Abstract
Parkinson’s disease (PD) affects about 8.5 million individuals worldwide. Oxidative and inflammatory cascades are implicated in the neurological sequels, that are mostly unresolved in PD treatments. However, proper nutrition offers one of the most effective and least costly ways to decrease the burden of many diseases and their associated risk factors. Moreover, prevention may be the best response to the progressive nature of PD, thus, the therapeutic novelty of honey and levodopa may be prospective. This study aimed to investigate the neuroprotective role of honey and levodopa against 1-methyl-4-phenyl-1,2,3,6-tetrahydropyridine (MPTP)-induced oxidative stress. Fifty-four adult male Swiss mice were divided into control and PD model groups of 27 mice. Each third of the control mice either received phosphate buffered saline, honey, or levodopa for 21 days. However, each third of the PD models was either pretreated with honey and levodopa or not pretreated. Behavioral studies and euthanasia were conducted 2 and 8 days after MPTP administration respectively. The result showed that there were significantly (P<0.05) higher motor activities in the PD models pretreated with the honey as well as levodopa. furthermore, the pretreatments protected the midbrain against the chromatolysis and astrogliosis induced by MPTP. The expression of antioxidant markers (glutathione [GSH] and nuclear factor erythroid 2-related factor 2 [Nrf2]) was also significantly upregulated in the pretreated PD models. It is thus concluded that honey and levodopa comparably protected the substantia nigra pars compacta neurons against oxidative stress by modulating the Nrf2 signaling molecule thereby increasing GSH level to prevent MPTP-induced oxidative stress.
Parkinson’s disease (PD) affects about 8.5 million persons worldwide [1]. In America, the yearly financial impact of PD was projected to be $51.9 billion [2]. One per cent of people 60 years or older are affected by PD [3]. Its prevalence is expected to increase twofold in the year 2040 [4], which is expected to be accompanied by societal and economic burdens unless effective cures or means of prevention are developed. Most neurodegenerative diseases are progressive, and irreversible; thus, early detection and prevention strategies dominate the recent advances in the search for novel treatments. In PD, about 70% of the dopamine-secreting substantia nigra pars compacta (SNpc) cells have been lost before the motor deficit manifestation and initiation of treatment [5]. Thus, investigating the preventive capacity of a nutritional supplement (honey) and repurposing levodopa may revolutionize the management strategies in individuals with high susceptibility to the development of PD, and existing patients.
The well-established cause of PD is ageing which is linked with the accumulation of oxidative stress among other factors [6]. Reactive oxygen species (ROS) generation is linked to the pathophysiology of PD, which damages the SNpc neurons via lipid peroxidation, as well as the oxidation of DNA and protein. Increased ROS is caused by alteration of the system that defends against oxidative stress (e.g., glutathione [GSH] as well as superoxide dismutase [SOD]) [7]. PD ranks as the second most prevalent neurodegenerative condition that is typified by the loss of SNpc cells. This leads to the reduction of dopamine input to the striatum [8]. Patients with PD suffer from high motor dysfunction which is coupled with depression and sleep disorders [9]. However, there is no cure for PD and the long-term usage of the existing drugs is associated with in-effectiveness and undesirable effects.
Positive lifestyle modification has been broadly suggested as an alternative way to manage PD [10]. One of the cardinal and efficient parts of lifestyle changes is diet modification. Moreover, studies have suggested certain food components which can be beneficial to PD patients [11], an example of such is present in honey, whole grains as well as vegetables. The ingestion of food supplements (which includes curcumin, GSH, vitamin C, vitamin E, and creatine [12] and functional foods (e.g., garlic, black pepper, cinnamon, black cumin, and resveratrol [13] that are rich in vitamins and antioxidants have been proposed to have a significant impact in the treatment and prevention of PD.
Honey is an insect-produced gel rich in nutraceutical polyphenols, e.g., luteolin, quercetin, kaempferol, as well as chrysin [14]. These polyphenols account for the majority of the medicinal properties of some therapeutic foods that are used as part of a treatment regimen for a variety of diseases. Regular consumption of polyphenol-containing foods and/or drinks has been associated with a delay in the commencement of Alzheimer’s disease and a decreased risk of developing PD [15]. However, levodopa is the mainstay drug for PD management as it is efficacious in the management of motor symptoms. However, there is a dearth of information concerning its neuroprotective ability.
54 Swiss mice (adult male weighing 25–30 g) were kept at the Central Research Laboratory, University of Ilorin, for the purpose of this research, and allowed to acclimatize for 14 days prior to the experiment. Both food as well as water were freely available. The University of Ilorin Ethical Review Committee endorsed every procedure used for this research (UERC/ASN/2021/2136). 1-methyl-4-phenyl-1,2,3,6-tetrahydropyridine (MPTP) hydrochloride (Catalog No: HY-15608) was purchased from MedChem Express. Phosphate buffered saline (PBS) was bought from Sigma-Aldrich and levodopa (xarachi-75700; Sinemet) was obtained from Momrota Pharmacy. Honey (A & Shine Int’l Ltd.) was purchased from Rotamedics Pharmacy.
The mice were randomly allocated to control and PD model groups of 27 mice. One-third of the control mice either get 0.2 ml PBS orally (PBS), 2 g/kg body weight of honey, or 80 mg/kg body weight of levodopa for 21 days. One-third of the PD models was also either pretreated with honey (honey & MPTP), levodopa (levodopa & MPTP) or not pretreated (MPTP). The intraperitoneal injection of 20 mg/kg body weight of MPTP four times at two hours intervals was employed for PD’s induction. Behavioral tests and animal euthaniation were performed 2 and 8 days post PD induction respectively.
Two days after the induction of PD some behavioral tests were carried out to evaluate locomotion, motor coordination, and gait in the experimental mice. The test includes an open field test (OFT), rotarod test, and footprint test.
To assess locomotor and motor coordination, the experimental mice were subjected to the OFT paradigm. Each mouse was positioned in the center of the paradigm (a well-illuminated box with a wall made of wood and a tiled ceramic floor divided into 4 by 4 squares) and allowed to freely explore it for 5-minute under a monitoring video. For locomotion and motor coordination, the video recordings were examined for the number of rearing and line crossed by the animals respectively.
The coordination of motor activities was also examined utilizing a rotarod paradigm. The experimental animals were trained on the paradigm (10 revolution/minute linearly) prior to the test proper for 3 minutes. the test was conducted at a uniform speed of 10 revolution/minute in 3 minutes. The activities were captured with a camera after which the time it takes for the animal to fall (fall latency) was noted from the recorded videos.
This was performed to evaluate the gait of the experimental animals. The forefeet and the hindfeet were coated with different colors of nontoxic paints. The mice had the opportunity to walk through a (25 cm long and 7 cm wide) side-enclosed runway. A piece of paper was laid on the runway’s floor. To assess the gait pattern, the length of stride and fore-base width were measured in centimeters and compared across groups.
The mice were sequentially perfused (transcardially) with PBS and neutral buffered formalin (NBF). Sections of the midbrain showing substantia nigra were then exercised, fixed in NBF, treated in increasing concentrations of alcohol, cleared and encased in paraffin wax. Midbrain slices of 5 μm were obtained and stained with cresyl fast violet. Immunohistochemical technique was also carried out on similar coronal slices of the midbrain. In brief, midbrain slices were deparaffinized and rehydrated in declining concentrations of alcohol before immersing in water. The antigen was then retrieved in a steamer for 30 minutes. After that, sections were washed in PBS before exposure to peroxidase blocking for 10 minutes. These were rewashed for 2 minutes in PBS before incubating them in 2.5% normal horse serum for 20 minutes to block unbounded protein. Finally, the midbrain slices were incubated in primary antibodies (glial fibrillary acidic protein [GFAP: #SC-33673], 1:100; tyrosine hydroxylase [TH: #SC-25269], 1:100). The midbrain slices were then washed and placed in ImmPRESSTM horseradish peroxidase (HRP) anti-rabbit immunoglobulin G polymer reagent (Vector® Labs) for 30 minutes before rewashing twice in PBS. The sections were thoroughly rinsed, counter-stained in hematoxylin, dehydrated using increasing concentrations of alcohol, and mounted in Permount (Fischer Scientific). Photomicrographs were obtained using the Amscope camera under a light microscope. The primary antibodies were obtained from Santa Cruz Biotechnology.
Three different trained individuals utilized the cell counter tool plugin within ImageJ to count the cells, accepting the average count as the value for each photomicrograph. This process involved opening an image in the ImageJ application and establishing a grid to avoid double-counting cells. Subsequently, the process included initializing and selecting the cell type of interest, followed by counting the cells by clicking on them.
Mice were euthanized at the end of the experiment under inhalation of isoflurane. the brains were carefully removed, after which the midbrain was excised from the brain, the midbrain was then homogenized in a cold solution of sucrose (0.25 M) and centrifuged (3,000 rpm) for 10 minutes, maintaining 4°C temperature. The oxidative stress (ROS, malondialdehyde [MDA], GSH, nuclear factor erythroid 2-related factor 2 [Nrf2], and SOD) markers were assayed from the supernatant.
The level of ROS was estimated by flow cytometry (Partec) which was used to track the rising dichloro-dihydro-fluorescein diacetate fluorescence. The signals were acquired with a 530 nm band pass filter (FL-1 channel).
The SOD activity assay was based on the ability of SOD to inhibit autoxidation of pyrogallol [16]. Five μl of distilled water and midbrain homogenates each were added into the blank and sample wells respectively, after which 100 μl of SOD assay buffer was pipetted to each well, 100 μl of chromogen was then added. The microplate was then gently mixed and the absorbance was read at 420 nm wavelength.
Assay of GSH concentration was done using the Ellman procedure. Ten µl of sample and standard were each added to their respective wells, 100 µl of GSH dilution buffer was then added into the sample and standard wells. Five µl of GSH chromogen was then added to both samples and standard wells; absorbance was read within 5 minutes at 412 nm wavelength.
The Assay of the MDA concentration was based on the reaction of 2-thiobarbituric acid with MDA at 25°C to produce a chromophore. One hundred µl of samples and distilled water were added into the microplate and blank wells respectively. One hundred µl of indicator was then added. The microplate was then heated at 65°C for 45 minutes and then read at a wavelength of 532 nm.
The sandwich-ELISA method was used as the basis for the assay of Nrf2 (Catalog No: E-EL-R1052; Elabscience Biotechnology Inc.) assay. Antibodies specific for Nrf2 were pre-coated on the supplied micro-ELISA plate. When the standards and specimens were pipetted into the wells, the antibody within the wells mixed with them. Following that, each microplate well was treated with a combination of avidin-HRP and a biotinylated detection antibody specific for Nrf2. The specimen wells which also contains biotinylated detection antibodies and avidin-HRP became blue when the substrate solutions were added to each well. The addition of a stop solution and subsequent yellowing of the substance brought an end to the enzyme-substrate reaction. At a wavelength of 450 nm, the optical density (OD) was determined. The OD value relates to the concentration of Nrf2 in the specimens.
The presented charts were plotted using the mean and the standard error of the mean. Differences between the experimental groups were considered statistically significant if the P-value was less than 0.05, employing one-way analysis of variance (ANOVA) and Tukey post-hoc test. The statistical analysis was conducted using GraphPad Prism version 7.0.
PD model: The grooming frequency of MPTP-induced PD models of adult male Swiss mice in the open field maze following exposure to honey and levodopa is shown in Fig. 1A. The grooming frequency of the animals exposed to MPTP only was significantly lower (P<0.05) than that of the PBS group, while the animals administered with honey has the highest grooming frequency. Moreover, honey was able to prevent the reduced grooming induced by MPTP as the grooming frequency of the honey & MPTP group was significantly (P<0.05) higher than that of the MPTP group. Furthermore, the levodopa & MPTP also have significantly higher grooming frequency compared to the non-treated MPTP-induced PD models.
PD model: The line-crossing frequency of MPTP-induced PD models of adult male Swiss mice in the open field maze following the ingestion of honey and levodopa is shown in Fig. 1B. the line-crossing frequency of the mice in the PBS group was significantly (P<0.05) more than those of the animals in the MPTP group. There was no significant difference between the line-crossing frequency of the control animals and honey-administered animals. Honey and levodopa were able to prevent the loss of ambulation induced by MPTP as the line crossing frequency of honey & MPTP as well as levodopa & MPTP groups were significantly higher (P<0.05) than that of the MPTP group.
The rearing frequency of MPTP-induced PD models of adult male Swiss mice in the open field maze after exposure to honey and levodopa is shown in Fig. 1C. The rearing frequency of the MPTP group was significantly the lowest (P<0.05). Honey was able to protect against the loss of vertical locomotion induced by MPTP as the rearing frequencies of the honey & MPTP group was significantly more than that of the MPTP group.
The result from the rotarod test is shown in Fig. 1D. the fall latency of the MPTP group was significantly (P<0.05) lower than PBS and honey & MPTP groups, indicative of the preservation of locomotion and motor coordination by honey. Rotarod test is used to evaluate alteration in basal ganglia pathway.
The mean stride length of MPTP-induced PD models of adult male Swiss mice after exposure to honey and levodopa is shown in Fig. 1E. The mean stride length of the MPTP group was significantly (P<0.05) shorter than that of the PBS group, although the difference in the stride length across all the groups was not significant (P<0.05).
The fore-base width of MPTP-induced PD models of adult male Swiss mice after exposure to honey and levodopa is shown in Fig. 1F. The mean fore-base width of the mice in the MPTP group was significantly (P<0.05) lower than that of levodopa group. However, there was no significant difference between the fore-base width of the non-treated and treated MPTP-induced PD models.
The representative photomicrographs showing the SNpc of the experimental mice stained with cresyl fast violet (CFV) are shown in Fig. 2A while the chromatolytic cell count is shown in Fig. 2B. The sections stained with CFV demonstrate the Nissl substance. Nissl substance is the protein production machinery of the neurons. The SNpc of the mice in the PBS (control) displayed neurons with intact Nissl substance (black arrows) and the number of chromatolytic neurons in this group was significantly lower than that of MPTP group (Fig. 2B). The SNpc of the mice in the MPTP group displayed palely stained neurons indicative of chromatolysis (red arrow), while the number of chromatolytic neurons was significantly (P<0.05) the highest compared to other groups. The SNpc of the mice in the levodopa group depicts more cells that are deeply stained with CFV (black arrow) compared to that of the MPTP group. The SNpc of mice in the honey group depicts neurons with deeply stained cytoplasm indicative of intact Nissl substance. The SNpc of mice in the honey & MPTP group has neurons with more deeply stained cytoplasm which is indicative of intact Nissl substance. Furthermore, there was a significantly lower number of chromatolytic neurons in the honey & MPTP group compared to the MPTP group. The SNpc of mice in levodopa & MPTP also depicts cells that are mostly stained with CFV, while the chromatolytic cell count was significantly lower than that of the MPTP group.
The representative photomicrographs showing the SNpc of the experimental mice stained for TH protein are shown in Fig. 3A while the TH-positive neuronal count is shown in Fig. 3B. The SNpc of the mice in the PBS group display numerous, deeply stained and intact dopaminergic (DA) neurons indicating very high TH immunoreactivity. The SNpc of the mice in the MPTP group displays fewer DA cells in comparison to the PBS group. The SNpc of the mice in the levodopa group shows numerous deeply stained and intact DA neurons indicating high TH expression. The SNpc of the mice in the honey group displays numerous, deeply stained and intact DA neuronal cells indicative of high TH expression. The SNpc of the animals in the honey & MPTP group displayed moderately populated, deeply stained and mostly intact DA neuronal cells indicating moderate TH expression. The SNpc of the animals in the levodopa & MPTP group also display moderately populated and deeply stained DA neurons indicating moderate reactivity.
The representative photomicrographs showing the SNpc of the experimental mice stained for GFAP protein are shown in Fig. 4A while the GFAP-positive cell count is shown in Fig. 4B. The SNpc of the PBS group displays very low GFAP immunoreactivity and few reactive astrocytes. The SNpc of the MPTP group displays very high GFAP reactivity indicative of high expression with numerous reactive astrocytes (red arrow). The SNpc of the levodopa group shows very low reactivity indicative of low GFAP expression and fewer reactive astrocytes. The SNpc of the honey group also displays very low GFAP expression and very few reactive astrocytes indicative of very low immunoreactivity. The SNpc of the honey & MPTP group displays a lower GFAP expression with fewer reactive astrocytes when compared to MPTP group of mice. Similarly, the SNpc of levodopa & MPTP group also shows low GFAP expression and few reactive astrocytes indicative of low reactivity.
The role of honey and levodopa on the level of ROS in the midbrain of the MPTP-induced PD model of adult male Swiss mice is shown in Fig. 5A. There was a significantly higher ROS activity in the midbrain of the MPTP group of mice when compared to the midbrain of the mice treated with PBS (P<0.05). The administration of honey and levodopa before exposure to MPTP significantly lowered ROS level as there was significant (P<0.05) difference between the midbrain ROS level of the honey & MPTP and levodopa & MPTP groups compared to that of the MPTP group.
The effect of honey and levodopa on the concentration of MDA in the midbrain of MPTP-induced PD model of adult male Swiss mice is shown in Fig. 5E. There was a statistically significant higher concentration of MDA in the midbrain of mice treated with MPTP when compared to that PBS, honey and levodopa treated mice (P<0.05) indicative of high lipid peroxidation. Pre-treatment with honey and levodopa before MPTP administration significantly lowers lipid peroxidation as the mean MDA concentration of the honey & MPTP group as well as levodopa & MPTP group was significantly (P<0.05) lower than that of the MPTP group. There was a statistically (P<0.05) significant difference between the mean MDA concentrations of honey & MPTP and levodopa & MPTP groups.
The effect of honey and levodopa on the concentration of reduced GSH in the midbrain of MPTP-induced PD model of adult male Swiss mice is displayed in Fig. 5C. There was a statistically significant (P<0.05) higher concentration of GSH in the midbrain of mice treated with PBS and honey when compared to that of MPTP-treated mice. However, the GSH concentration of honey-treated mice was significantly (P<0.05) higher in comparison to PBS group. In addition to this, administration of honey and levodopa before exposure to MPTP significantly increased the GSH concentration. Moreover, the concentration of GSH in honey & MPTP and levodopa & MPTP was significantly (P<0.05) higher in comparison to MPTP group.
The effect of honey and levodopa on the activities of SOD in the midbrain of the MPTP-induced PD model of adult male Swiss mice is shown in Fig. 5D. The activity of SOD of honey & MPTP group was higher than that of the MPTP group. Levodopa & MPTP group had the highest midbrain SOD activity. There was no statistically (P<0.05) significant difference between the SOD activities of the mice pretreated with honey (honey & MPTP) and levodopa (levodopa & MPTP) before MPTP exposure.
The impact of honey and levodopa on the concentration of Nrf2 in the midbrain of MPTP-induced PD model of adult male Swiss mice is shown in Fig. 5B. There was a significantly (P<0.05) higher level of Nrf2 in the midbrain of mice in the PBS and HON groups when compared to the MPTP group. In addition to this, pretreatment with honey before exposure to MPTP significantly (P<0.05) increased the Nrf2 concentration as there was a statistically significant difference between the midbrain Nrf2 of the honey & MPTP group and the MPTP group. There was no significant difference between the midbrain Nrf2 of the honey & MPTP and levodopa & MPTP groups.
Prevention of PD will go a long way in reducing the burden caused by PD. Moreover, most of the SNpc neurons have been lost before PD diagnosis which makes it difficult to manage. Oxidative stress has been noted as prominent factors as far as the etiology and progression of PD are concerned, significant is the activation of glial cells, serving as the main source of oxidative stressors, inflammatory cytokines, and the release of antioxidants [17].
The movement-associated parameters from the OFT depict that MPTP significantly hindered the movement of the experimental mice evidenced by the least line crossing and rearing frequencies of this group of animals compared to other groups. MPTP models are one of the validated and established animal models for the creation of therapeutic compounds designed to study basal ganglia alterations associated with PD [18], MPTP administration causes motor behavioral aberrations in experimental animals [18]. It was reported that line-crossing frequency and the total distance covered by MPTP-administered mice were significantly lower compared to the control group [19]. However, honey and levodopa were able to prevent the reduced movement induced by MPTP. It can thus be inferred that honey and levodopa were able to prevent the loss of movement induced by MPTP administration by preventing the loss of the SNpc neurons, hence preventing the striatal dopamine depletion via its antioxidative activities. Various studies have reported the neurobehavioral role of honey and its polyphenols; it was reported that honey enhanced locomotion and exploration while diminishing anxiety in rats exposed to lead [20]. Chrysin, one of the honey polyphenols was also reported to have attenuated and restored the altered behavioural changes in PD animal models [21].
Alteration in motor coordination is also a symptom of PD. It was observed that honey and levodopa were able to prevent the altered motor coordination induced by MPTP. This was evidenced by higher rearing and grooming frequency in the MPTP-induced PD models pretreated as compared to the non-pretreated MPTP-induced PD models. Honey pretreatment was also associated with higher latency to fall from the rotarod compared to the non-treated PD models. This finding is similar to the findings of [22] who stated that mice in the MPTP group exhibited decreased forelimb utilization and a shorter time to fall from the rotarod in comparison to both normal control mice and those treated with celastrol. Honey was also reported to have ameliorated neuroinflammation, cognitive impairment, anxiety, and motor impairment induced by lipopolysaccharide [23]. In addition to this, honey-purified bee venom phospholipase-A2 was also documented to ameliorate motor deficit induced by MPTP.
Gait disturbances are common in PD, patients often display slow, shuffling walks with reduced arm swing, sluggish strides, and a hunched posture, The advancing disruption in walking, coupled with postural instability, ultimately robs patients of motor skills thereby reducing their quality of life. Gait disturbance has also been reported in PD models [24-26]. Moreover, it was reported that selegiline alleviates gait impairments and DA neuronal deficit in a MPTP model of PD [25], Zhang and co [24] also observed that eicosanoid protected against MPTP-induced gait deficit which was evidenced by lower stridal length, fore and hind base widths of the MPTP administered. This study also adds to the body of knowledge as the result showed that MPTP induced gait deficit in the adult male Swiss mice while pretreatment with honey and levodopa prevented the gait deficit.
To ascertain that the preservation of motor behaviors by honey and levodopa involves the basal ganglia, TH expression was demonstrated by immunohistochemically staining the midbrain for TH. The group of mice that received MPTP had a lower expression of TH and loss of the DA neurons as there are fewer TH-positive neurons in their SNpc compared to other groups indicative that honey and levodopa protected the neurons from MPTP-induced damage. Supporting the role of honey on TH expression is the [27] report that kaempferol (a honey polyphenol) treatment prevented TH-positive neuronal deficit caused by MPTP. Moreover, MPTP exerts its toxicity via oxidative stress caused by the inhibition of the electron transport chain’s (ETC) complex I ultimately causing elevated levels of ROS. Honey and its polyphenols have been reported to have a profound radical scavenging property, mediation of antioxidant enzyme activities and amelioration of apoptosis thereby preventing cell loss [28]. Honey has also been documented to enhance keratinocytes’ wound-healing abilities [29]. In summary honey and levodopa were able to preserve the SNpc neurons thereby preventing striatal dopamine depletion, and ultimately leading to the preservation of the motor behaviours.
Histopathology of PD usually presents as depigmentation, gliosis, and a decrease in the neuron population, predominantly in the SNpc [30]. It was observed from this study that the SNpc of the PD models had a higher number of chromatolytic neurons. The chromatolytic feature could have resulted from DNA fragmentation and chromatin condensation which in turn leads to nuclear breakdown and neuropil vacuolation. This is contrary to the observation from the MPTP-administered animals that were pretreated with honey and levodopa. The role of MPTP on the histochemistry of SNpc is similar to the findings of Gao and colleagues [31] who reported that MPTP led to a reduced number of SNpc neurons. To reinforce the significance of honey in the preservation of the SNpc; Dietary polyphenols abundant in honey have been reported to show a strong potential to benefit the brain by shielding neurons from harm caused by oxidative stress, reducing neuroinflammation, and enhancing the management of cardiovascular risk factors and function, it mitigates neurotoxic effects and neurodegeneration [32].
Astrogliosis which is characterized by a rise in the quantity of astrocytes was found in the SNpc of MPTP-administered animals. This is similar to what was reported in various studies [33-35]. The pathogenesis and the advancement of PD relies on cellular processes such as malfunctions in protein degradation, oxidative insult, mitochondrial dysfunction, altered mitochondrial autophagy, and the gradual build-up of α-synuclein facilitated by glial cell and neuron interactions, culminating in apoptosis [36]. Research indicates that the accumulation of astrocytic α-synuclein triggers the activation of phagocytic microglia, causing targeted destruction of neurons in specific brain regions [37]. The result from this study showed that honey and levodopa were effective in preventing the astrogliosis induced by MPTP. Astrocytes undergo a reactive transformation in reaction to insults like central nervous system injury or during the progression of neurodegenerative diseases. This transformation is marked by the enlargement of cellular processes and an increase in intermediate filament proteins like vimentin and GFAP [38]. The neuroprotective effect of honey on astrocytes was also reported by Ali and Kunugi [14], that honey protected astrocytes from oxidative damage possibly via its free radical scavenging and mediation of antioxidant properties.
ROS-induced DNA damage stands out as a notable characteristic of PD as its level is elevated in PD patients and MPTP-induced PD models [39]. The result from this study indicates that honey as well as levodopa pretreatment in the PD models prevented the excessive production of ROS. The overproduction of ROS has the potential to induce tissue damage, triggering an inflammatory response reported to exacerbate PD. MPTP induced the production of ROS as its metabolite methyl-4-phenylpyridinium+ acts by altering the function of ETC while inducing lower expression of antiapoptotic proteins [40]. It was also observed that the inhibitory role of honey on thrombin-induced ROS generation by phagocytes might offer advantages in disrupting the pathological progression of cardiovascular disease [41]. It was further suggested that the possible availability antioxidants provided by topically applying honey has the potential to alleviate the impact of ROS activity in the course of ulcer development [42].
ROS target lipids especially polyunsaturated fatty acids (PUFAs), via lipid peroxidation. The brain contains a lot of PUFAs. MDA has been utilized as a handy marker of lipid peroxidation [43]. The result obtained from the study showed that the MDA concentration of PD-modelled mice was significantly the highest of all the groups indicative that MPTP caused the peroxidation lipids, supporting the findings of high ROS level, this is in line with the report by Liu and colleagues [44] who observed that MPTP induced lipid peroxidation. Furthermore, elevated lipid peroxidation has been observed in the plasma of sporadic PD patients as well as in the brains of vanadium-induced parkinsonism mice [45, 46]. However, honey as well as levodopa pretreatment prevented the lipid peroxidation induced by MPTP, this might be owing to their capability to scavenge or inhibit the free radicals’ production. It was also reported that honey prevented lipid peroxidation induced by lead acetate in rats [20]. Honey supplementation also significantly decreased MDA levels in the pancreas [47] and liver of experimental animals. Levodopa was also reported to inhibit lipid peroxidation in PD patients [45].
GSH maintains homeostasis, by detoxification, forming conjugates with toxicants and inhibiting apoptosis, in addition to its antioxidative role [48]. PD is associated with GSH depletion [49]. Honey and levodopa pretreatment prevented the GSH depletion by MPTP, suggestive of the role of honey and levodopa in the preservation of antioxidants. Moreover, honey and its polyphenols were reported to increase the concentration and activities of GSH and GSH-px respectively. Honey was also reported to be hepatoprotective against alcohol and paracetamol-induced liver damage by increasing the activity of GSH-px while inhibiting lipid peroxidation [50].
SOD controls ROS and RNS levels, thereby minimizing their potential toxicity. Reduced levels of SOD activity have been reported in PD patients [51] and MPTP-induced PD mice models [24]. Honey and levodopa ingestion significantly preserved the SOD activity of the PD mouse model. It was also reported that honey ingestion increased SOD activity in diabetic animal models [52]. Honey was also reported to heal gastric ulcers caused by acetic acid via its antioxidant as well as anti-inflammatory properties [53].
Due to its location at the confluence of the mitochondrial and antioxidant pathways, Nrf2 has become a prospective therapeutic target for PD. Nrf2 controls the endogenous antioxidant response pathway and regulates mitochondria protein expression [54]. A positive correlation exists between increased Nrf2 transcriptional activity and lower risk and delayed onset of PD [55]. Also, the finding that depletion of Nrf2 causes increased α-synuclein accumulation and neuronal death in an α-synuclein overexpressing animal provides more proof that targeting Nrf2 is important in treating PD [56].
Findings from this study indicate that MPTP induced Nrf2 depletion. Nrf2 depletion increases MPTP sensitivity and MPTP-induced dopamine transporter loss in the striatum [57]. However, honey and levodopa pretreatment prevented MPTP-induced Nrf2 depletion, this agrees with the report that honey and chamomile inhibited KEAP1-Nrf2 interaction thereby promoting the production of antioxidants. Additionally, honey boosted cell proliferation, preserved mitochondrial function, triggered the AMPK/Nrf2/ARE signaling pathway, and increased the levels of antioxidant enzymes [58]. Honey also prevented lipopolysaccharide-induced inflammation and oxidative stress by modulating Nrf2 and nuclear factor-κB factors [59]. Thus, honey and levodopa pretreatment prevented Nrf2 depletion thereby increasing GSH, and SOD to combat the increase in the ROS and ultimately preventing cell loss.
In conclusion, honey and levodopa pretreatment was able to preserve the SNpc neurons of MPTP-induced PD model by preventing the alteration of ROS-Nrf2-GSH signaling pathway induced by MPTP (Fig. 6).
Acknowledgements
We hereby acknowledge the Dr. Ijomone, O.M of Neurostein Lab, Ondo, Adeyemo E.A, Emaye M.A and Yussuf R.A. Olaoye J, Jimoh H.B, and Ashir F, for their technical support. The technical team of the central research laboratory, Tanke is also well-appreciated.
Notes
Author Contributions
Conceptualization: FAS, MSA. Data acquisition: FAS, RYI, AI, ALO, ALI, MS, SAB. Data analysis or interpretation: FAS, RYI, AI, ALO, ALI, MS, SAB, REK, GOO, MSA. Drafting of the manuscript: FAS. Critical revision of the manuscript: RYI, AI, ALO, ALI, MS, SAB, REK, GOO, MSA. Approval of the final version of the manuscript: all authors.
References
1. World Health Organization (WHO). 2022. Oct. 1. Ageing and health [Internet]. WHO;Available from: https://www.who.int/news-room/fact-sheets/detail/ageing-and-health. cited 2023 Dec 29.
2. Yang W, Hamilton JL, Kopil C, Beck JC, Tanner CM, Albin RL, Ray Dorsey E, Dahodwala N, Cintina I, Hogan P, Thompson T. 2020; Current and projected future economic burden of Parkinson's disease in the U.S. NPJ Parkinsons Dis. 6:15. DOI: 10.1038/s41531-020-0117-1. PMID: 32665974. PMCID: PMC7347582.
3. Tysnes OB, Storstein A. 2017; Epidemiology of Parkinson's disease. J Neural Transm (Vienna). 124:901–5. DOI: 10.1007/s00702-017-1686-y.
4. Simon DK, Tanner CM, Brundin P. 2020; Parkinson disease epidemiology, pathology, genetics, and pathophysiology. Clin Geriatr Med. 36:1–12. DOI: 10.1016/j.cger.2019.08.002. PMID: 31733690. PMCID: PMC6905381.
5. Bove C, Travagli RA. 2019; Neurophysiology of the brain stem in Parkinson's disease. J Neurophysiol. 121:1856–64. DOI: 10.1152/jn.00056.2019. PMID: 30917059. PMCID: PMC6589717.
6. Kouli A, Torsney KM, Kuan WL. Stoker TB, Greenland JC, editors. Parkinson's disease: etiology, neuropathology, and pathogenesis. Parkinson's disease: pathogenesis and clinical aspects. Codon Publications;2018.
7. Jenner P, Olanow CW. 1996; Oxidative stress and the pathogenesis of Parkinson's disease. Neurology. 47(6 Suppl 3):S161–70. DOI: 10.1212/WNL.47.6_Suppl_3.161S.
8. Magrinelli F, Picelli A, Tocco P, Federico A, Roncari L, Smania N, Zanette G, Tamburin S. 2016; Pathophysiology of motor dysfunction in Parkinson's disease as the rationale for drug treatment and rehabilitation. Parkinsons Dis. 2016:9832839. DOI: 10.1155/2016/9832839. PMID: 27366343. PMCID: PMC4913065.
9. Ball N, Teo WP, Chandra S, Chapman J. 2019; Parkinson's disease and the environment. Front Neurol. 10:218. DOI: 10.3389/fneur.2019.00218.
10. Nag N, Jelinek GA. 2019; A narrative review of lifestyle factors associated with Parkinson's disease risk and progression. Neurodegener Dis. 19:51–9. DOI: 10.1159/000502292. PMID: 31487721.
11. Teodoro AJ. 2019; Bioactive compounds of food: their role in the prevention and treatment of diseases. Oxid Med Cell Longev. 2019:3765986. DOI: 10.1155/2019/3765986. PMID: 30984334. PMCID: PMC6432691.
12. ParkinsonsDisease.net. Dietary supplements for Parkinson's disease [Internet]. ParkinsonsDisease.net.;2017. Mar. 17. cited 2023 Dec 29. Available from: https://parkinsonsdisease.net/treatment/complementary-alternative-supplements.
13. Iranshahy M, Javadi B, Sahebkar A. 2022; Protective effects of functional foods against Parkinson's disease: a narrative review on pharmacology, phytochemistry, and molecular mechanisms. Phytother Res. 36:1952–89. DOI: 10.1002/ptr.7425. PMID: 35244296.
14. Ali AM, Kunugi H. 2019; Bee honey protects astrocytes against oxidative stress: a preliminary in vitro investigation. Neuropsychopharmacol Rep. 39:312–4. DOI: 10.1002/npr2.12079. PMID: 31529692. PMCID: PMC7292328.
15. Kesavan P, Banerjee A, Banerjee A, Murugesan R, Marotta F, Pathak S. Watson RR, Preedy VR, Zibadi S, editors. An overview of dietary polyphenols and their therapeutic effects. Polyphenols: mechanisms of action in human health and disease. Elsevier;2018. p. 221–35. DOI: 10.1016/B978-0-12-813006-3.00017-9.
16. Glick D. Methods of biochemical analysis. Wiley;1954. DOI: 10.1002/9780470110171.
17. Percário S, da Silva Barbosa A, Varela ELP, Gomes ARQ, Ferreira MES, de Nazaré Araújo Moreira T, Dolabela MF. 2020; Oxidative stress in Parkinson's disease: potential benefits of antioxidant supplementation. Oxid Med Cell Longev. 2020:2360872. DOI: 10.1155/2020/2360872. PMID: 33101584. PMCID: PMC7576349.
18. Aznavour N, Cendres-Bozzi C, Lemoine L, Buda C, Sastre JP, Mincheva Z, Zimmer L, Lin JS. 2012; MPTP animal model of Parkinsonism: dopamine cell death or only tyrosine hydroxylase impairment? A study using PET imaging, autoradiography, and immunohistochemistry in the cat. CNS Neurosci Ther. 18:934–41. DOI: 10.1111/cns.12009. PMID: 23106974. PMCID: PMC6493344.
19. Zhou JS, Zhu Z, Wu F, Zhou Y, Sheng R, Wu JC, Qin ZH. 2019; NADPH ameliorates MPTP-induced dopaminergic neurodegeneration through inhibiting p38MAPK activation. Acta Pharmacol Sin. 40:180–91. DOI: 10.1038/s41401-018-0003-0. PMID: 29769744. PMCID: PMC6329815.
20. Abdulmajeed WI, Sulieman HB, Zubayr MO, Imam A, Amin A, Biliaminu SA, Oyewole LA, Owoyele BV. 2016; Honey prevents neurobehavioural deficit and oxidative stress induced by lead acetate exposure in male Wistar rats- a preliminary study. Metab Brain Dis. 31:37–44. DOI: 10.1007/s11011-015-9733-6. PMID: 26435406.
21. Angelopoulou E, Pyrgelis ES, Piperi C. 2020; Neuroprotective potential of chrysin in Parkinson's disease: molecular mechanisms and clinical implications. Neurochem Int. 132:104612. DOI: 10.1016/j.neuint.2019.104612. PMID: 31785348.
22. Lin MW, Lin CC, Chen YH, Yang HB, Hung SY. 2019; Celastrol inhibits dopaminergic neuronal death of Parkinson's disease through activating mitophagy. Antioxidants (Basel). 9:37. DOI: 10.3390/antiox9010037. PMID: 31906147. PMCID: PMC7022523.
23. Adeniyi IA, Babalola KT, Adekoya VA, Oyebanjo O, Ajayi AM, Onasanwo SA. 2023; Neuropharmacological effects of honey in lipopolysaccharide-induced neuroinflammation, cognitive impairment, anxiety and motor impairment. Nutr Neurosci. 26:511–24. DOI: 10.1080/1028415X.2022.2063578. PMID: 35470773.
24. Zhang ZN, Hui Z, Chen C, Liang Y, Tang LL, Wang SL, Xu CC, Yang H, Zhang JS, Zhao Y. 2021; Neuroprotective effects and related mechanisms of echinacoside in MPTP-induced PD mice. Neuropsychiatr Dis Treat. 17:1779–92. DOI: 10.2147/NDT.S299685. PMID: 34113108. PMCID: PMC8184243.
25. Zhao Q, Cai D, Bai Y. 2013; Selegiline rescues gait deficits and the loss of dopaminergic neurons in a subacute MPTP mouse model of Parkinson's disease. Int J Mol Med. 32:883–91. DOI: 10.3892/ijmm.2013.1450.
26. Geldenhuys WJ, Guseman TL, Pienaar IS, Dluzen DE, Young JW. 2015; A novel biomechanical analysis of gait changes in the MPTP mouse model of Parkinson's disease. PeerJ. 3:e1175. DOI: 10.7717/peerj.1175. PMID: 26339553. PMCID: PMC4558067.
27. Li S, Pu XP. 2011; Neuroprotective effect of kaempferol against a 1-methyl-4-phenyl-1,2,3,6-tetrahydropyridine-induced mouse model of Parkinson's disease. Biol Pharm Bull. 34:1291–6. DOI: 10.1248/bpb.34.1291. PMID: 21804220.
28. Mijanur Rahman M, Gan SH, Khalil MI. 2014; Neurological effects of honey: current and future prospects. Evid Based Complement Alternat Med. 2014:958721. DOI: 10.1155/2014/958721. PMID: 24876885. PMCID: PMC4020454.
29. Kotian SR, Bhat KMR, Padma D, Pai KSR. 2019; Influence of traditional medicines on the activity of keratinocytes in wound healing: an in-vitro study. Anat Cell Biol. 52:324–32. DOI: 10.5115/acb.19.009. PMID: 31598362. PMCID: PMC6773891.
30. Hornykiewicz O. Riederer P, Reichmann H, Youdim MBH, Gerlach M, editors. The discovery of dopamine deficiency in the parkinsonian brain. Parkinson's disease and related disorders. Springer Vienna;2006. p. 9–15. DOI: 10.1007/978-3-211-45295-0_3.
31. Gao JX, Li Y, Wang SN, Chen XC, Lin LL, Zhang H. 2019; Overexpression of microRNA-183 promotes apoptosis of substantia nigra neurons via the inhibition of OSMR in a mouse model of Parkinson's disease. Int J Mol Med. 43:209–20. DOI: 10.3892/ijmm.2018.3982.
32. Castelli V, Grassi D, Bocale R, d'Angelo M, Antonosante A, Cimini A, Ferri C, Desideri G. 2018; Diet and brain health: which role for polyphenols? Curr Pharm Des. 24:227–38. DOI: 10.2174/1381612824666171213100449. PMID: 29237377.
33. Yasuda Y, Shimoda T, Uno K, Tateishi N, Furuya S, Yagi K, Suzuki K, Fujita S. 2008; The effects of MPTP on the activation of microglia/astrocytes and cytokine/chemokine levels in different mice strains. J Neuroimmunol. 204:43–51. DOI: 10.1016/j.jneuroim.2008.08.003. PMID: 18817984.
34. O'Callaghan JP, Seidler FJ. 1992; 1-Methyl-4-phenyl-1,2,3,6-tetrahydropyridine (MPTP)-induced astrogliosis does not require activation of ornithine decarboxylase. Neurosci Lett. 148:105–8. DOI: 10.1016/0304-3940(92)90815-O.
35. Serra PA, Sciola L, Delogu MR, Spano A, Monaco G, Miele E, Rocchitta G, Miele M, Migheli R, Desole MS. 2002; The neurotoxin 1-methyl-4-phenyl-1,2,3,6-tetrahydropyridine induces apoptosis in mouse nigrostriatal glia. Relevance to nigral neuronal death and striatal neurochemical changes. J Biol Chem. 277:34451–61. DOI: 10.1074/jbc.M202099200. PMID: 12084711.
36. MacMahon Copas AN, McComish SF, Fletcher JM, Caldwell MA. 2021; The pathogenesis of Parkinson's disease: a complex interplay between astrocytes, microglia, and T lymphocytes? Front Neurol. 12:666737. DOI: 10.3389/fneur.2021.666737. PMID: 34122308. PMCID: PMC8189423.
37. Minden-Birkenmaier BA, Cherukuri K, Smith RA, Radic MZ, Bowlin GL. 2019; Manuka honey modulates the inflammatory behavior of a dHL-60 neutrophil model under the cytotoxic limit. Int J Biomater. 2019:6132581. DOI: 10.1155/2019/6132581. PMID: 30936919. PMCID: PMC6415307.
38. Middeldorp J, Hol EM. 2011; GFAP in health and disease. Prog Neurobiol. 93:421–43. DOI: 10.1016/j.pneurobio.2011.01.005. PMID: 21219963.
39. Manoharan S, Guillemin GJ, Abiramasundari RS, Essa MM, Akbar M, Akbar MD. 2016; The role of reactive oxygen species in the pathogenesis of Alzheimer's disease, Parkinson's disease, and Huntington's disease: a mini review. Oxid Med Cell Longev. 2016:8590578. DOI: 10.1155/2016/8590578. PMID: 28116038. PMCID: PMC5223034.
40. Gluck MR, Krueger MJ, Ramsay RR, Sablin SO, Singer TP, Nicklas WJ. 1994; Characterization of the inhibitory mechanism of 1-methyl-4-phenylpyridinium and 4-phenylpyridine analogs in inner membrane preparations. J Biol Chem. 269:3167–74. DOI: 10.1016/S0021-9258(17)41844-8. PMID: 8106350.
41. Ahmad A, Khan RA, Mesaik MA. 2009; Anti inflammatory effect of natural honey on bovine thrombin-induced oxidative burst in phagocytes. Phytother Res. 23:801–8. DOI: 10.1002/ptr.2648. PMID: 19142984.
42. Hunter M, Kellett J, D'Cunha NM, Toohey K, McKune A, Naumovski N. 2020; The effect of honey as a treatment for oral ulcerative lesions: a systematic review. Explor Res Hypothesis Med. 5:27–37. DOI: 10.14218/ERHM.2019.00029.
43. Ayala A, Muñoz MF, Argüelles S. 2014; Lipid peroxidation: production, metabolism, and signaling mechanisms of malondialdehyde and 4-hydroxy-2-nonenal. Oxid Med Cell Longev. 2014:360438. DOI: 10.1155/2014/360438. PMID: 24999379. PMCID: PMC4066722.
44. Liu C, Kershberg L, Wang J, Schneeberger S, Kaeser PS. 2018; Dopamine secretion is mediated by sparse active zone-like release sites. Cell. 172:706–18.e15. DOI: 10.1016/j.cell.2018.01.008. PMID: 29398114. PMCID: PMC5807134.
45. Agil A, Durán R, Barrero F, Morales B, Araúzo M, Alba F, Miranda MT, Prieto I, Ramírez M, Vives F. 2006; Plasma lipid peroxidation in sporadic Parkinson's disease. Role of the L-dopa. J Neurol Sci. 240:31–6. DOI: 10.1016/j.jns.2005.08.016. PMID: 16219327.
46. Adekeye AO, Irawo GJ, Fafure AA. 2020; Ficus exasperata Vahl leaves extract attenuates motor deficit in vanadium-induced parkinsonism mice. Anat Cell Biol. 53:183–93. DOI: 10.5115/acb.19.205. PMID: 32647086. PMCID: PMC7343565.
47. Erejuwa OO, Sulaiman SA, Ab Wahab MS, Sirajudeen KN, Salleh S, Gurtu S. 2012; Honey supplementation in spontaneously hypertensive rats elicits antihypertensive effect via amelioration of renal oxidative stress. Oxid Med Cell Longev. 2012:374037. DOI: 10.1155/2012/374037. PMID: 22315654. PMCID: PMC3270456.
48. Awasthi YC, Chaudhary P, Vatsyayan R, Sharma A, Awasthi S, Sharma R. 2009; Physiological and pharmacological significance of glutathione-conjugate transport. J Toxicol Environ Health B Crit Rev. 12:540–51. DOI: 10.1080/10937400903358975. PMID: 20183533.
49. Aoyama K, Matsumura N, Watabe M, Nakaki T. 2008; Oxidative stress on EAAC1 is involved in MPTP-induced glutathione depletion and motor dysfunction. Eur J Neurosci. 27:20–30. DOI: 10.1111/j.1460-9568.2007.05979.x. PMID: 18093171.
50. Wang Y, Li D, Cheng N, Gao H, Xue X, Cao W, Sun L. 2015; Antioxidant and hepatoprotective activity of vitex honey against paracetamol induced liver damage in mice. Food Funct. 6:2339–49. DOI: 10.1039/C5FO00345H. PMID: 26084988.
51. Younes-Mhenni S, Frih-Ayed M, Kerkeni A, Bost M, Chazot G. 2007; Peripheral blood markers of oxidative stress in Parkinson's disease. Eur Neurol. 58:78–83. DOI: 10.1159/000103641. PMID: 17565220.
52. Brandes MS, Zweig JA, Tang A, Gray NE. 2021; NRF2 activation ameliorates oxidative stress and improves mitochondrial function and synaptic plasticity, and in A53T α-synuclein hippocampal neurons. Antioxidants (Basel). 11:26. DOI: 10.3390/antiox11010026. PMID: 35052530. PMCID: PMC8772776.
53. Almasaudi SB, Abbas AT, Al-Hindi RR, El-Shitany NA, Abdel-Dayem UA, Ali SS, Saleh RM, Al Jaouni SK, Kamal MA, Harakeh SM. 2017; Manuka honey exerts antioxidant and anti-inflammatory activities that promote healing of acetic acid-induced gastric ulcer in rats. Evid Based Complement Alternat Med. 2017:5413917. DOI: 10.1155/2017/5413917. PMID: 28250794. PMCID: PMC5307292.
54. Esteras N, Dinkova-Kostova AT, Abramov AY. 2016; Nrf2 activation in the treatment of neurodegenerative diseases: a focus on its role in mitochondrial bioenergetics and function. Biol Chem. 397:383–400. DOI: 10.1515/hsz-2015-0295. PMID: 26812787.
55. von Otter M, Landgren S, Nilsson S, Celojevic D, Bergström P, Håkansson A, Nissbrandt H, Drozdzik M, Bialecka M, Kurzawski M, Blennow K, Nilsson M, Hammarsten O, Zetterberg H. 2010; Association of Nrf2-encoding NFE2L2 haplotypes with Parkinson's disease. BMC Med Genet. 11:36. DOI: 10.1186/1471-2350-11-36. PMID: 20196834. PMCID: PMC2843602.
56. Anandhan A, Nguyen N, Syal A, Dreher LA, Dodson M, Zhang DD, Madhavan L. 2021; NRF2 loss accentuates Parkinsonian pathology and behavioral dysfunction in human α-synuclein overexpressing mice. Aging Dis. 12:964–82. DOI: 10.14336/AD.2021.0511. PMID: 34221542. PMCID: PMC8219498.
57. Chen PC, Vargas MR, Pani AK, Smeyne RJ, Johnson DA, Kan YW, Johnson JA. 2009; Nrf2-mediated neuroprotection in the MPTP mouse model of Parkinson's disease: critical role for the astrocyte. Proc Natl Acad Sci U S A. 106:2933–8. DOI: 10.1073/pnas.0813361106. PMID: 19196989. PMCID: PMC2650368.
58. Alvarez-Suarez JM, Giampieri F, Cordero M, Gasparrini M, Forbes-Hernández TY, Mazzoni L, Afrin S, Beltrán-Ayala P, González-Paramás AM, Santos-Buelga C, Varela-Lopez A, Quiles JL, Battino M. 2016; Activation of AMPK/Nrf2 signalling by Manuka honey protects human dermal fibroblasts against oxidative damage by improving antioxidant response and mitochondrial function promoting wound healing. J Funct Foods. 25:38–49. DOI: 10.1016/j.jff.2016.05.008.
59. Ranneh Y, Akim AM, Hamid HA, Khazaai H, Fadel A, Mahmoud AM. 2019; Stingless bee honey protects against lipopolysaccharide induced-chronic subclinical systemic inflammation and oxidative stress by modulating Nrf2, NF-κB and p38 MAPK. Nutr Metab (Lond). 16:15. DOI: 10.1186/s12986-019-0341-z. PMID: 30858869. PMCID: PMC6391794.
Fig. 1
Honey and levodopa pretreatment comparably preserved locomotion (A–C), motor coordination (D), and gait (E, F). a,b,c,dIndicate significant differences compared to PBS, MPTP, levodopa, and honey groups respectively, while *signifies significant difference between honey & MPTP and levodopa & MPTP. All at P<0.05; n=6. PBS, MPTP, levodopa, honey, honey & MPTP, levodopa & MPTP stands for the mice that received, PBS. MPTP, honey, honey & MPTP, and levodopa & MPTP respectively. PBS, phosphate buffered saline; MPTP, 1-methyl-4-phenyl-1,2,3,6-tetrahydropyridine.
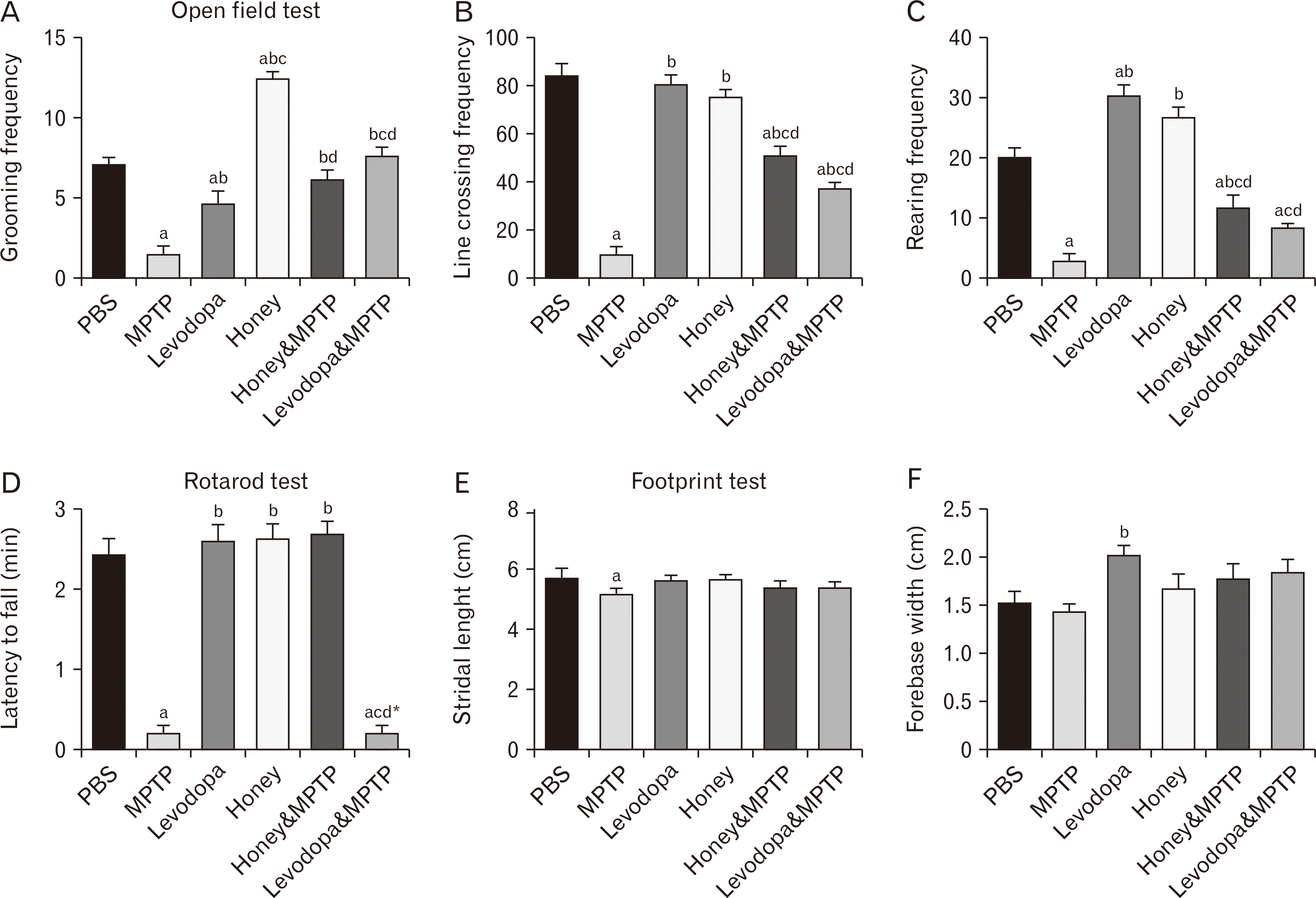
Fig. 2
Nissl substance of SNpc neurons. (A) CFV stained sections of the midbrain (scale bar=10 µm), slide; PBS (PBS group) showed neurons with intact Nissl bodies (black arrows); MPTP (MPTP group) exhibits neurons with pale cytoplasm, indicating Nissl body loss (red arrows); levodopa (levodopa group) displayed neurons with intact Nissl bodies (black arrows); honey (honey group) showed deeply stained neurons with intact Nissl bodies (black arrows); honey & MPTP (honey & MPTP group) depicts mostly intact Nissl bodies (black arrows), with a few lost ones; levodopa & MPTP (levodopa & MPTP group) presents neurons with well-stained cytoplasm indicating intact Nissl bodies (black arrows) and some pale neurons (red arrows). (B) Chromatolytic cell count. A neuron is deemed chromatolytic when it loses its Nissl bodies. a,b,dIndicate significant differences compared to PBS, MPTP, and honey groups respectively, at P<0.05; n=6. PBS, MPTP, levodopa, honey, honey & MPTP, levodopa & MPTP stands for the mice that received, PBS. MPTP, honey, honey & MPTP, and levodopa & MPTP respectively. SNpc, substantia nigra pars compacta; CFV, cresyl fast violet; PBS, phosphate buffered saline; MPTP, 1-methyl-4-phenyl-1,2,3,6-tetrahydropyridine.
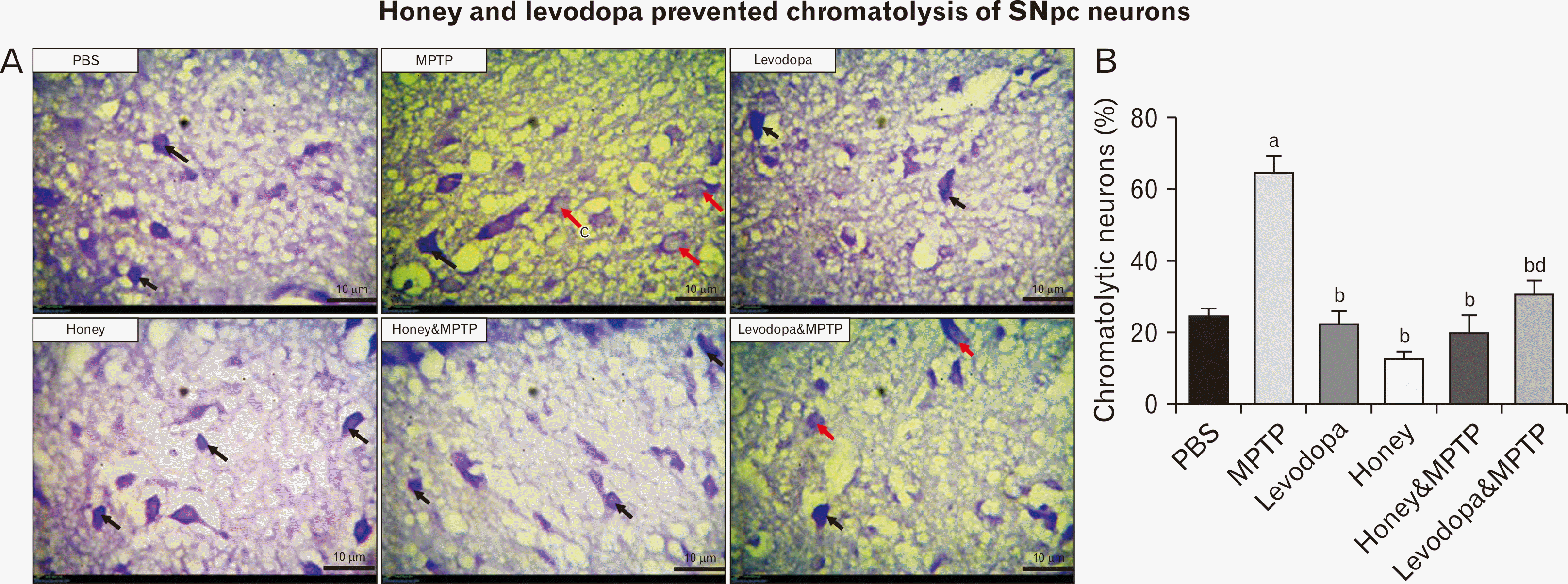
Fig. 3
Honey and levodopa preserved SNpc neurons (A) immunostaining for TH protein (scale bar=50 µm), slide: PBS (PBS group) showed numerous dopaminergic neurons (black arrow). MPTP (MPTP group) exhibited fewer dopaminergic neurons. Levodopa (levodopa group) showed a high number of dopaminergic neurons. Honey (honey group) also had numerous dopaminergic neurons. Honey & MPTP (honey & MPTP group) and levodopa & MPTP (levodopa & MPTP group) demonstrated more dopaminergic neurons than the MPTP slide. (B) TH+ cell count. a,bIndicate significant differences compared to PBS and MPTP groups respectively, at P<0.05; n=6. PBS, MPTP, levodopa, honey, honey & MPTP, levodopa & MPTP stands for the mice that received, PBS. MPTP, honey, honey & MPTP, and levodopa & MPTP respectively. SNpc, substantia nigra pars compacta; TH, tyrosine hydroxylase; PBS, phosphate buffered saline; MPTP, 1-methyl-4-phenyl-1,2,3,6-tetrahydropyridine.
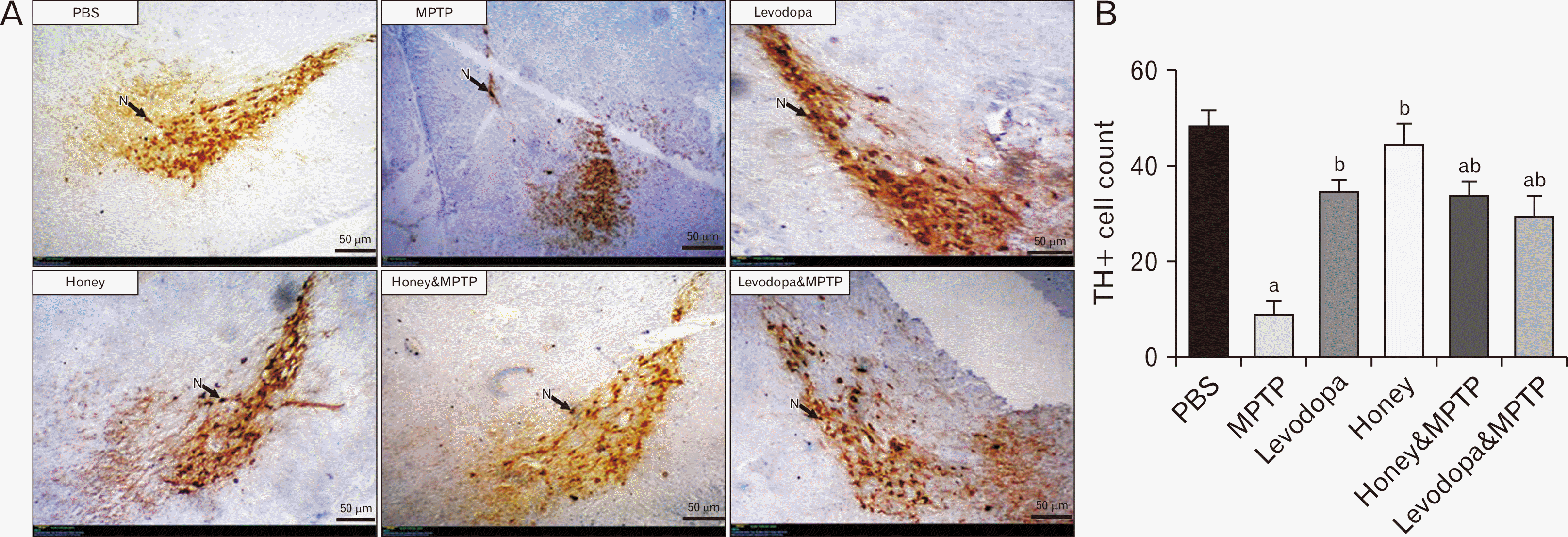
Fig. 4
Astrogliosis of the SNpc. (A) immunostaining for GFAP in the midbrain (scale bar=50 µm), slide: PBS (PBS group) depicted fewer astrocytes; MPTP (MPTP group) exhibited notably numerous reactive astrocytes (red arrows); levodopa (levodopa group) and honey (honey group) showed only a few astrocytes; honey & MPTP (honey & MPTP group) and levodopa & MPTP (levodopa & MPTP group) displayed fewer reactive astrocytes compared to the MPTP group. (B) GFAP+ cell count. The cells identified as astrocytes are GFAP-positive (GFAP+ cells). a,b,dSignify significant differences compared to PBS, MPTP, and honey groups respectively, at P<0.05; n=6. PBS, MPTP, levodopa, honey, honey & MPTP, levodopa & MPTP stands for the mice that received, PBS. MPTP, honey, honey & MPTP, and levodopa & MPTP respectively. SNpc, substantia nigra pars compacta; GFAP, glial fibrillary acidic protein; PBS, phosphate buffered saline; MPTP, 1-methyl-4-phenyl-1,2,3,6-tetrahydropyridine.
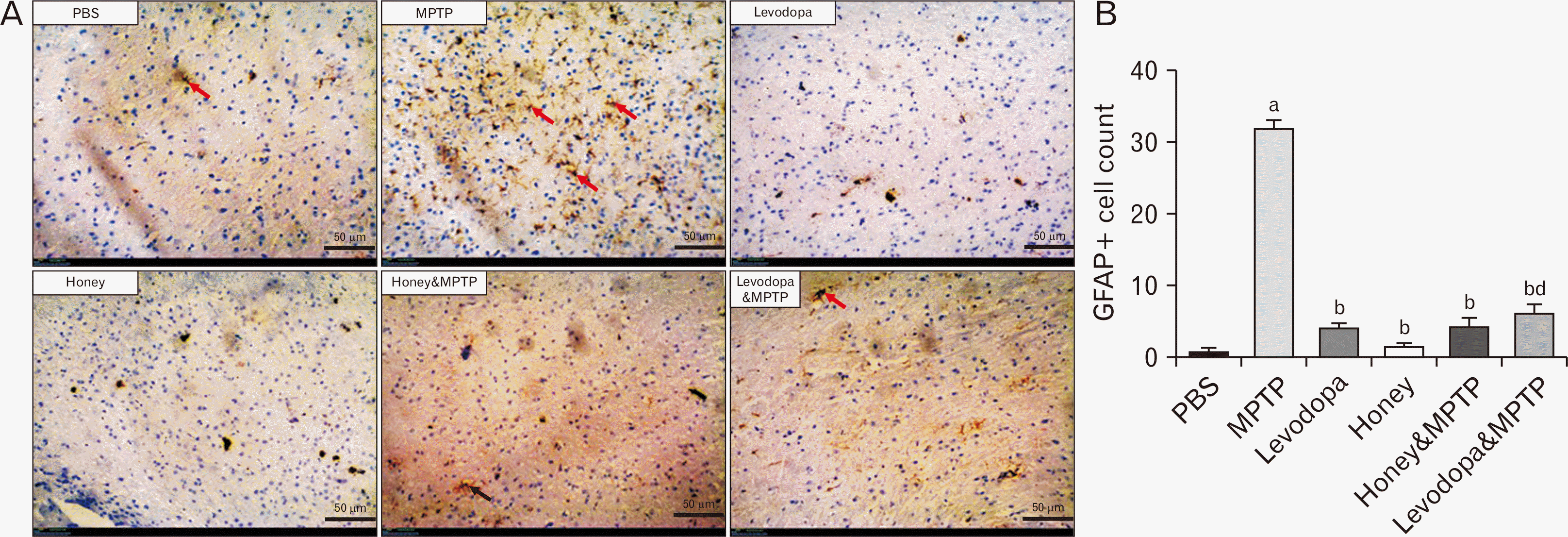
Fig. 5
Honey and levodopa pretreatment comparably prevented oxidative damage (A) oxidative stressors, (B–D) antioxidants, and (E) product of oxidative stress. a,b,c,dSignifies significant differences compared to PBS, MPTP, levodopa, and honey groups respectively, while *signifies significant difference between honey & MPTP and levodopa & MPTP. All at P<0.05; n=6. PBS, MPTP, levodopa, honey, honey & MPTP, levodopa & MPTP stands for the mice that received, PBS. MPTP, honey, honey & MPTP, and levodopa and MPTP respectively. PBS, phosphate buffered saline; MPTP, 1-methyl-4-phenyl-1,2,3,6-tetrahydropyridine.
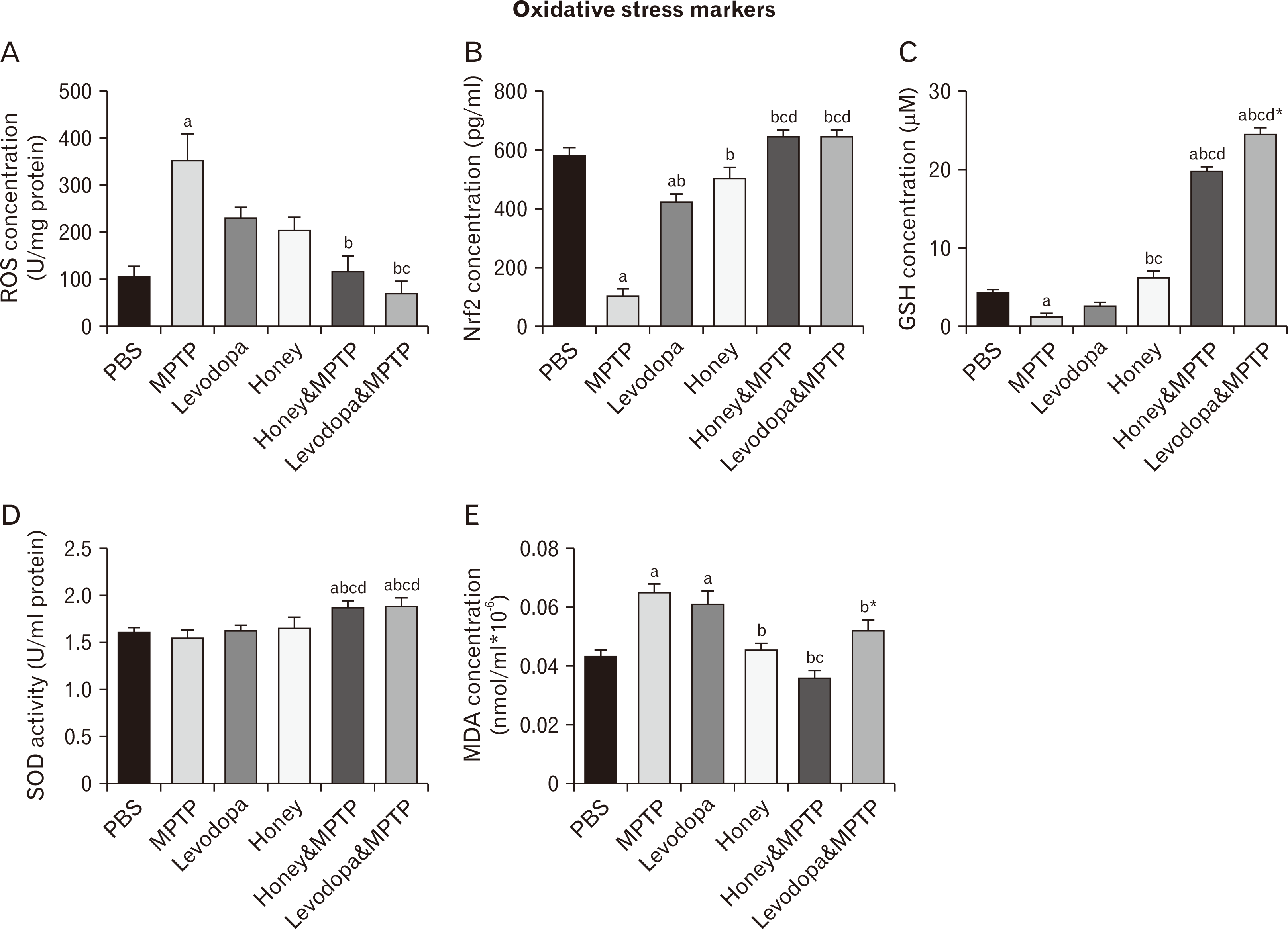
Fig. 6
Graphical summary of the proposed mechanism of action of the neuroprotective effects of honey and levodopa against MPTP-induced PD in adult male Swiss mice. MPTP induced oxidative stress, raising ROS levels. Honey and levodopa possibly activated Nrf2, boosting glutathione production, and countering ROS effects. This reduced malondialdehyde and astrogliosis, preserving SNpc neurons and motor functions. MPTP, 1-methyl-4-phenyl-1,2,3,6-tetrahydropyridine; PD, Parkinson’s disease; ROS, reactive oxygen species; Nrf2, nuclear factor erythroid 2-related factor 2; SNpc, substantia nigra pars compacta; MDA, malondialdehyde; SOD, superoxide dismutase; GSH, glutathione; DA, dopaminergic.
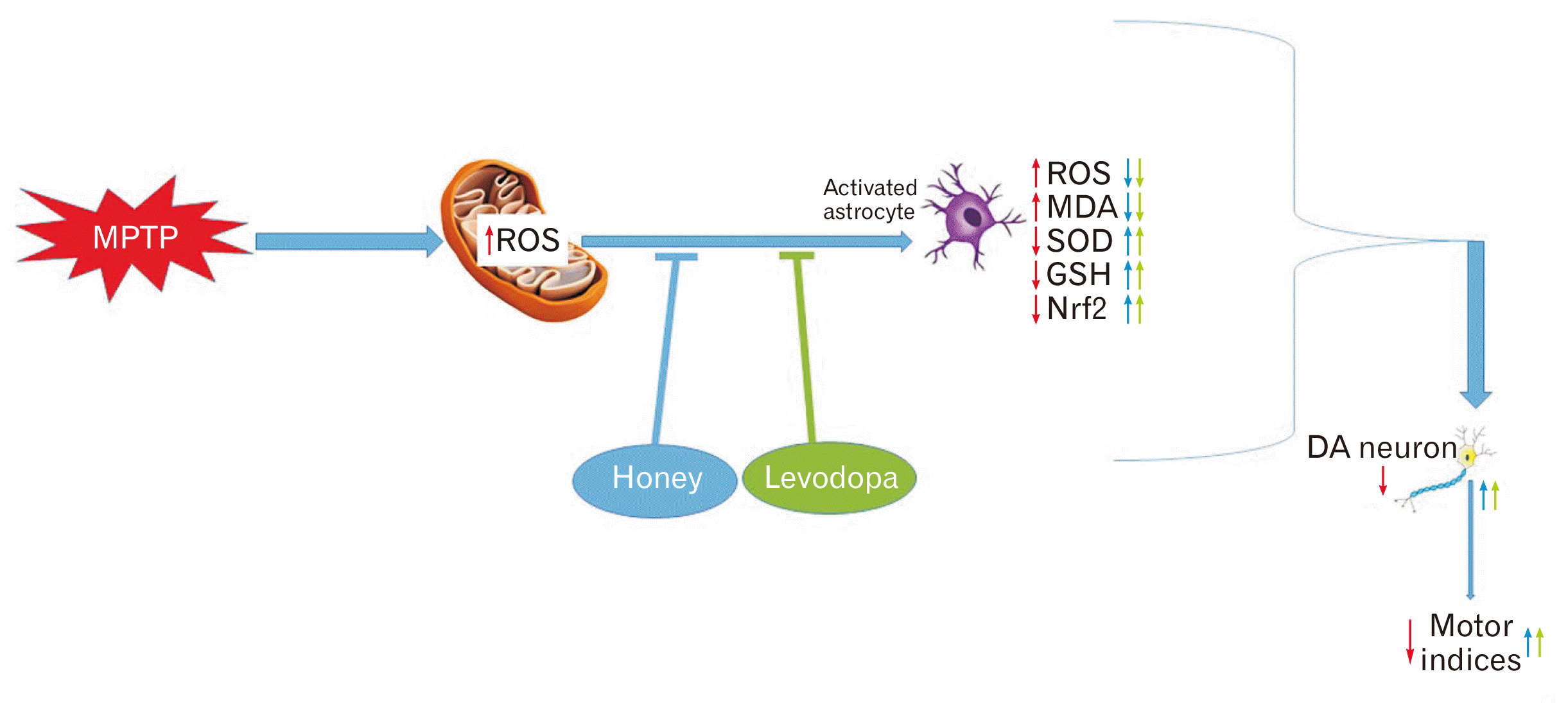