Abstract
Nerve growth factor (NGF) is a neurotrophic protein that has crucial roles in survival, growth and differentiation. It is expressed in neuronal and non-neuronal tissues. NGF exerts its effects via two types of receptors including the high affinity receptor, tropomyosin receptor kinase A and the low affinity receptor p75 neurotrophin receptor highlighting the complex signaling pathways that underlie the roles of NGF. In pain perception and transmission, multiple studies shed light on the effects of NGF on different types of pain including inflammatory, neuropathic, cancer and visceral pain. Also, the binding of NGF to its receptors increases the availability of many nociceptive receptors such as transient receptor potential vanilloid 1, transient receptor potential ankyrin 1, N-methyl-D-aspartic acid, and P2X purinoceptor 3 as well as nociceptive transmitters such as substance P and calcitonin gene-related peptide. The role of NGF in pain has been documented in pre-clinical and clinical studies. This review aims to shed light on the role of NGF and its signaling in different types of pain.
In the early 1950's, NGF was discovered by Rita Levi-Montalcini in a tumor tissue [1]. The discovery of NGF was considered a landmark achievement in neurobiology. Since then, the diverse roles of NGF were determined in multiple era including the pain field. NGF belongs to a large family of proteins that include, in mammals, neurotrophin-3, neurotrophin-4/5 and brain-derived neurotrophic factor [2]. NGF is important for the survival, growth and differentiation of sympathetic and sensory afferent neurons during development and in the modulation of nociception in adulthood [1]. It is expressed in neuronal and non-neuronal tissues. In more detail, it is widely expressed in the central nervous cells, peripheral Schwann cells, glands, endothelial cells, immune cells and skeletal muscles [3]. Notably, there is shift in the role of NGF from neuronal growth to the regulation of the sensitivity of the peripheral nervous system to noxious stimuli [4]. NGF has two receptors including the high affinity receptor, tropomyosin receptor kinase A (TrkA) and the low affinity receptor p75 neurotrophin receptor (p75NTR) [5]. Through its interaction with the high-affinity receptor TrkA, NGF suppresses the synthesis of p75. This neurotrophic protein is produced as pro-NGF that has more affinity to p75NTR compared to NGF that binds with higher affinity to TrkA [5]. TrkA receptor is highly expressed in dorsal root ganglion (DRG) neurons during embryogenesis and its role declines post-natally [6]. Accordingly, NGF is a major contributor to pain signaling and transmission [2,7]. In this regard, it was found that rats develop hyperalgesia when degradation of NGF is blocked by matrix metalloproteinases-2 inhibitor through a peripheral mechanism [8]. The role of NGF in neuronal development and the perception of localized tissue pain has been highlighted in multiple studies [2]. Also, investigators found that increased NGF levels are linked to inflammation, persistent pain, injuries, and discomfort [7]. To add, multiple studies showed that NGF is a marker for the peptidergic nociceptors that express TrkA compared to non-peptidergic nociceptors that express glial cell line-derived neurotrophic factor [9]. Furthermore, several lines of evidences implicated the role of NGF in different types of pain such as inflammatory, neuropathic, cancer, orofacial, musculoskeletal, and low back pain (LBP) [10–13]. In fact, earlier reports pointed to the critical role of NGF-mediated signaling in the initiation and maintenance of chronic pain [2]. Thus, this review is written to shed light on the role of NGF and its signaling in different types of pain.
NGF plays a key role in nociception through several mechanisms including the release of inflammatory mediators, increase in the activity and availability of many nociceptive ion channels and receptors [14]. The binding of NGF to TrkA increases the availability of nociceptive receptors such as transient receptor potential vanilloid 1 (TRPV1), transient receptor potential ankyrin 1 (TRPA1), N-methyl-D-aspartic acid, and P2X purinoceptor 3 as well as nociceptive transmitters such as substance P and calcitonin gene-related peptide (CGRP) [15,16]. Thus, NGF plays a crucial role in peripheral and central sensitization. It sensitizes nociceptors directly in the short term and/or changes gene expression in the long term [2]. Earlier reports showed that NGF binds to TrkA receptor and participates in the initiation and maintenance of nociception through several intracellular signaling pathways, including mitogen-activated protein kinases (MAPKs)/extracellular signal-regulated kinases and phosphoinositide-3-kinase(PI3K) [14]. Furthermore, NGF increases the release of serotonin, histamine, prostaglandin E2, and NGF itself, that collectively binds to their receptors on the peripheral terminals of nociceptors [17]. Also, it sets off a cascade of events that cause nociceptive pain. Furthermore, TrkA binds NGF which is then retrogradely delivered to the cell bodies of neurons. Within these cell bodies, it regulates the expression of TRPV1, bradykinin receptors, voltage-gated sodium channels, acid sensing ion channel 2/3, and other receptors [18]. The nociceptive receptors on the surface of these cells that are involved in nociception trigger peripheral sensitization and increase pain perception [19]. Meanwhile, central sensitization is produced by changes in gene expression induced by NGF-TrkA signaling [2]. In conclusion, NGF plays a key role in pain perception by regulating the expression of genes related to pain, controlling the activity of key channels/receptors in pain and increasing the pro-inflammatory mediators (Fig. 1).
OA is an age-related chronic joint disease that leads to cartilage destruction caused by several factors including inflammation [20]. Inflammation is correlated to the role of NGF in increasing the sensitivity of nociceptive neurons in the area, thus regulating the production of pain molecules centrally and peripherally [10,11]. In more detail, it is well-known that pro-NGF controls both inflammation and apoptosis [21]. Further, neuropeptides, neurotransmitters, and immune-active cytokines are all produced due to NGF release and inflammation [11]. Also, the innate and adaptive immune responses are directly impacted by NGF, since it interacts with several cells that are involved in the immunological response, such as macrophages, lymphocytes, and mast cells [3]. Depending on the presence or absence of its receptors, NGF may promote or reduce inflammation. Accordingly, it is considered an endogenous molecule that serves two separate purposes initiating pathways that regulate inflammation/restrict tissue damage and stimulating immune responses. NGF has a role in the treatment of chronic inflammatory illnesses as it increases the pain that is associated with neurogenic inflammation of tissues [18]. In this regard, several lines of evidences highlighted the key roles of NGF in OA albeit controversy in its role as a pro-inflammatory [22] or anti-inflammatory mediator [23]. The following paragraphs are details about the involvement of NGF in inflammatory pain. For instance, it was found that NGF receptors are expressed by joint cells [24]. Also, a large body of evidences showed that the lack of NGF leads to the loss of sympathetic and sensory neurons similar to TrkA-knocking down in animals [6,25]. People with inflammatory or degenerative rheumatic illnesses, including rheumatoid arthritis, OA, and spondyloarthritis, have elevated levels of NGF in their synovial fluid. Further, a meta-analysis of thirteen placebo-controlled trials examining OA of the hip or knee was carried out by Schnitzer and Marks, 2015 [26]. The researchers discovered that blocking NGF significantly reduced pain when compared to a placebo. In a mouse model of medial meniscus destabilization (MMD) associated with OA, the effects of NGF and its soluble receptor, TrkAd5, on pain rating were examined. The study demonstrated that TrkAd5 effectively decreased discomfort in mice with OA [27]. In 2015, it was found that canine-specific anti-NGF mAb could cure degenerative joint disease in dogs [28,29].
Investigators tested the efficacy of several intra-articular and intraperitoneal injection of an anti-NGF into the knees of rats and reported the effectiveness in reversing OA-induced pain [30]. Also, Dakin et al. [31] revealed that blocking NGF reduced pain behavior in two OA rat models. In addition, the effects of tanezumab on weight-bearing and cartilage degradation were shown in a study using the rat medial meniscal tear model whereby rats with meniscal injury had a less aberrant gait after receiving tanezumab treatment, regardless of dosage and increase in the incidence of subchondral bone sclerosis and cartilage degradation [32]. According to Xu et al. [33], it was revealed that anti-NGF mAb reduced the severity and discomfort of OA but increased cartilage destruction compared to the control group. This effect was particularly seen in the early stages of the disease [33]. After joint or orthopaedic surgery, Majuta et al. [34] showed that using anti-NGF in the knee enhanced limb performance in a mouse model and reported that NGF has two distinct roles in development and maturity [34].
After injecting NGF antibody in the knee, it was revealed that there was a shift in the levels of CGRP in DRGs, a matter that causes interference with walking patterns [34]. Furthermore, a new NGF vaccination was effective in reducing the spontaneous pain behaviour in mice with surgically induced OA. Overall, these findings support the idea that inhibiting NGF signaling might be beneficial in alleviating chronic pain, particularly in OA patients [35]. Additionally, previous studies showed that NGF decelerated chondrocyte differentiation and improved ligament healing [36,37]. In a study conducted on chondrocytes, the authors reported that the mechanical loading of cartilage accompanied with an increase of visfatin/nicotinamide phosphoribosyltransferase and interleukin 1β (IL-1β) aggravated OA pain though the stimulation of NGF expression and release by these cells [38]. Also, it was reported that there was a decrease in patient's OA symptoms including pain when anti-NGF-treatment was used [39].
Lane and Corr [40] discussed the efficacy of NGF inhibitors in reducing musculoskeletal pain. Additionally, Wise et al. [13] reviewed the clinical studies evaluating NGF inhibitors' efficacy, such as tanezumab, in chronic pain states like OA pain and discussed the significant pain relief observed in clinical trials and the associated risks of rapidly progressive OA. Meng et al. [41] reviewed the efficacy of NGF inhibitors in the treatment of OA, while Pallav et al. [42] published that anti-NGF mAbs (tanezumab, fasinumab, and fulranumab) caused a significant decrease in pain and physical function scores compared to the control groups. These studies collectively underscore the therapeutic potential of NGF inhibitors in managing chronic musculoskeletal pain.
LBP is a type of pain that imposes huge burdens in the society. In 2007, the US estimated that 100–200 billion dollars are lost every year due to loss of productivity of employees or absence from work caused by LBP. Thus, various studies were conducted to find treatments for LBP. Nonradicular LBP is one kind of musculoskeletal pain that tanezumab showed promise in treatment. Anti-NGF showed low to moderate effects in alleviating LBP in patients [43]. In this regard, Markman et al. [44] discussed the safety and efficacy of tanezumab in treating nonradicular LBP that persists after medication. Another meta-analysis study revealed that the use of NGF agents improved the symptoms of LBP patients [45]. Researchers compared 5 or 10 mg of tanezumab administered subcutaneously every 8 weeks against 100 to 300 mg of tramadol taken orally once in a day. In contrast to tramadol, tanezumab at a dosage of 10 mg reduced the severity of LBP after 16 weeks. Complete joint replacement surgery was necessary for seven patients (1.4% of the total) in the 10 mg group compared to other groups. Tanezumab seems to be effective for chronic LBP [46]. In a study conducted in patients with chronic LBP, it was reported that tanezumab provided more improvement in pain and global assessment scores compared to the groups that received a placebo and naproxen [47]. On the other hand, other studies demonstrated the other side of NGF effects. In these studies, NGF was used to create a model of LBP in rats associated with mechanical somatosensory changes [48].
Over 60 years ago, during a transplantation experiment with a malignant mouse sarcoma, NGF was first discovered by Levi-Montalcini indicating the role of NGF in cancer. NGF alone cannot promote the growth of neoplasms cells. Indeed, it plays a substantial role in the development of neoplasms when expressed in conjunction with substances that promote neoplasms. Several lines of evidence indicate the key role of NGF in cancer. For instance, sortilin, a membrane receptor, has been associated with carcinogenesis by acting as a co-receptor for proNGF in cooperation with p75NTR to promote cancer cell invasion [10]. Further, neuropilin-1, found in many nociceptors, is considered a key player in the TrkA-mediated pain signaling cascade [49]. In the pancreatic ductal adenocarcinoma model, it was found that there is a reduction in TRPA1 transcription after treatment with NGF inhibitor [50].
The role of TrkA and/or p75NTR receptors in enhancing pro-survival signaling in tumors vary [51]. In breast cancer, NGF plays an important role by promoting cell proliferation via TrkA and cell survival via p75NTR [51]. Furthermore, earlier studies revealed that the activation of p75NTR enhances the ability of breast cancer cells to withstand chemotherapy-induced cell death [52]. Stimulating the Ras (rat sarcoma) pathways, which are mediated by TrkA, allows cells to survive and nerve fibers to proliferate. Also, this pathway was implicated in the TrkA-activated PI3Ks pathway leading to proliferation, invasion, and metastasis [21]. In prostate cells; it was found that p75NTR promotes cell death and inhibits tumor growth in normal prostate cells [21]. Further, there is strong evidence that NGF affects liver cancer progression and metastasis [53]. NGF modulates signaling pathways linked to cell migration, cytoskeleton structure, and liver cancer cell polarity [53]. At high NGF levels, cells are shielded from planned cell death and detachment-induced cell death, and undergo cellular metamorphosis, which is characterized by increased mobility and susceptibility to directional and structural alterations [53]. Furthermore, the use of anti-NGF mAbs has been explored in many preclinical trials as a potential remedy for cancer-related discomfort [54]. Scientists showed that, in contrast to conventional morphine treatment, a new NGF sequestering antibody significantly reduced pain-related behaviors in a bone cancer mice model [55]. It was revealed that anti-NGF treatment considerably mitigated functional connectivity alterations and reduced cancer [56]. Another study highlighted the connections between oral cancer pain, proliferation, cachexia, and NGF. Also, it was shown that cancer pain reduced when anti-NGF medicine was administered at the early and late stages of the disease, according to Jimenez-Andrade et al. [56]. In a mouse model of cancer-induced bone pain, Guedon et al. [57] investigated the effects of anti-NGF and found that anti-NGF mAbs reduced skeletal pain-related behaviors and affected cutaneous hypersensitivity.
According to the International Association for the Study of Pain: neuropathic pain is "pain caused by a lesion or disease of the somatosensory system" regardless of the cause [58]. The number of people suffering from neuropathic pain reached two million people according to US estimates [59]. In animal models of neuropathic pain, allodynia and thermal hyperalgesia were reduced by the intrathecal administration of NGF [60]. Meanwhile, in human clinical trials of peripheral neuropathic pain, NGF showed positive effects on neuropathic pain and pain sensitivity in human immunodeficiency virus (HIV)-associated sensory neuropathy [61]. Furthermore, NGF was effective in preventing the progression of peripheral neuropathies that are associated with HIV or diabetes [62]. Anti-NGF mAb therapy has been the subject of several preclinical investigations for the treatment of neuropathic pain including cancer-induced neuropathic pain. Research by Dai et al. [63] showed that inhibiting NGF reduced the activities of p65 and MAPK and alleviated neuropathic pain associated with chronic constriction injury. Additionally, local application of anti-NGF decreased the severity of heat-induced hypersensitivity in rats with trigeminal neuropathic pain, according to a study conducted by Dos Reis et al. [64]. As shown by Sainoh et al. [65], anti-NGF antibodies have the potential to be a useful tool in the management of neuropathic cancer pain.
Several lines of evidences show the role of NGF in orofacial pain. Jasim and co-workers conducted an examination to assess the levels of NGF in the saliva and plasma of 39 patients with chronic temporomandibular disorder (TMD)-myalgia and 39 pain-free individuals and found that the salivary levels of NGF decreased in TMD-myalgia patients [66]. Also, injecting NGF into the masseter muscle increased mechanical sensitization in women more than men indicating that NGF-induced muscle pain is sex-related [67]. Patients with multiple extractions of teeth perceived an increase in pain, particularly mechanical hyperalgesia, which might be prevented by using antibodies that reduce NGF activity. Furthermore, it was found that the trigeminal ganglia (TG) and periodontal tissues had elevation in the levels of NGF expression and that the application of mechanical stimuli to periodontal fibroblasts increased NGF production [68]. Thus, NGF-based gene therapy was proposed as a mediator for the mechanical hyperalgesia that results from tooth movement [69].
According to this study, control volunteers reported significant changes in the excitability of the corticomotor, while NGF (injected to the masseter muscle of individuals suffering from teeth grinding) did not demonstrate any changes in the central regulation of motor pathways due to NGF-induced sensitization combined with a motor training assignment [70]. According to Mai et al, NGF is involved in orofacial pain via regulating TRPV1 expression at the nociceptor level [70]. In addition, NGF antagonists reversed complete Freund’s adjuvant-induced increase in the number of TRPV1-positive neurons that innervate the lower lip [50]. Also, it was found that blocking NGF reduced TRPA1 expression in an oral cancer pain model suggesting that NGF may contribute to the development of hyperalgesia in the orofacial region via TRPA1 activation [71].
Mixed results were reported for the effects of NGF on visceral pain. In patients suffering from diarrhea-predominant irritable bowel syndrome, it was found that NGF interacts with sensory nerve fibers and mast cells in mediating visceral hypersensitivity [72]. Also, NGF was found in high concentrations in the urine of patients suffering from visceral dysfunction [73]. Also, in a model of neonatal colon inflammation, NGF was correlated with gastric hypersensitivity and its levels increased in gastric fundus [74]. Attenuation of NGF with gastrula mAbs, especially when humanized, is being examined in clinical trials as an analgesic option for a variety of visceral pain conditions such as diabetes and post-surgery [75,76]. In bioelectrical rehabilitation, the results suggested that microneedling could also up-regulate the expression of NGF genes, hence playing a significant role in the accelerated process of nerve regeneration in addition to restoring the normal functioning of the peripheral nerves [77]. According to Liang et al. [78], the researchers explored the involvement of electroacupuncture in visceral pain management as well as NGF producing nanocomponents in colorectal pain and revealed that the nanocomponents displayed the capability to relieve visceral pain by increasing NGF expression.
Elevated levels of NGF have been associated with various pain states making it a target for pain management [3,5]. Accumulating pieces of evidence have shown that the available drugs (i.e., opiates and non-steroidal anti-inflammatory drugs [NSAIDs]) that are used for the treatment of chronic pain leads to long-term side effects such as cardiac, gastrointestinal, or renal adverse effects, suggesting the need to look for other alternatives [5,16]. Antibodies or neutralizing medicines that target NGF signaling and its ability to modulate pain in the long term, have been studied in animal models. Under the request to license the subcutaneous injection of 2.5 mg tanezumab, the Food and Drug Administration (FDA) approved its use on March 2, 2020 [79,80]. NGF inhibitors are safer and more cost-effective than joint replacement surgery, the conventional alternative treatment for knee and hip OA [79,80]. Multiple approaches were established for targeting NGF to relive pain such as preventing NGF binding, activation or inhibiting of TrkA binding/signaling and sequestration of free NGF [2,5]. NGF inhibitors were used as analgesics in chronic pain conditions such as chronic LBP and OA with better outcomes compared to NSAIDs [13]. Also worth mentioning is the importance of pointing to the different signaling of NGF in the acute and chronic phases of pain. For example, it was revealed that there is an increase in p75NTR-mRNA level in the chronic phase of the experimental model of autoimmune encephalomyelitis leading to an elevation in the p75NTR to TrkA at the chronic phase of this model [81]. Also, the expression of NGF protein increased slightly during the acute phase whereas the expression of TrkA in the brains of mice was stable during this phase [81]. Additionally, several reports showed that NGF drives local neuronal sprouting and increases the excitability of the nervous system (promotes sensitization of neurons) which is a key process in many conditions of chronic pain [2]. These findings indicate the importance of tailoring drugs that are used in the acute phase differently than the ones used for the chronic phase. Hirose et al. [12] discussed the development of analgesics targeting NGF/TrkA signaling, noting that this approach can be effective for treating intractable pain without the adverse effects that are commonly associated with traditional analgesic drugs. Also, several phase 2/3 studies have been done that target NGF and TrkA inhibitors. Furthermore, humanized anti-NGF mAbs (i.e., tanezumab, fulranumab, and fasinumab) were used in phase III trials in OA [82]. Additionally, the use of anti-NGF antibodies in patients suffering from knee OA improved stiffness, physical function, and patient global assessment [68]. Chang et al. [2] reported the effectiveness of NGF inhibitors for neuropathic and other types of pain, such as cancer and visceral pain. Peach et al. [49] found that the co-receptor for NGF is responsible for the transfer of signals to TrkA receptors, and that neuropilin 1 antagonism in nociceptors is a new approach for NGF-mediated pain. Also, another study demonstrated that development of abnormal somatosensory behavior was prevented by anti-NGF, suggesting its beneficial effect in the treatment for central pain [83]. Another important aspect in designing valuable drugs is to understand the dual roles of NGF as a pro-inflammatory and anti-inflammatory mediator which is dependent on the type and expression of NGF receptors, cellular context, and the stage of the inflammatory process. Understating these roles is crucial to tailor the inhibition of NGF in the right place and to avoid inhibiting NGF when it has an anti-inflammatory effect. Providing more detail, El-Hashim et al. [84] pointed to the role of NGF (when administered by inhalation or intracerebroventricularly) in cough and airway obstruction in guinea pigs by increasing the phosphorylation of TrkA receptors in the bronchi. On the other hand, it was shown that the expression of anti-inflammatory mediators such as IL-10 can be induced by NGF [85].
Despite the promising analgesic efficacy of anti-NGF mAbs, their development has been complicated by safety concerns, particularly regarding their long-term use. These concerns include the possibility of aggravating peripheral neuropathies and joint issues. Also, the use of anti-NGF mAbs (mainly tanezumab) in the treatment of OA was correlated with some side effects in clinical trials such as rapidly progressive OA of the knee and hip joints [13] or neurological effects [86]. Also, Bernard [87] used NGF vaccine in a mouse model of OA (destabilization of the medial meniscus) and reported the effectiveness of this vaccine in pain reduction.
Although the evidence of the efficacy for NGF inhibitors in knee OA is robust, the incidence of adverse effects increases with higher doses, longer exposure, and concomitant use of NSAIDs. In patients with musculoskeletal pain, according to Katz et al. [46], the intravenous administration of 200 μg/kg tanezumab plus an oral placebo twice a day showed analgesic effects with some adverse effects. Lane and Corr [40] discussed the efficacy of NGF inhibitors in reducing musculoskeletal pain and reported some adverse effects associated with the use of these inhibitors, such as accelerated arthropathy. Thus, the FDA halted all anti-NGF trials due to reports of rare cases of rapidly progressive joint degeneration that necessitated joint replacement, especially in those co-treated with NSAIDs [38].
As discussed in this review, the use of anti-NGF therapy showed promising effects in alleviating different types of pain. At the same time, there are adverse effects for using this therapy. More research is needed to find out routes for applying anti-NGF directly to the site of pain and to decrease its systemic side effects. Nanoparticles can be useful in this regard. To add, combinational therapy using anti-NGF therapy with other drugs can be good area for future research. Also, there is a need to explore the efficacy of anti-NGF therapy in the long term. Additionally, it would be very beneficial if scientists could design and tailor personalized NGF therapies based on the proteomics, genetic, and metabolomics analysis of patients. Finally, more research is needed to elucidate the differential mechanisms of NGF in acute and chronic pain in order to tailor drugs specifically for each case.
Collectively, all of the aforementioned studies point to the effectiveness and the mechanisms of NGF in alleviating different types of pain. Future work is needed to find the adverse effects of NFG that appear when combining NGF and other drugs. Filling this gap can be effective in mitigating these problems.
ACKNOWLEDGMENTS
This review was published with the support of the Deanship of Scientific Research and Graduate Studies at Philadelphia University, Amman, Jordan.
Notes
REFERENCES
1. Levi-Montalcini R, Angeletti PU. 1963; Essential role of the nerve growth factor in the survival and maintenance of dissociated sensory and sympathetic embryonic nerve cells in vitro. Dev Biol. 6:653–9. DOI: 10.1016/0012-1606(63)90149-0. PMID: 13930092.
2. Chang DS, Hsu E, Hottinger DG, Cohen SP. 2016; Anti-nerve growth factor in pain management: current evidence. J Pain Res. 9:373–83. DOI: 10.2147/JPR.S89061. PMID: 27354823. PMCID: PMC4908933.
3. Yuan H, Du S, Chen L, Xu X, Wang Y, Ji F. 2020; Hypomethylation of nerve growth factor (NGF) promotes binding of C/EBPα and contributes to inflammatory hyperalgesia in rats. J Neuroinflammation. 17:34. DOI: 10.1186/s12974-020-1711-1. PMID: 31980031. PMCID: PMC6982391.
4. Bennett DL. 2001; Neurotrophic factors: important regulators of nociceptive function. Neuroscientist. 7:13–7. DOI: 10.1177/107385840100700105. PMID: 11486340.
5. Hefti FF, Rosenthal A, Walicke PA, Wyatt S, Vergara G, Shelton DL, et al. 2006; Novel class of pain drugs based on antagonism of NGF. Trends Pharmacol Sci. 27:85–91. DOI: 10.1016/j.tips.2005.12.001. PMID: 16376998.
6. Crowley C, Spencer SD, Nishimura MC, Chen KS, Pitts-Meek S, Armanini MP, et al. 1994; Mice lacking nerve growth factor display perinatal loss of sensory and sympathetic neurons yet develop basal forebrain cholinergic neurons. Cell. 76:1001–11. DOI: 10.1016/0092-8674(94)90378-6. PMID: 8137419.
7. Schmelz M, Mantyh P, Malfait AM, Farrar J, Yaksh T, Tive L, et al. 2019; Nerve growth factor antibody for the treatment of osteoarthritis pain and chronic low-back pain: mechanism of action in the context of efficacy and safety. Pain. 160:2210–20. DOI: 10.1097/j.pain.0000000000001625. PMID: 31145219. PMCID: PMC6756297.
8. Osikowicz M, Longo G, Allard S, Cuello AC, Ribeiro-da-Silva A. 2013; Inhibition of endogenous NGF degradation induces mechanical allodynia and thermal hyperalgesia in rats. Mol Pain. 9:37. Erratum in: Mol Pain 2013; 9: 55. DOI: 10.1186/1744-8069-9-37. PMID: 23889761. PMCID: PMC3737061.
9. Price TJ, Flores CM. 2007; Critical evaluation of the colocalization between calcitonin gene-related peptide, substance P, transient receptor potential vanilloid subfamily type 1 immunoreactivities, and isolectin B4 binding in primary afferent neurons of the rat and mouse. J Pain. 8:263–72. DOI: 10.1016/j.jpain.2006.09.005. PMID: 17113352. PMCID: PMC1899162.
10. Truzzi F, Marconi A, Lotti R, Dallaglio K, French LE, Hempstead BL, et al. 2008; Neurotrophins and their receptors stimulate melanoma cell proliferation and migration. J Invest Dermatol. 128:2031–40. DOI: 10.1038/jid.2008.21. PMID: 18305571.
11. Ricci A, Salvucci C, Castelli S, Carraturo A, de Vitis C, D'Ascanio M. 2022; Adenocarcinomas of the lung and neurotrophin system: a review. Biomedicines. 10:2531. DOI: 10.3390/biomedicines10102531. PMID: 36289793. PMCID: PMC9598928.
12. Hirose M, Kuroda Y, Murata E. 2016; NGF/TrkA signaling as a therapeutic target for pain. Pain Pract. 16:175–82. DOI: 10.1111/papr.12342. PMID: 26452158.
13. Wise BL, Seidel MF, Lane NE. 2021; The evolution of nerve growth factor inhibition in clinical medicine. Nat Rev Rheumatol. 17:34–46. DOI: 10.1038/s41584-020-00528-4. PMID: 33219344.
14. Denk F, Bennett DL, McMahon SB. 2017; Nerve growth factor and pain mechanisms. Annu Rev Neurosci. 40:307–25. DOI: 10.1146/annurev-neuro-072116-031121. PMID: 28441116.
15. Zhang X, Huang J, McNaughton PA. 2005; NGF rapidly increases membrane expression of TRPV1 heat-gated ion channels. EMBO J. 24:4211–23. DOI: 10.1038/sj.emboj.7600893. PMID: 16319926. PMCID: PMC1356334.
16. Oo WM, Hunter DJ. 2021; Nerve growth factor (NGF) inhibitors and related agents for chronic musculoskeletal pain: a comprehensive review. BioDrugs. 35:611–41. DOI: 10.1007/s40259-021-00504-8. PMID: 34807432.
17. Barker PA, Mantyh P, Arendt-Nielsen L, Viktrup L, Tive L. 2020; Nerve growth factor signaling and its contribution to pain. J Pain Res. 13:1223–41. DOI: 10.2147/JPR.S247472. PMID: 32547184. PMCID: PMC7266393.
18. Vera DB, Fredes AN, Garrido MP, Romero C. 2021; Role of mitochondria in interplay between NGF/TRKA, miR-145 and possible therapeutic strategies for epithelial ovarian cancer. Life (Basel). 12:8. DOI: 10.3390/life12010008. PMID: 35054401. PMCID: PMC8779980.
19. Iftinca M, Defaye M, Altier C. 2021; TRPV1-targeted drugs in development for human pain conditions. Drugs. 81:7–27. DOI: 10.1007/s40265-020-01429-2. PMID: 33165872.
20. Shane Anderson A, Loeser RF. 2010; Why is osteoarthritis an age-related disease? Best Pract Res Clin Rheumatol. 24:15–26. DOI: 10.1016/j.berh.2009.08.006. PMID: 20129196. PMCID: PMC2818253.
21. Molloy NH, Read DE, Gorman AM. 2011; Nerve growth factor in cancer cell death and survival. Cancers (Basel). 3:510–30. DOI: 10.3390/cancers3010510. PMID: 24212627. PMCID: PMC3756375.
22. Seidel MF, Herguijuela M, Forkert R, Otten U. 2010; Nerve growth factor in rheumatic diseases. Semin Arthritis Rheum. 40:109–26. DOI: 10.1016/j.semarthrit.2009.03.002. PMID: 19481238.
23. Banks BE, Vernon CA, Warner JA. 1984; Nerve growth factor has anti-inflammatory activity in the rat hindpaw oedema test. Neurosci Lett. 47:41–5. DOI: 10.1016/0304-3940(84)90383-5. PMID: 6462528.
24. Gigante A, Bevilacqua C, Pagnotta A, Manzotti S, Toesca A, Greco F. 2003; Expression of NGF, Trka and p75 in human cartilage. Eur J Histochem. 47:339–44. DOI: 10.4081/844. PMID: 14706929.
25. Smeyne RJ, Klein R, Schnapp A, Long LK, Bryant S, Lewin A, et al. 1994; Severe sensory and sympathetic neuropathies in mice carrying a disrupted Trk/NGF receptor gene. Nature. 368:246–9. DOI: 10.1038/368246a0. PMID: 8145823.
26. Schnitzer TJ, Marks JA. 2015; A systematic review of the efficacy and general safety of antibodies to NGF in the treatment of OA of the hip or knee. Osteoarthritis Cartilage. 23 Suppl 1:S8–17. DOI: 10.1016/j.joca.2014.10.003. PMID: 25527221.
27. McNamee KE, Burleigh A, Gompels LL, Feldmann M, Allen SJ, Williams RO, et al. 2010; Treatment of murine osteoarthritis with TrkAd5 reveals a pivotal role for nerve growth factor in non-inflammatory joint pain. Pain. 149:386–92. DOI: 10.1016/j.pain.2010.03.002. PMID: 20350782.
28. Voga M, Majdic G. 2022; Articular cartilage regeneration in veterinary medicine. Adv Exp Med Biol. 1401:23–55. DOI: 10.1007/5584_2022_717. PMID: 35733035.
29. von Loga IS, El-Turabi A, Jostins L, Miotla-Zarebska J, Mackay-Alderson J, Zeltins A, et al. 2019; Active immunisation targeting nerve growth factor attenuates chronic pain behaviour in murine osteoarthritis. Ann Rheum Dis. 78:672–5. DOI: 10.1136/annrheumdis-2018-214489. PMID: 30862648. PMCID: PMC6517802.
30. Aso K, Izumi M, Okanoue Y, Ikeuchi M. 2020; Effects of intraarticular injection of anti-nerve growth factor neutralizing antibody on pain in osteoarthritis rat. Int J Pain Relief. 4:1–5.
31. Dakin P, DiMartino SJ, Gao H, Maloney J, Kivitz AJ, Schnitzer TJ, et al. 2019; The efficacy, tolerability, and joint safety of fasinumab in osteoarthritis pain: a phase IIb/III double-blind, placebo-controlled, randomized clinical trial. Arthritis Rheumatol. 71:1824–34. DOI: 10.1002/art.41012. PMID: 31207169. PMCID: PMC6900077.
32. Miyagi M, Ishikawa T, Kamoda H, Suzuki M, Inoue G, Sakuma Y, et al. 2017; Efficacy of nerve growth factor antibody in a knee osteoarthritis pain model in mice. BMC Musculoskelet Disord. 18:428. DOI: 10.1186/s12891-017-1792-x. PMID: 29100502. PMCID: PMC5670727.
33. Xu L, Nwosu LN, Burston JJ, Millns PJ, Sagar DR, Mapp PI, et al. 2016; The anti-NGF antibody muMab 911 both prevents and reverses pain behaviour and subchondral osteoclast numbers in a rat model of osteoarthritis pain. Osteoarthritis Cartilage. 24:1587–95. DOI: 10.1016/j.joca.2016.05.015. PMID: 27208420. PMCID: PMC5009895.
34. Majuta LA, Guedon JG, Mitchell SAT, Ossipov MH, Mantyh PW. 2017; Anti-nerve growth factor therapy increases spontaneous day/night activity in mice with orthopedic surgery-induced pain. Pain. 158:605–17. DOI: 10.1097/j.pain.0000000000000799. PMID: 28301858. PMCID: PMC5370196.
35. LaBranche TP, Bendele AM, Omura BC, Gropp KE, Hurst SI, Bagi CM, et al. 2017; Nerve growth factor inhibition with tanezumab influences weight-bearing and subsequent cartilage damage in the rat medial meniscal tear model. Ann Rheum Dis. 76:295–302. DOI: 10.1136/annrheumdis-2015-208913. PMID: 27381034. PMCID: PMC5264211.
36. Huang H, Shank G, Ma L, Tallents RH, Kyrkanides S. 2013; Nerve growth factor induced after temporomandibular joint inflammation decelerates chondrocyte differentiation. Oral Dis. 19:604–10. DOI: 10.1111/odi.12045. PMID: 23231346.
37. Mammoto T, Seerattan RA, Paulson KD, Leonard CA, Bray RC, Salo PT. 2008; Nerve growth factor improves ligament healing. J Orthop Res. 26:957–64. DOI: 10.1002/jor.20615. PMID: 18302239.
38. Pecchi E, Priam S, Gosset M, Pigenet A, Sudre L, Laiguillon MC, et al. 2014; Induction of nerve growth factor expression and release by mechanical and inflammatory stimuli in chondrocytes: possible involvement in oa pain. Osteoarthritis Cartilage. 22:S23. DOI: 10.1016/j.joca.2014.02.065.
39. Johnson AC, Farmer AD, Ness TJ, Greenwood-Van Meerveld B. 2020; Critical evaluation of animal models of visceral pain for therapeutics development: a focus on irritable bowel syndrome. Neurogastroenterol Motil. 32:e13776. DOI: 10.1111/nmo.13776. PMID: 31833625. PMCID: PMC7890461.
40. Lane NE, Corr M. 2017; Osteoarthritis in 2016: anti-NGF treatments for pain - two steps forward, one step back? Nat Rev Rheumatol. 13:76–8. DOI: 10.1038/nrrheum.2016.224. PMID: 28119540.
41. Meng F, Li H, Feng H, Long H, Yang Z, Li J, et al. 2022; Efficacy and safety of biologic agents for the treatment of osteoarthritis: a meta-analysis of randomized placebo-controlled trials. Ther Adv Musculoskelet Dis. 14:1759720X221080377. DOI: 10.1177/1759720X221080377. PMID: 35282570. PMCID: PMC8908403.
42. Pallav M, Zaripova L, Tazhibaeva D, Kabdualieva N. 2022; POS1126 clinical efficacy and safety of monoclonal antibody against nerve growth factor and fibroblast growth factor-18 therapy of osteoarthritis. Ann Rheum Dis. 81:892. DOI: 10.1136/annrheumdis-2022-eular.3584.
43. Leite VF, Buehler AM, El Abd O, Benyamin RM, Pimentel DC, Chen J, et al. 2014; Anti-nerve growth factor in the treatment of low back pain and radiculopathy: a systematic review and a meta-analysis. Pain Physician. 17:E45–60. DOI: 10.36076/ppj.2014/17/E45. PMID: 24452657.
44. Markman JD, Bolash RB, McAlindon TE, Kivitz AJ, Pombo-Suarez M, Ohtori S, et al. 2020; Tanezumab for chronic low back pain: a randomized, double-blind, placebo- and active-controlled, phase 3 study of efficacy and safety. Pain. 161:2068–78. DOI: 10.1097/j.pain.0000000000001928. PMID: 32453139. PMCID: PMC7431140.
45. Yang S, Huang Y, Ye Z, Li L, Zhang Y. 2020; The efficacy of nerve growth factor antibody for the treatment of osteoarthritis pain and chronic low-back pain: a meta-analysis. Front Pharmacol. 11:817. DOI: 10.3389/fphar.2020.00817. PMID: 33981217. PMCID: PMC8108005.
46. Katz N, Borenstein DG, Birbara C, Bramson C, Nemeth MA, Smith MD, et al. 2011; Efficacy and safety of tanezumab in the treatment of chronic low back pain. Pain. 152:2248–58. DOI: 10.1016/j.pain.2011.05.003. PMID: 21696889.
47. Kivitz AJ, Gimbel JS, Bramson C, Nemeth MA, Keller DS, Brown MT, et al. 2013; Efficacy and safety of tanezumab versus naproxen in the treatment of chronic low back pain. Pain. 154:1009–21. DOI: 10.1016/j.pain.2013.03.006. PMID: 23628600.
48. Reed NR, Reed WR, Syrett M, Richey ML, Frolov A, Little JW. 2022; Somatosensory behavioral alterations in a NGF-induced persistent low back pain model. Behav Brain Res. 418:113617. DOI: 10.1016/j.bbr.2021.113617. PMID: 34606776.
49. Peach CJ, Tonello R, Gomez K, Calderon-Rivera A, Bruni R, Bansia H, et al. 2024. Neuropilin-1 is a co-receptor for NGF and TrkA-evoked pain. bioRxiv 570398 [Preprint]. Available at: https://doi.org/10.1101/2023.12.06.570398. cited 2024 July 3. DOI: 10.1101/2023.12.06.570398.
50. Mai L, Huang F, Zhu X, He H, Fan W. 2020; Role of nerve growth factor in orofacial pain. J Pain Res. 13:1875–82. DOI: 10.2147/JPR.S250030. PMID: 32801845. PMCID: PMC7399448.
51. Descamps S, Toillon RA, Adriaenssens E, Pawlowski V, Cool SM, Nurcombe V, et al. 2001; Nerve growth factor stimulates proliferation and survival of human breast cancer cells through two distinct signaling pathways. J Biol Chem. 276:17864–70. DOI: 10.1074/jbc.M010499200. PMID: 11359788.
52. Verbeke S, Meignan S, Lagadec C, Germain E, Hondermarck H, Adriaenssens E, et al. 2010; Overexpression of p75(NTR) increases survival of breast cancer cells through p21(waf1). Cell Signal. 22:1864–73. DOI: 10.1016/j.cellsig.2010.07.014. PMID: 20667470.
53. Lin H, Huang H, Yu Y, Chen W, Zhang S, Zhang Y. 2021; Nerve growth factor regulates liver cancer cell polarity and motility. Mol Med Rep. 23:288. DOI: 10.3892/mmr.2021.11927. PMID: 33649819. PMCID: PMC7905331.
54. Buehlmann D, Ielacqua GD, Xandry J, Rudin M. 2019; Prospective administration of anti-nerve growth factor treatment effectively suppresses functional connectivity alterations after cancer-induced bone pain in mice. Pain. 160:151–9. DOI: 10.1097/j.pain.0000000000001388. PMID: 30161041.
55. Sevcik MA, Ghilardi JR, Peters CM, Lindsay TH, Halvorson KG, Jonas BM, et al. 2005; Anti-NGF therapy profoundly reduces bone cancer pain and the accompanying increase in markers of peripheral and central sensitization. Pain. 115:128–41. DOI: 10.1016/j.pain.2005.02.022. PMID: 15836976.
56. Jimenez-Andrade JM, Ghilardi JR, Castañeda-Corral G, Kuskowski MA, Mantyh PW. 2011; Preventive or late administration of anti-NGF therapy attenuates tumor-induced nerve sprouting, neuroma formation, and cancer pain. Pain. 152:2564–74. DOI: 10.1016/j.pain.2011.07.020. PMID: 21907491. PMCID: PMC3199350.
57. Guedon JG, Longo G, Majuta LA, Thomspon ML, Fealk MN, Mantyh PW. 2016; Dissociation between the relief of skeletal pain behaviors and skin hypersensitivity in a model of bone cancer pain. Pain. 157:1239–47. DOI: 10.1097/j.pain.0000000000000514. PMID: 27186713. PMCID: PMC5142607.
58. Jensen TS, Baron R, Haanpää M, Kalso E, Loeser JD, Rice ASC, et al. 2011; A new definition of neuropathic pain. Pain. 152:2204–5. DOI: 10.1016/j.pain.2011.06.017. PMID: 21764514.
59. Foley KM. 2003; Opioids and chronic neuropathic pain. N Engl J Med. 348:1279–81. DOI: 10.1056/NEJMe030014. PMID: 12660393.
60. Cirillo G, Cavaliere C, Bianco MR, De Simone A, Colangelo AM, Sellitti S, et al. 2010; Intrathecal NGF administration reduces reactive astrocytosis and changes neurotrophin receptors expression pattern in a rat model of neuropathic pain. Cell Mol Neurobiol. 30:51–62. DOI: 10.1007/s10571-009-9430-2. PMID: 19585233.
61. McArthur JC, Yiannoutsos C, Simpson DM, Adornato BT, Singer EJ, Hollander H, et al. 2000; A phase II trial of nerve growth factor for sensory neuropathy associated with HIV infection. AIDS Clinical Trials Group Team 291. Neurology. 54:1080–8. Erratum in: Neurology 2000; 55: 162. DOI: 10.1212/WNL.54.5.1080. PMID: 10720278.
62. Schnitzer TJ, Khan A, Bessette L, Davignon I, Brown MT, Pixton G, et al. 2020; Onset and maintenance of efficacy of subcutaneous tanezumab in patients with moderate to severe osteoarthritis of the knee or hip: a 16-week dose-titration study. Semin Arthritis Rheum. 50:387–93. DOI: 10.1016/j.semarthrit.2020.03.004. PMID: 32252976.
63. Dai WL, Yan B, Bao YN, Fan JF, Liu JH. 2020; Suppression of peripheral NGF attenuates neuropathic pain induced by chronic constriction injury through the TAK1-MAPK/NF-κB signaling pathways. Cell Commun Signal. 18:66. DOI: 10.1186/s12964-020-00556-3. PMID: 32312253. PMCID: PMC7171864.
64. Dos Reis RC, Kopruszinski CM, Nones CF, Chichorro JG. 2016; Nerve growth factor induces facial heat hyperalgesia and plays a role in trigeminal neuropathic pain in rats. Behav Pharmacol. 27:528–35. DOI: 10.1097/FBP.0000000000000246. PMID: 27392124.
65. Sainoh T, Sakuma Y, Miyagi M, Orita S, Yamauchi K, Inoue G, et al. 2014; Efficacy of anti-nerve growth factor therapy for discogenic neck pain in rats. Spine (Phila Pa 1976). 39:E757–62. DOI: 10.1097/BRS.0000000000000340. PMID: 24732837.
66. Jasim H, Ghafouri B, Gerdle B, Hedenberg-Magnusson B, Ernberg M. 2020; Altered levels of salivary and plasma pain related markers in temporomandibular disorders. J Headache Pain. 21:105. DOI: 10.1186/s10194-020-01160-z. PMID: 32842964. PMCID: PMC7449051.
67. Alhilou AM, Shimada A, Svensson CI, Svensson P, Ernberg M, Cairns BE, et al. 2021; Sex-related differences in response to masseteric injections of glutamate and nerve growth factor in healthy human participants. Sci Rep. 11:13873. DOI: 10.1038/s41598-021-93171-2. PMID: 34230516. PMCID: PMC8260580.
68. Gao Y, Hu Z, Huang Y, Liu W, Ren C. 2022; Efficacy and safety of anti-nerve growth factor antibody therapy for hip and knee osteoarthritis: a meta-analysis. Orthop J Sports Med. 10:23259671221088590. DOI: 10.1177/23259671221088590. PMID: 35494494. PMCID: PMC9047886.
69. Radithia D, Soebadi B, Parmadiati AE, Winias S. Rahmi. 2022; Nerve growth factor and S100B: molecular marker of neuroregeneration after injection of freeze-dried platelet rich plasma. J Oral Biol Craniofac Res. 12:570–4. DOI: 10.1016/j.jobcr.2022.07.006. PMID: 35957941. PMCID: PMC9361313.
70. Boscato N, Exposto FG, Costa YM, Svensson P. 2022; Effect of standardized training in combination with masseter sensitization on corticomotor excitability in bruxer and control individuals: a proof of concept study. Sci Rep. 12:17469. DOI: 10.1038/s41598-022-21504-w. PMID: 36261447. PMCID: PMC9581922.
71. Ye Y, Dang D, Zhang J, Viet CT, Lam DK, Dolan JC, et al. 2011; Nerve growth factor links oral cancer progression, pain, and cachexia. Mol Cancer Ther. 10:1667–76. DOI: 10.1158/1535-7163.MCT-11-0123. PMID: 21750223. PMCID: PMC3375020.
72. Xu XJ, Zhang YL, Liu L, Pan L, Yao SK. 2017; Increased expression of nerve growth factor correlates with visceral hypersensitivity and impaired gut barrier function in diarrhoea-predominant irritable bowel syndrome: a preliminary explorative study. Aliment Pharmacol Ther. 45:100–14. DOI: 10.1111/apt.13848. PMID: 27862119.
73. Coelho A, Oliveira R, Antunes-Lopes T, Cruz CD. 2019; Partners in crime: NGF and BDNF in visceral dysfunction. Curr Neuropharmacol. 17:1021–38. DOI: 10.2174/1570159X17666190617095844. PMID: 31204623. PMCID: PMC7052822.
74. Li Q, Winston JH, Sarna SK. 2016; Noninflammatory upregulation of nerve growth factor underlies gastric hypersensitivity induced by neonatal colon inflammation. Am J Physiol Regul Integr Comp Physiol. 310:R235–42. DOI: 10.1152/ajpregu.00342.2015. PMID: 26608656. PMCID: PMC4796752.
75. Barker K. 2022. Targeting pro-inflammatory mediators to treat visceral pain. [Doctoral dissertation]. University of Cambridge;Cambridge:
76. Chen Y, Cheng J, Zhang Y, Chen JDZ, Seralu FM. 2021; Electroacupuncture at ST36 relieves visceral hypersensitivity via the NGF/TrkA/TRPV1 peripheral afferent pathway in a rodent model of post-inflammation rectal hypersensitivity. J Inflamm Res. 14:325–39. Erratum in: J Inflamm Res 2021; 14: 393. DOI: 10.2147/JIR.S285146. PMID: 33584100. PMCID: PMC7875081.
77. Regmi B, Shah MK. 2020; Possible implications of animal models for the assessment of visceral pain. Animal Model Exp Med. 3:215–28. DOI: 10.1002/ame2.12130. PMID: 33024943. PMCID: PMC7529330.
78. Liang D, Ren Y, Huang L, Jin S. 2022; A study on the mechanism of electroacupuncture to alleviate visceral pain and NGF expression. Comput Intell Neurosci. 2022:3755439. DOI: 10.1155/2022/3755439. PMID: 36275969. PMCID: PMC9586762.
79. Jiao Y, Lin Y, Zheng J, Shi L, Zheng Y, Zhang Y, et al. 2022; Propionibacterium acnes contributes to low back pain via upregulation of NGF in TLR2-NF-κB/JNK or ROS pathway. Microbes Infect. 24:104980. DOI: 10.1016/j.micinf.2022.104980. PMID: 35430372.
80. Rizzo RRN, Ferraro MC, Wewege MA, Cashin AG, Leake HB, O'Hagan ET, et al. 2022; Targeting neurotrophic factors for low back pain and sciatica: a systematic review and meta-analysis. Rheumatology (Oxford). 61:2243–54. DOI: 10.1093/rheumatology/keab785. PMID: 34677587.
81. Delivanoglou N, Boziki M, Theotokis P, Kesidou E, Touloumi O, Dafi N, et al. 2020; Spatio-temporal expression profile of NGF and the two-receptor system, TrkA and p75NTR, in experimental autoimmune encephalomyelitis. J Neuroinflammation. 17:41. DOI: 10.1186/s12974-020-1708-9. PMID: 31996225. PMCID: PMC6990493.
82. Yeh JF, Akinci A, Al Shaker M, Chang MH, Danilov A, Guileen R, et al. 2017; Monoclonal antibodies for chronic pain: a practical review of mechanisms and clinical applications. Mol Pain. 13:1744806917740233. DOI: 10.1177/1744806917740233. PMID: 29056066. PMCID: PMC5680940.
83. Gwak YS, Bae JY, Jang JH, Yoon DM. 2003; Attenuation of spinal cord injury-induced hyperalgesia by administration of antibody to nerve growth factor in the rat. Korean J Pain. 16:7–13.
84. El-Hashim AZ, Jaffal SM, Al-Rashidi FT, Luqmani YA, Akhtar S. 2013; Nerve growth factor enhances cough via a central mechanism of action. Pharmacol Res. 74:68–77. DOI: 10.1016/j.phrs.2013.05.003. PMID: 23742790.
85. Minnone G, De Benedetti F, Bracci-Laudiero L. 2017; NGF and its receptors in the regulation of inflammatory response. Int J Mol Sci. 18:1028. DOI: 10.3390/ijms18051028. PMID: 28492466. PMCID: PMC5454940.
86. Brown MT, Murphy FT, Radin DM, Davignon I, Smith MD, West CR. 2012; Tanezumab reduces osteoarthritic knee pain: results of a randomized, double-blind, placebo-controlled phase III trial. J Pain. 13:790–8. DOI: 10.1016/j.jpain.2012.05.006. PMID: 22784777.
87. Bernard NJ. 2019; NGF vaccine reduces pain. Nat Rev Rheumatol. 15:251. DOI: 10.1038/s41584-019-0213-y. PMID: 30923347.
Fig. 1
Signaling pathways of NGF in pain. NGF: nerve growth factor, EP: prostaglandin E2 receptor, NMDA: N-methyl-D-aspartic acid, TrkA: tropomyosin receptor kinase A, P2X3: P2X purinoceptor 3, DRG: dorsal root ganglion, TRPV1: transient receptor potential vanilloid 1, SP: substance P, CGRP: calcitonin gene-related peptide, NK1: neurokinin-1.
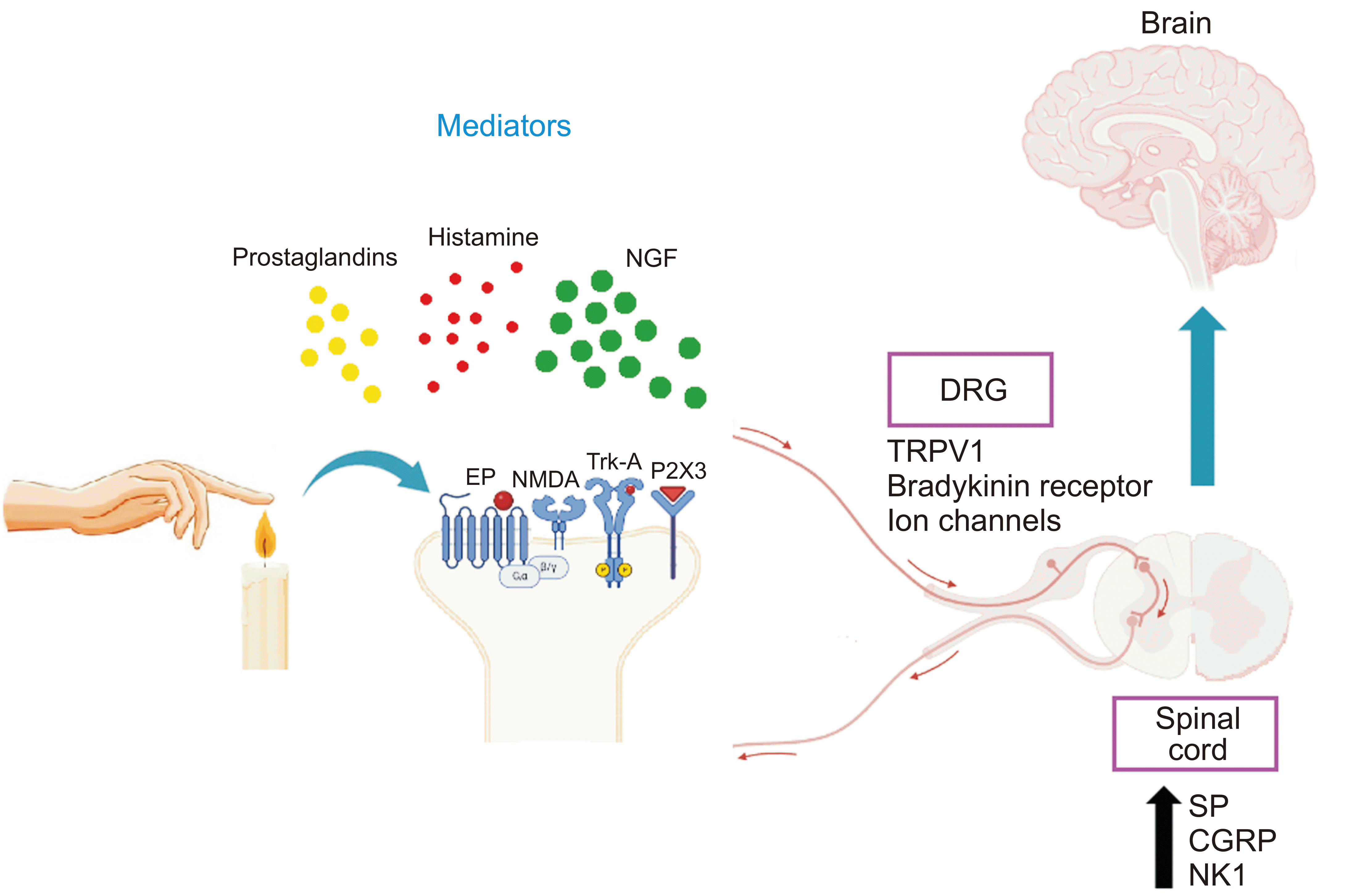