition from acute to chronic pain after surgery. Lancet. 393:1537–46. DOI: 10.1016/S0140-6736(19)30352-6. PMID: 30983589.
4. Dworkin RH, McDermott MP, Raja SN. 2010; Preventing chronic postsurgical pain: how much of a difference makes a difference? Anesthesiology. 112:516–8. DOI: 10.1097/ALN.0b013e3181cf4253. PMID: 20124976.
5. Kim BR, Yoon SH, Lee HJ. 2023; Practical strategies for the prevention and management of chronic postsurgical pain. Korean J Pain. 36:149–62. DOI: 10.3344/kjp.23080. PMID: 36973967. PMCID: PMC10043790.
6. Irwin MG, Chung CKE, Ip KY, Wiles MD. 2020; Influence of propofol-based total intravenous anaesthesia on peri-operative outcome measures: a narrative review. Anaesthesia. 75 Suppl 1:e90–100. DOI: 10.1111/anae.14905. PMID: 31903578.
7. Bandschapp O, Filitz J, Ihmsen H, Berset A, Urwyler A, Koppert W, et al. 2010; Analgesic and antihyperalgesic properties of propofol in a human pain model. Anesthesiology. 113:421–8. DOI: 10.1097/ALN.0b013e3181e33ac8. PMID: 20613472.
8. Singler B, Tröster A, Manering N, Schüttler J, Koppert W. 2007; Modulation of remifentanil-induced postinfusion hyperalgesia by propofol. Anesth Analg. 104:1397–403. DOI: 10.1213/01.ane.0000261305.22324.f3. PMID: 17513631.
9. Matta JA, Cornett PM, Miyares RL, Abe K, Sahibzada N, Ahern GP. 2008; General anesthetics activate a nociceptive ion channel to enhance pain and inflammation. Proc Natl Acad Sci U S A. 105:8784–9. DOI: 10.1073/pnas.0711038105. PMID: 18574153. PMCID: PMC2438393.
10. Maiga AW, Deppen SA, Denton J, Matheny ME, Gillaspie EA, Nesbitt JC, et al. 2019; Uptake of video-assisted thoracoscopic lung resections within the veterans affairs for known or suspected lung cancer. JAMA Surg. 154:524–9. DOI: 10.1001/jamasurg.2019.0035. PMID: 30865221. PMCID: PMC6583397.
11. Bendixen M, Jørgensen OD, Kronborg C, Andersen C, Licht PB. 2016; Postoperative pain and quality of life after lobectomy via video-assisted thoracoscopic surgery or anterolateral thoracotomy for early stage lung cancer: a randomised controlled trial. Lancet Oncol. 17:836–44. DOI: 10.1016/S1470-2045(16)00173-X. PMID: 27160473.
12. Bayman EO, Parekh KR, Keech J, Selte A, Brennan TJ. 2017; A prospective study of chronic pain after thoracic surgery. Anesthesiology. 126:938–51. DOI: 10.1097/ALN.0000000000001576. PMID: 28248713. PMCID: PMC5395336.
13. Gierada DS, Black WC, Chiles C, Pinsky PF, Yankelevitz DF. 2020; Low-dose CT screening for lung cancer: evidence from 2 decades of study. Radiol Imaging Cancer. 2:e190058. DOI: 10.1148/rycan.2020190058. PMID: 32300760. PMCID: PMC7135238.
14. von Elm E, Altman DG, Egger M, Pocock SJ, Gøtzsche PC, Vandenbroucke JP. STROBE Initiative. 2007; The Strengthening the Reporting of Observational Studies in Epidemiology (STROBE) statement: guidelines for reporting observational studies. Ann Intern Med. 147:573–7. Erratum in: Ann Intern Med 2008; 148: 168.DOI: 10.7326/0003-4819-147-8-200710160-00010. PMID: 17938396.
15. Yoon S, Lee HB, Na KJ, Park S, Bahk J, Lee HJ. 2022; Effect of continuous infusion of intravenous nefopam on postoperative opioid consumption after video-assisted thoracic surgery: a double-blind randomized controlled trial. Pain Physician. 25:491–500. PMID: 36122258.
16. Lee HJ, Choi S, Yoon S, Yoon S, Bahk JH. 2024; Effect of an intravenous acetaminophen/ibuprofen fixed-dose combination on postoperative opioid consumption and pain after video-assisted thoracic surgery: a double-blind randomized controlled trial. Surg Endosc. 38:3061–9. DOI: 10.1007/s00464-024-10821-y. PMID: 38609589. PMCID: PMC11133211.
17. Maheshwari K, Avitsian R, Sessler DI, Makarova N, Tanios M, Raza S, et al. 2020; Multimodal analgesic regimen for spine surgery: a randomized placebo-controlled trial. Anesthesiology. 132:992–1002. DOI: 10.1097/ALN.0000000000003143. PMID: 32235144.
18. Jung H, Lee KH, Jeong Y, Lee KH, Yoon S, Kim WH, et al. 2020; Effect of fentanyl-based intravenous patient-controlled analgesia with and without basal infusion on postoperative opioid consumption and opioid-related side effects: a retrospective cohort study. J Pain Res. 13:3095–106. DOI: 10.2147/JPR.S281041. PMID: 33262644. PMCID: PMC7699445.
19. Peng Z, Li H, Zhang C, Qian X, Feng Z, Zhu S. 2014; A retrospective study of chronic post-surgical pain following thoracic surgery: prevalence, risk factors, incidence of neuropathic component, and impact on qualify of life. PLoS One. 9:e90014. DOI: 10.1371/journal.pone.0090014. PMID: 24587187. PMCID: PMC3938555.
20. Schulte PJ, Mascha EJ. 2018; Propensity score methods: theory and practice for anesthesia research. Anesth Analg. 127:1074–84. DOI: 10.1213/ANE.0000000000002920. PMID: 29750691.
21. Yu EH, Tran DH, Lam SW, Irwin MG. 2016; Remifentanil tolerance and hyperalgesia: short-term gain, long-term pain? Anaesthesia. 71:1347–62. DOI: 10.1111/anae.13602. PMID: 27734470.
22. Wang X, Ida M, Uyama K, Naito Y, Kawaguchi M. 2023; Impact of different doses of remifentanil on chronic postsurgical pain after video-assisted thoracic surgery: a propensity score analysis. Medicine (Baltimore). 102:e34442. DOI: 10.1097/MD.0000000000034442. PMID: 37505168. PMCID: PMC10378888.
23. VanderWeele TJ, Ding P. 2017; Sensitivity analysis in observational research: introducing the E-Value. Ann Intern Med. 167:268–74. DOI: 10.7326/M16-2607. PMID: 28693043.
24. Yoshida K, Bartel A. 2022; tableone: create 'Table 1' to describe baseline characteristics with or without propensity score weights [Internet]. R package version 0.13.2. https://CRAN.R-project.org/package=tableone.
25. Lumley T. 2022; Survey: analysis of complex survey samples [Internet]. R package version 4.2-1. http://cran.r-project.org/web/packages/survey/index.html.
26. Song JG, Shin JW, Lee EH, Choi DK, Bang JY, Chin JH, et al. 2012; Incidence of post-thoracotomy pain: a comparison between total intravenous anaesthesia and inhalation anaesthesia. Eur J Cardiothorac Surg. 41:1078–82. DOI: 10.1093/ejcts/ezr133. PMID: 22290901.
27. Cho AR, Kwon JY, Kim KH, Lee HJ, Kim HK, Kim ES, et al. 2013; The effects of anesthetics on chronic pain after breast cancer surgery. Anesth Analg. 116:685–93. DOI: 10.1213/ANE.0b013e31827ee372. PMID: 23400991.
28. Ogurlu M, Sari S, Küçük M, Bakis M, Ugur B, Eshraghi YE, et al. 2014; Comparison of the effect of propofol and sevoflurane anaesthesia on acute and chronic postoperative pain after hysterectomy. Anaesth Intensive Care. 42:365–70. DOI: 10.1177/0310057X1404200314. PMID: 24794477.
29. Yu H, Xu Z, Dai SH, Jiang JL, He LL, Zheng JQ, et al. 2021; The effect of propofol versus volatile anesthetics on persistent pain after cardiac surgery: a randomized controlled trial. J Cardiothorac Vasc Anesth. 35:2438–46. DOI: 10.1053/j.jvca.2020.10.025. PMID: 33183935.
30. Lefebvre-Kuntz D, Dualé C, Albi-Feldzer A, Nougarède B, Falewee MN, Ouchchane L, et al. 2015; General anaesthetic agents do not influence persistent pain after breast cancer surgery: a prospective nationwide cohort study. Eur J Anaesthesiol. 32:697–704. DOI: 10.1097/EJA.0000000000000215.
31. Yang R, Zhao D, Zhang XH, Liu RH, Xu GH, Shen QY. 2021; Comparison of sevoflurane and propofol on the incidence of postoperative pain and quality of life in patients undergoing total knee arthroplasty with chronic pain before surgery. Pain Pract. 21:37–44. DOI: 10.1111/papr.12931. PMID: 32615020.
32. Lim A, Braat S, Hiller J, Riedel B. 2018; Inhalational versus propofol-based total intravenous anaesthesia: practice patterns and perspectives among Australasian anaesthetists. Anaesth Intensive Care. 46:480–7. DOI: 10.1177/0310057X1804600509. PMID: 30189822.
33. Steyaert A, De Kock M. 2012; Chronic postsurgical pain. Curr Opin Anaesthesiol. 25:584–8. DOI: 10.1097/ACO.0b013e32835743b7. PMID: 22895123.
34. Colvin LA, Bull F, Hales TG. 2019; Perioperative opioid analgesia-when is enough too much? A review of opioid-induced tolerance and hyperalgesia. Lancet. 393:1558–68. DOI: 10.1016/S0140-6736(19)30430-1. PMID: 30983591.
35. Thapa P, Euasobhon P. 2018; Chronic postsurgical pain: current evidence for prevention and management. Korean J Pain. 31:155–73. DOI: 10.3344/kjp.2018.31.3.155. PMID: 30013730. PMCID: PMC6037807.
36. Theunissen M, Peters ML, Bruce J, Gramke HF, Marcus MA. 2012; Preoperative anxiety and catastrophizing: a systematic review and meta-analysis of the association with chronic postsurgical pain. Clin J Pain. 28:819–41. DOI: 10.1097/AJP.0b013e31824549d6. PMID: 22760489.
37. Chen D, Yang H, Yang L, Tang Y, Zeng H, He J, et al. 2024; Preoperative psychological symptoms and chronic postsurgical pain: analysis of the prospective China Surgery and Anaesthesia Cohort study. Br J Anaesth. 132:359–71. DOI: 10.1016/j.bja.2023.10.015. PMID: 37953200.
38. Clarke H, Poon M, Weinrib A, Katznelson R, Wentlandt K, Katz J. 2015; Preventive analgesia and novel strategies for the prevention of chronic post-surgical pain. Drugs. 75:339–51. DOI: 10.1007/s40265-015-0365-2. PMID: 25752774.
39. Willingham M, Rangrass G, Curcuru C, Ben Abdallah A, Wildes TS, McKinnon S, et al. 2020; Association between postoperative complications and lingering post-surgical pain: an observational cohort study. Br J Anaesth. 124:214–21. DOI: 10.1016/j.bja.2019.10.012. PMID: 31771788.
40. Shin SW, Cho AR, Lee HJ, Kim HJ, Byeon GJ, Yoon JW, et al. 2010; Maintenance anaesthetics during remifentanil-based anaesthesia might affect postoperative pain control after breast cancer surgery. Br J Anaesth. 105:661–7. DOI: 10.1093/bja/aeq257. PMID: 20876698.
41. Qiu Q, Choi SW, Wong SS, Irwin MG, Cheung CW. 2016; Effects of intra-operative maintenance of general anaesthesia with propofol on postoperative pain outcomes - a systematic review and meta-analysis. Anaesthesia. 71:1222–33. DOI: 10.1111/anae.13578. PMID: 27506326.
42. Peng K, Liu HY, Wu SR, Liu H, Zhang ZC, Ji FH. 2016; Does propofol anesthesia lead to less postoperative pain compared with inhalational anesthesia? A systematic review and meta-analysis. Anesth Analg. 123:846–58. DOI: 10.1213/ANE.0000000000001504. PMID: 27636574.
43. Carley ME, Chaparro LE, Choinière M, Kehlet H, Moore RA, Van Den Kerkhof E, et al. 2021; Pharmacotherapy for the prevention of chronic pain after surgery in adults: an updated systematic review and meta-analysis. Anesthesiology. 135:304–25. DOI: 10.1097/ALN.0000000000003837. PMID: 34237128.
Fig. 2
Covariate balance plot of the distribution of standardized mean differences of covariates between the two groups before and after stabilized inverse probability of treatment weighting. ASA: American Society of Anesthesiologists, NP: neuropsychiatric, POD: postoperative day, BMI: body mass index.
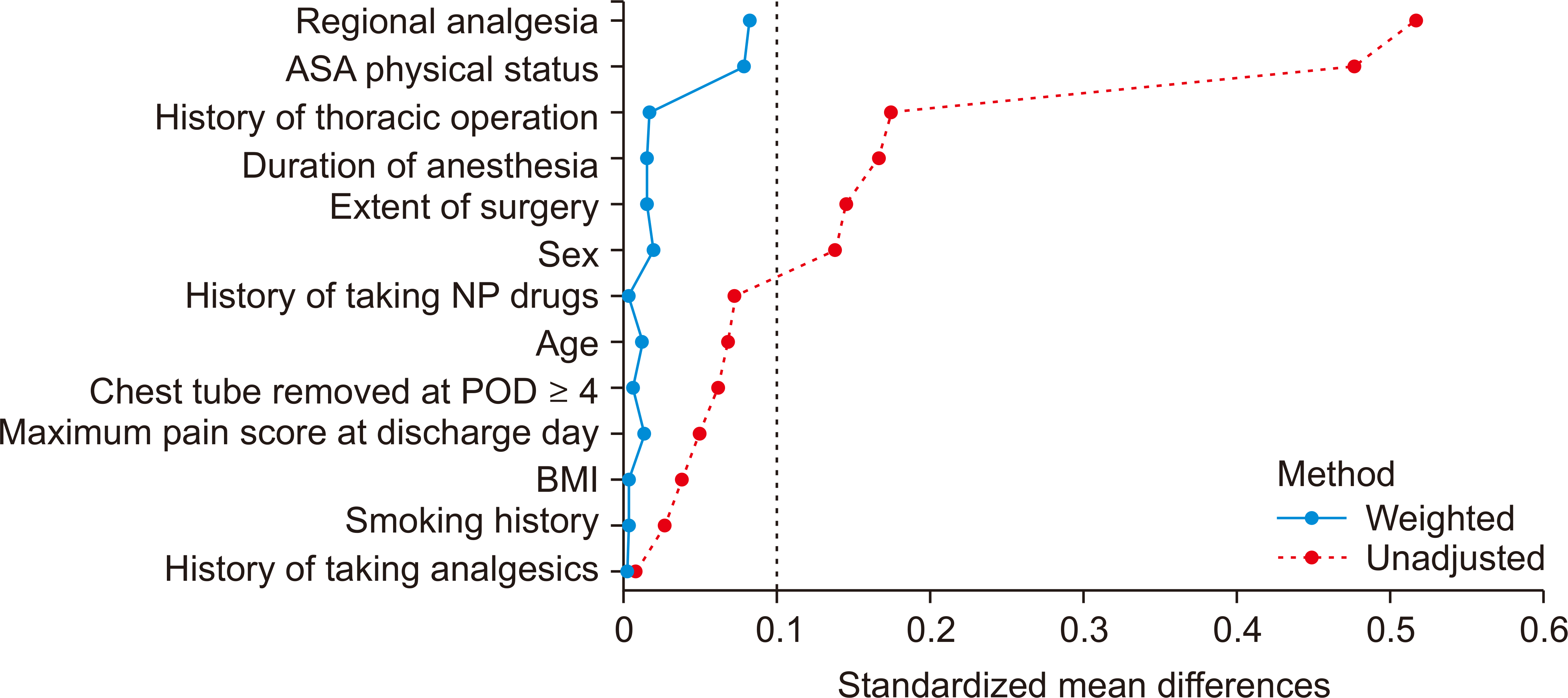
Fig. 3
Results from different logistic models on the association between propofol-based total intravenous anesthesia and risk of chronic postsurgical pain. sIPTW: stabilized inverse probability of treatment weighting, CI: confidence interval.
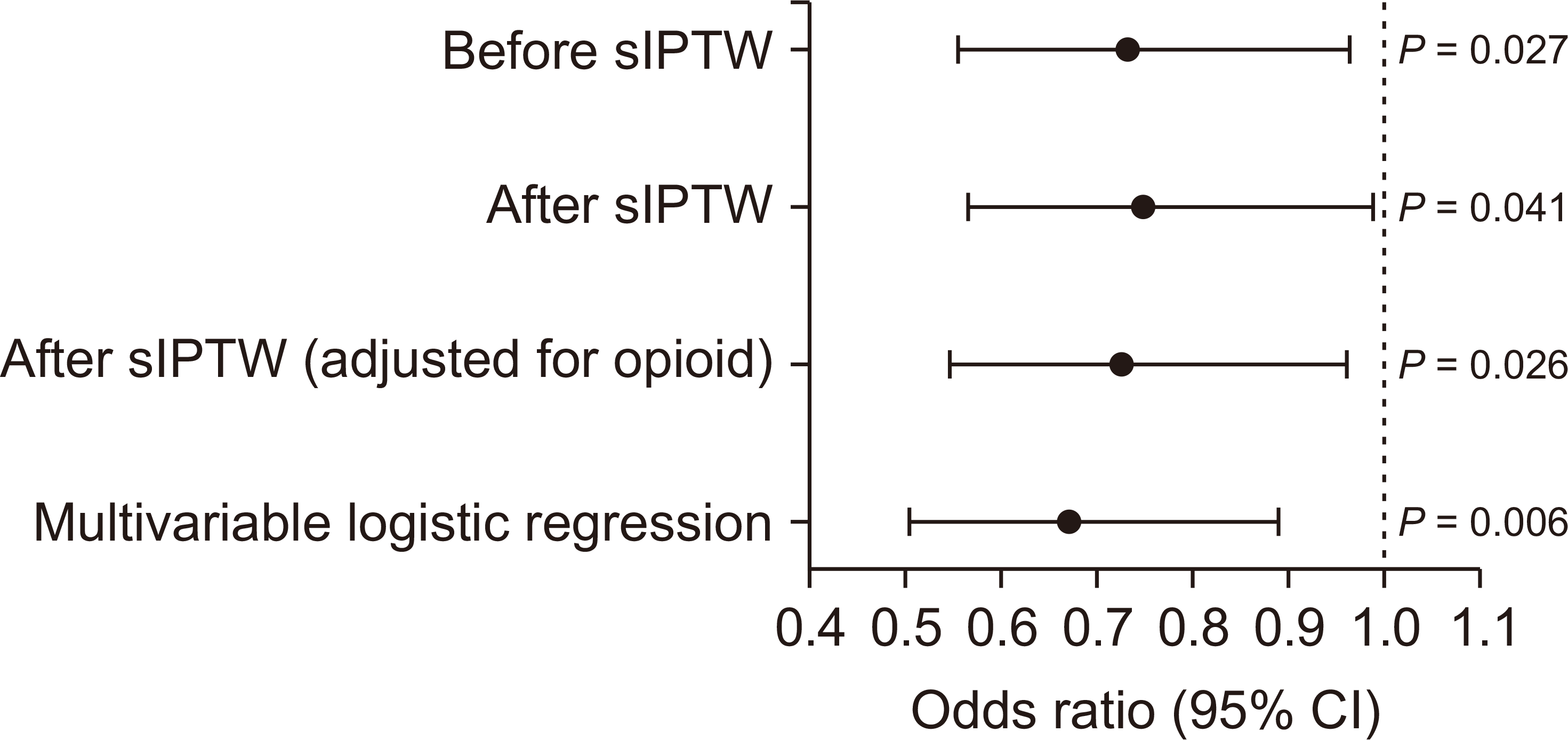
Table 1
Comparison of baseline characteristics and perioperative variables between patients included in the analysis and those without an outpatient record on surgical pain assessment
Table 2
Baseline characteristics compared between two groups before and after stabilized inverse probability of treatment weighting
Table 3
Comparison of primary and secondary outcomes before and after stabilized inverse probability of treatment weighting
Table 4
Results of the univariable and multivariable logistic regression analysis for chronic postsurgical pain
Table 5
Interactions between propofol-based total intravenous anesthesia and selected variables