Abstract
Morphological evaluation of the small intestine mucosa and apoptosis activity (caspase-3) is necessary to assess the severity of damage to the small intestine. At the same time, proliferative index based on Ki-67 can be used to assess the regenerative potential of the small intestine. Fragments of small intestine of Wistar rats (n=60) of three groups: I) control (n=20); II) experimental group (n=20; local single electron irradiation at a dose of 2 Gy), III) experimental group (n=20; local single electron irradiation at a dose of 8 Gy) were studied by light microscopy using hematoxylin and eosin staining and immunohistochemical reactions with antibodies to Ki-67 and caspase-3. In all samples of the experimental groups, a decrease in all morphometric indices was observed on day 1 with a tendency to recover on day 3. Small intestinal electron irradiation led to disturbances in the histoarchitecture of varying severity, and an increase in cell apoptosis was observed (increased expression of caspase-3 and decrease in Ki-67). In addition, modulation of the PI3K/AKT and MAPK/ERK signaling pathways was detected. The most pronounced destructive changes were observed in the group of 8 Gy single electron irradiation. Local irradiation of the small intestine with electrons at a dose of 2 and 8 Gy results in a decrease in the number of enterocytes, mainly stem cells of the intestinal crypts.
Digestion and absorption of nutrients occurs in the small intestine, and also provides a barrier function against toxic and immunogenic substances [1]. These functions are performed due to the unique structure of the mucous membrane. However, the small intestinal mucosa is extremely radiosensitive [2, 3].
Currently, radiation is one of the main methods of treating malignant tumors. Radiation therapy can have a damaging effect on the small intestinal mucosa mainly due to depletion of the pool of stem cells in the crypts. Such damage can cause not only abnormalities in tissue structure, but also completely change the structure and function of the small intestine. Despite the availability of data on the morphological features of radiation-induced damage during various types of irradiation, there is very little information about the effect of electrons on the small intestine. Recently, electron irradiation has become more common for intraoperative use and in the treatment of superficial malignancies [4, 5]. New data on the effects of electron irradiation on healthy tissue will help expand understanding of possible applications.
To study the effect of electron irradiation on the morphofunctional state of the epithelium, it is necessary to assess the state of the villi-crypt system: histological and morphometric indicators. The molecular mechanisms of proliferative-apoptotic processes also remain unstudied and require significant additions, so it would be interesting to study the activity of the genes of the PI3K/AKT and MAPK/ERK molecular pathways, which is a classically conserved intracellular signalling pathway that transmits signals which regulate cell proliferation, differentiation, apoptosis and survival [6].
Some studies have shown that radiation exposure occurs through three main pathways that initiate apoptosis: cellular ionization and the formation of reactive oxygen species, which leads to an imbalance of the antioxidant system and damage to DNA molecules (intrinsic pathway); interaction with cell death receptors (extrinsic pathway); and membrane damage [7]. The intrinsic pathway is mediated by the p53 protein, which controls DNA damage and triggers the caspase cascade, which involves initiator (caspase-8, -9, -10) and effector (caspase-3, -6, -7) caspases. Initiator caspases are responsible for the initiation of the cascade and activate effector caspases, which, in turn, ensure cell destruction and death [8]. The extrinsic apoptosis pathway also occurs through the caspase cascade, and membrane damage immediately leads to the activation of caspase-3. It is worth noting that the epithelium of the small intestine has a high proliferative potential. The proliferative index is assessed based on the expression of Ki-67 in the G1, S, G2, and M phases of the cell cycle [9, 10]. The severity of radiation injury correlates with the level of Ki-67 expression, since irradiation, especially at a dose of 8 Gy, leads to arrest of the G2/M phase of the cell cycle and a decrease in cell proliferation. Indicators of the proliferative index can be used not only for diagnosis, but also for further prognosis of radiation injury [11-13].
Thus, based on the assessment of apoptotic and proliferative it is possible to assess the effect of electron irradiation on the functional state of the small intestine epithelium.
Sexually mature Wistar rats (n=60) were divided into two groups: I) control (n=20); II) experimental group (n=20, local single electron irradiation at a dose of 2 Gy), III) experimental group (n=20; local single electron irradiation at a dose of 8 Gy).
The animals were irradiated at the Department of Radiation Biophysics of Russian Research Center named after A.F. Tsyb (Obninsk, Russia) using the Electron beam intraoperative radiation therapy system NOVAC-11 (dose rate 1 Gy/min, energy 10 MeV, frequency 9 Hz, field size=100 mm).
All manipulations were performed according to the “International Recommendations for Biomedical Research Using Animals” (European Economic Community, Strasburg, 1985) and the Declaration of Helsinki of the World Medical Association.
Animal experiments were conducted in strict accordance with the Guidelines for the Care and Use of Laboratory Animals of National Medical Research Radiological Centre of the Ministry of Health of the Russian Federation. The animal experimental protocols used were approved by the Ethics Committee on Animal Experimentation (permit number: AP-153334) no. 22; December 4, 2021 year.
Half of the animals from each group were removed from the experiment on the 1st and 3rd days. Fragments of jejunum were fixed in formalin, embedded in paraffin blocks, sliced on a 3 µm thick microtome, and stained with hematoxylin and eosin. Microscopic analysis was performed using a video microscopy system (Leica DM2000 microscope, Leica ICC50 HD camera; Leica). Morphometric data were obtained using Leica Application Suite, Version 4.9.0, image processing and analysis software. The height of villi and depth of intestinal crypts in 10 fields of view of a light microscope were determined at ×100. For the analysis of the number of goblet cells, 10 random fields of view were chosen that included the entire intestinal villi and crypt. The number of Paneth cells was counted in 10 random fields of view that included small intestinal crypts.
Immunohistochemical analysis was performed according to a standard protocol in automatic mode in a Bond-Max immunohistostainer (Leica). Primary antibodies: to caspase-3 (74T2, 1:50; Invitrogen/Thermo Fisher Scientific) and Ki-67 (ab15580, 1:100; Abcam); secondary - universal antibodies (HiDef DetectionTM HRP Polymer system; Cell Marque). The number of immunopositive cells was counted in 10 fields of view, at magnification ×400. The proliferation index was the number of Ki-67-positive cells per crypt. The data were presented as percentage of positive cells, as the mean±SD.
The obtained data were processed using SPSS 10.0 for Windows statistical software package (IBM Co.) and calculated arithmetic mean, mean error, and Student’s test. Differences between the averages were considered significant at P≤0.05.
The expression of the main members of the MAPK/ERK1,2 signaling pathway (ERK1, ERK2) and the PI3K/AKT signaling pathway (PIK3R1, AKT1, AKT2) was assessed using reverse transcription polymerase chain reaction (RT-PCR). We extracted complementary DNA (cDNA) for all the samples. Small intestinal fragments were placed in a stabilizing solution and stored at –70°C until testing. Samples were homogenized according to standard protocol. Total RNA extraction was carried out using a set of ready-made reagents RNeasy Plus Mini Kit (Qiagen). cDNA synthesis was performed using Super ScriptTM VILOTM Master Mix (Invitrogen). The isolated cDNAs were subjected to RT-PCR using a ready-made mixture of ABsolute Blue QPCR Mix reagents (Thermo Scientific) with SYBR Green I. RT-PCR was carried out using the Step One System (Applied Biosystems) and standard software. Gene expression analysis was performed using the threshold cycle (Ct) method and calculating relative gene expression according to the protocol. Control was performed against the housekeeping reference gene GAPDH. The selection of primers was carried out based on publicly available materials on DNA and mRNA sequences of genes in the National Center for Biotechnology Information database using Primer-BLAST (Table 1).
In the jejunum of the control group on days 1 and 3, no signs of inflammation or histoarchitectural disorders were detected. Paneth cells are secretory cells located in the crypts of Lieberkühn, adjacent to intestinal stem cells.
In all jejunal samples irradiated with an electron dose of 2 Gy (group II), on day 1 there was a slight decrease in the number of goblet cells in the epithelium of the villi and intestinal crypts and a decrease in Paneth cells compared to the control group. A weak inflammatory infiltration was observed in the lamina propria of the mucous membrane. On the contrary, on the 3rd day the number of goblet cells and Paneth cells approached control values (Table 2, Fig. 1).
When examining samples of group III 1 day after irradiation with a dose of 8 Gy (group III), moderate lymphocytic infiltration of the inner lamina and mucous membrane was revealed. The mucous membrane is thickened due to tissue swelling. The number of goblet cells and Paneth cells decreased by 1.37 and 1.57 times, respectively, compared to the control group. In the mucous membrane, the villus-crypt ratio was disrupted due to a significant decrease in the number and height of intestinal villi, and peeling of the epithelium was observed. On the 3rd day after irradiation, an increase and expansion of intestinal crypts was observed due to an active regenerative process - restoration of the number of goblet cells and Paneth cells (Table 2), as well as the disappearance of tissue edema. Moderate lymphocytic infiltration was preserved in the inner lamina of the mucous membrane (Table 2, Fig. 1).
When assessing the height of intestinal villi and the depth of intestinal crypts, it was found that in group II the mean height of villi corresponded to control values at all-time points. The depth of crypts decreased at first and recovered to control values on the 3rd day (Table 3, Fig. 2). In group III, we found a sharp decrease in morphometric parameters in the villus-crypt system. One day after irradiation the height of villi decreased 1.9-fold, and after 3 days - 1.8-fold compared to the control group. Similar reductions were observed when measuring crypt depth: after 1 day - 2.5 times, after 3 days - 2.3 times (Table 3, Fig. 2).
The immunohistochemical study showed a pattern of predominance of apoptotic processes over proliferative ones on 1 day after the experiment. In group II, the number of caspase-3-positive cells was increased 3.0-fold (6.34%±2.17% vs. 2.21%±0.87%). In contrast, the proliferative index assessed by Ki-67 expression decreased 1.2-fold relative to the control group (72.94%±8.09% vs. 89.83%±9.41%) (Table 4, Fig. 3).
On day 3, the beginning of regenerative processes was noted: the number of caspase-3-positive cells decreased and the proliferation index increased. In group II, the number of caspase-3-positive cells was increased 2.0-fold (4.11%±1.79% vs. 2.21%±0.87%) relative to the control group. The proliferative index assessed by Ki-67 expression remained 1.1-fold lower relative to the control group (80.11%±8.23% vs. 89.83%±9.41%) (Table 4, Fig. 3).
After irradiation with 8 Gy on day 1 after local electron irradiation, the number of caspase-3-positive enterocytes was increased 5.6-fold relative to group I. On day 3, the number of caspase-3-positive cells did not statistically differ from the control values (P>0.05).
The proportion of Ki-67-positive enterocytes in the experimental group was 1.9 times lower in 1 day after the radiation, and 1.4 times lower on day 3 compared to the control group (Table 4, Fig. 3).
Using RT-PCR, we studied the activity of genes in the PI3K/AKT and MAPK/ERK signaling pathways. In the small intestinal, 3 days after electron irradiation at a dose of 8 Gy, a statistically significant decrease in the relative expression of the genes PIK3R1, AKT1, AKT2, ERK1 and ERK2 was found compared to the control group. After 2 Gy electron irradiation the expression of the above genes did not differ from the control group, but was statistically significantly higher than in group III (Fig. 4).
The present work is devoted to the study of morphofunctional changes in the villus-crypt system and the features of proliferation and apoptosis of the small intestine epithelium after electron irradiation at a dose of 2 Gy and 8 Gy. Comparative analysis was performed at two time points, taking into account cycles of jejunal epithelium proliferation [14, 15].
Decrease in intestinal villi height and intestinal crypt depth observed 24 hours after electron irradiation was due to reduction of epithelium, as apical enterocytes and stem cells are extremely sensitive to exposure of radiation. From the microenvironment (bystander effect), local inflammatory reactions in the form of interstitial edema and lymphocytic-histiocytic infiltration were noted [16, 17]. Changes in jejunum the villus-crypt system led to impaired cavity, parietal and membrane digestion and decreased barrier function.
In 3 days after electron irradiation, a complete recovery of the epithelium of the jejunum in the experimental group was observed, indicating the activation of regenerative-proliferative processes. It is known that proliferative activity of jejunum depends on crypt depth since stem cells differentiation and their further migration occur here. So, the crypt depth can be considered as a predictor of radiation-induced damage degree [2, 18].
To better understand the processes of damage and repair, we investigated markers characterizing proliferation and apoptosis. To study apoptosis, we examined the level of caspase-3. During immunohistochemistry-research, a significant increase in caspase-3 expression predominantly in small intestinal epithelial stem cells after local electron irradiation was observed on day 1. Caspase-3 is the main effector caspase that triggers cell destructurization, indicating a terminal stage of apoptosis in immunopositive enterocytes [7, 19]. Nevertheless, it is worth noting that the number of apoptotic cells during electron irradiation is significantly lower than during irradiation of experimental animals with a similar dose by other types of irradiations, which indicates its less damaging effect [20, 21].
The proliferative index (mitotic potential) was assessed by Ki-67 labeling. A significant decrease in the number of Ki-67-immunopositive cells on the first day indicates the inability of irradiated cells to enter further phases of the cell cycle [22-24]. It is worth noting that the decrease in the number of immunopositive cells is associated with shortening of the intestinal crypts. That is, stem cells are the most radiosensitive to electron irradiation. However, by day 3, the number of Ki-67-immunopositive enterocytes was fully restored after 2 Gy irradiation and partially - after 8 Gy.
Thus, it should be noted that electron irradiation has a significant dose-dependent effect. Complete recovery of all morphometric and immunohistochemical parameters on the 3rd day after irradiation at a dose of 2 Gy suggests that stem cells are able to provide rapid regeneration of the epithelium within one cycle of renewal: 3 to 5 days. When irradiated at a dose of 8 Gy there is a more significant depletion of the pool of stem cells, which requires a longer period of regeneration of the epithelium. These morphometric and immunohistochemical parameters must be considered when creating clinical guidelines and choosing the radiation dose. In addition, it is necessary to study the effect of radioprotective drugs [12, 13].
As a result of our study, we found that intestinal crypt stem cells have the greatest radiosensitivity to electron irradiation by caspase-3-mediated apoptosis. However, irradiation with electrons at a dose of 2 and 8 Gy does not lead to irreversible disorders in the structure and function of the small intestine, which allows the epithelium to recover in minimal time after irradiation.
We also found that the activity of the PI3K/AKT and MAPK/ERK signaling pathway genes correlates with the radiation dose. This may be due to cell death as a result of exposure to electron irradiation. However, it is possible that these pathways are the first to respond to electron irradiation in the small intestine. Similar results were obtained by other authors [25].
In conclusion, local irradiation of the small intestine with electrons at a dose of 2 and 8 Gy results in a decrease in the number of enterocytes, mainly stem cells of the intestinal crypts. In the study of proliferative-apoptotic processes on the 1st day there was a shift towards apoptosis, with restoration of proliferation on the 3rd day.
Notes
Author Contributions
Conceptualization: GD, Dmitrii Atiakshin. Data acquisition: EK, SS, Daniia Ashgalieva. Data analysis or interpretation: UB, ME, OS, Daniil Kovalev. Drafting of the manuscript: VS, EE, Darya Kabanova. Critical revision of the manuscript: GD. Approval of the final version of the manuscript: all authors.
References
1. Collins JT, Nguyen A, Badireddy M. Anatomy, abdomen and pelvis, small intestine. StatPearls Publishing;2024.
2. Park HS, Goodlad RA, Ahnen DJ, Winnett A, Sasieni P, Lee CY, Wright NA. 1997; Effects of epidermal growth factor and dimethylhydrazine on crypt size, cell proliferation, and crypt fission in the rat colon. cell proliferation and crypt fission are controlled independently. Am J Pathol. 151:843–52. PMID: 9284833. PMCID: PMC1857833.
3. Livanova AA, Fedorova AA, Zavirsky AV, Bikmurzina AE, Krivoi II, Markov AG. 2021; Dose and time dependence of functional impairments in rat jejunum following ionizing radiation exposure. Physiol Rep. 9:e14960. DOI: 10.14814/phy2.14960. PMID: 34337895. PMCID: PMC8326886.
4. Moraes FY, Carvalho Hde A, Hanna SA, Silva JL, Marta GN. 2013; Literature review of clinical results of total skin electron irradiation (TSEBT) of mycosis fungoides in adults. Rep Pract Oncol Radiother. 19:92–8. DOI: 10.1016/j.rpor.2013.08.003. PMID: 24936326. PMCID: PMC4054998.
5. Barney BM, Petersen IA, Dowdy SC, Bakkum-Gamez JN, Klein KA, Haddock MG. 2013; Intraoperative electron beam radiotherapy (IOERT) in the management of locally advanced or recurrent cervical cancer. Radiat Oncol. 8:80. DOI: 10.1186/1748-717X-8-80. PMID: 23566444. PMCID: PMC3641982.
6. Brzezianska E, Pastuszak-Lewandoska D. 2011; A minireview: the role of MAPK/ERK and PI3K/Akt pathways in thyroid follicular cell-derived neoplasm. Front Biosci (Landmark Ed). 16:422–39. DOI: 10.2741/3696. PMID: 21196179.
7. Olsson M, Zhivotovsky B. 2011; Caspases and cancer. Cell Death Differ. 18:1441–9. DOI: 10.1038/cdd.2011.30. PMID: 21455218. PMCID: PMC3178435.
8. Beroske L, Van den Wyngaert T, Stroobants S, Van der Veken P, Elvas F. 2021; Molecular Imaging of apoptosis: the case of caspase-3 radiotracers. Int J Mol Sci. 22:3948. DOI: 10.3390/ijms22083948. PMID: 33920463. PMCID: PMC8069194.
9. Gerdes J, Schwab U, Lemke H, Stein H. 1983; Production of a mouse monoclonal antibody reactive with a human nuclear antigen associated with cell proliferation. Int J Cancer. 31:13–20. DOI: 10.1002/ijc.2910310104. PMID: 6339421.
10. Ishibashi N, Maebayashi T, Aizawa T, Sakaguchi M, Nishimaki H, Masuda S. 2017; Correlation between the Ki-67 proliferation index and response to radiation therapy in small cell lung cancer. Radiat Oncol. 12:16. DOI: 10.1186/s13014-016-0744-1. PMID: 28086989. PMCID: PMC5237196.
11. Keam S, MacKinnon KM, D'Alonzo RA, Gill S, Ebert MA, Nowak AK, Cook AM. 2022; Effects of photon radiation on DNA damage, cell proliferation, cell survival, and apoptosis of murine and human mesothelioma cell lines. Adv Radiat Oncol. 7:101013. DOI: 10.1016/j.adro.2022.101013. PMID: 36420194. PMCID: PMC9677206.
12. Akedo I, Ishikawa H, Ioka T, Kaji I, Narahara H, Ishiguro S, Suzuki T, Otani T. 2001; Evaluation of epithelial cell proliferation rate in normal-appearing colonic mucosa as a high-risk marker for colorectal cancer. Cancer Epidemiol Biomarkers Prev. 10:925–30. PMID: 11535542.
13. Andrés-Sánchez N, Fisher D, Krasinska L. 2022; Physiological functions and roles in cancer of the proliferation marker Ki-67. J Cell Sci. 135:jcs258932. DOI: 10.1242/jcs.258932. PMID: 35674256.
14. Blanpain C, Horsley V, Fuchs E. 2007; Epithelial stem cells: turning over new leaves. Cell. 128:445–58. DOI: 10.1016/j.cell.2007.01.014. PMID: 17289566. PMCID: PMC2408375.
15. Gordon JI, Hermiston ML. 1994; Differentiation and self-renewal in the mouse gastrointestinal epithelium. Curr Opin Cell Biol. 6:795–803. DOI: 10.1016/0955-0674(94)90047-7. PMID: 7880525.
16. Marín A, Martín M, Liñán O, Alvarenga F, López M, Fernández L, Büchser D, Cerezo L. 2014; Bystander effects and radiotherapy. Rep Pract Oncol Radiother. 20:12–21. DOI: 10.1016/j.rpor.2014.08.004. PMID: 25535579. PMCID: PMC4268598.
17. Najafi M, Fardid R, Hadadi G, Fardid M. 2014; The mechanisms of radiation-induced bystander effect. J Biomed Phys Eng. 4:163–72. PMID: 25599062. PMCID: PMC4289523.
18. Potten CS, Owen G, Roberts SA. 1990; The temporal and spatial changes in cell proliferation within the irradiated crypts of the murine small intestine. Int J Radiat Biol. 57:185–99. DOI: 10.1080/09553009014550431. PMID: 1967288.
19. Zhou M, Liu X, Li Z, Huang Q, Li F, Li CY. 2018; Caspase-3 regulates the migration, invasion and metastasis of colon cancer cells. Int J Cancer. 143:921–30. DOI: 10.1002/ijc.31374. PMID: 29524226. PMCID: PMC6204286.
20. Potten CS, Grant HK. 1998; The relationship between ionizing radiation-induced apoptosis and stem cells in the small and large intestine. Br J Cancer. 78:993–1003. DOI: 10.1038/bjc.1998.618. PMID: 9792141. PMCID: PMC2063142.
21. Miyoshi-Imamura T, Kakinuma S, Kaminishi M, Okamoto M, Takabatake T, Nishimura Y, Imaoka T, Nishimura M, Murakami-Murofushi K, Shimada Y. 2010; Unique characteristics of radiation-induced apoptosis in the postnatally developing small intestine and colon of mice. Radiat Res. 173:310–8. DOI: 10.1667/RR1905.1. PMID: 20199216.
22. Seidal T, Edvardsson H. 1999; Expression of c-kit (CD117) and Ki67 provides information about the possible cell of origin and clinical course of gastrointestinal stromal tumours. Histopathology. 34:416–24. DOI: 10.1046/j.1365-2559.1999.00643.x. PMID: 10231416.
23. Cheng Y, Dong Y, Hou Q, Wu J, Zhang W, Tian H, Li D. 2019; The protective effects of XH-105 against radiation-induced intestinal injury. J Cell Mol Med. 23:2238–47. DOI: 10.1111/jcmm.14159. PMID: 30663222. PMCID: PMC6378229.
24. Li LT, Jiang G, Chen Q, Zheng JN. 2015; Ki67 is a promising molecular target in the diagnosis of cancer (review). Mol Med Rep. 11:1566–72. DOI: 10.3892/mmr.2014.2914. PMID: 25384676.
25. Lu L, Li W, Chen L, Su Q, Wang Y, Guo Z, Lu Y, Liu B, Qin S. 2019; Radiation-induced intestinal damage: latest molecular and clinical developments. Future Oncol. 15:4105–18. DOI: 10.2217/fon-2019-0416. PMID: 31746639.
Fig. 1
Villus and crypts in the control and experimental groups. Staining with hematoxylin and eosin, magnification ×200.
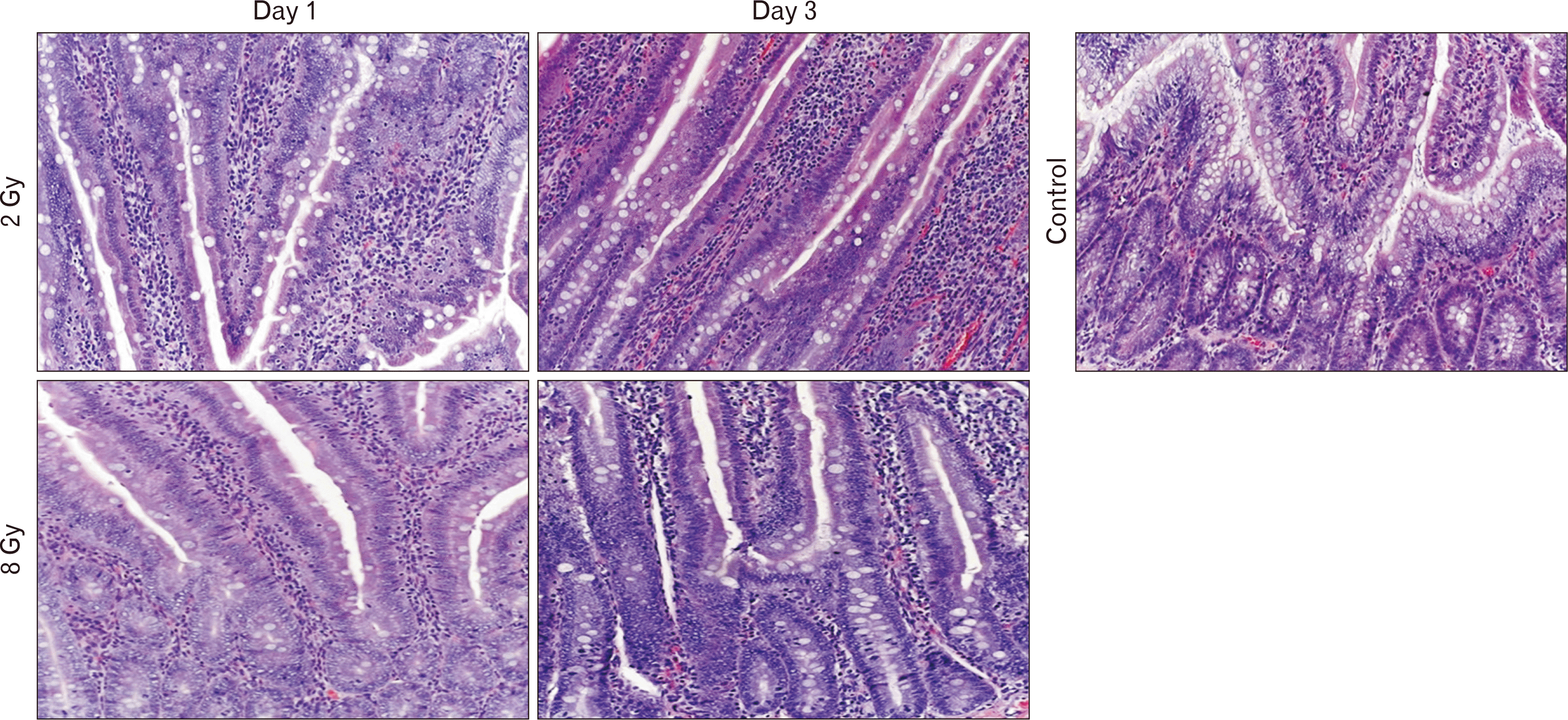
Fig. 2
Morphometric evaluation of villus height (black arrow) and crypt depth (yellow arrow) in the experimental groups. Staining with hematoxylin, magnification ×200.

Fig. 3
The results of immunohistochemical study of the small intestine after electron irradiation at a dose of 2 Gy. Staining with hematoxylin, magnification ×200.
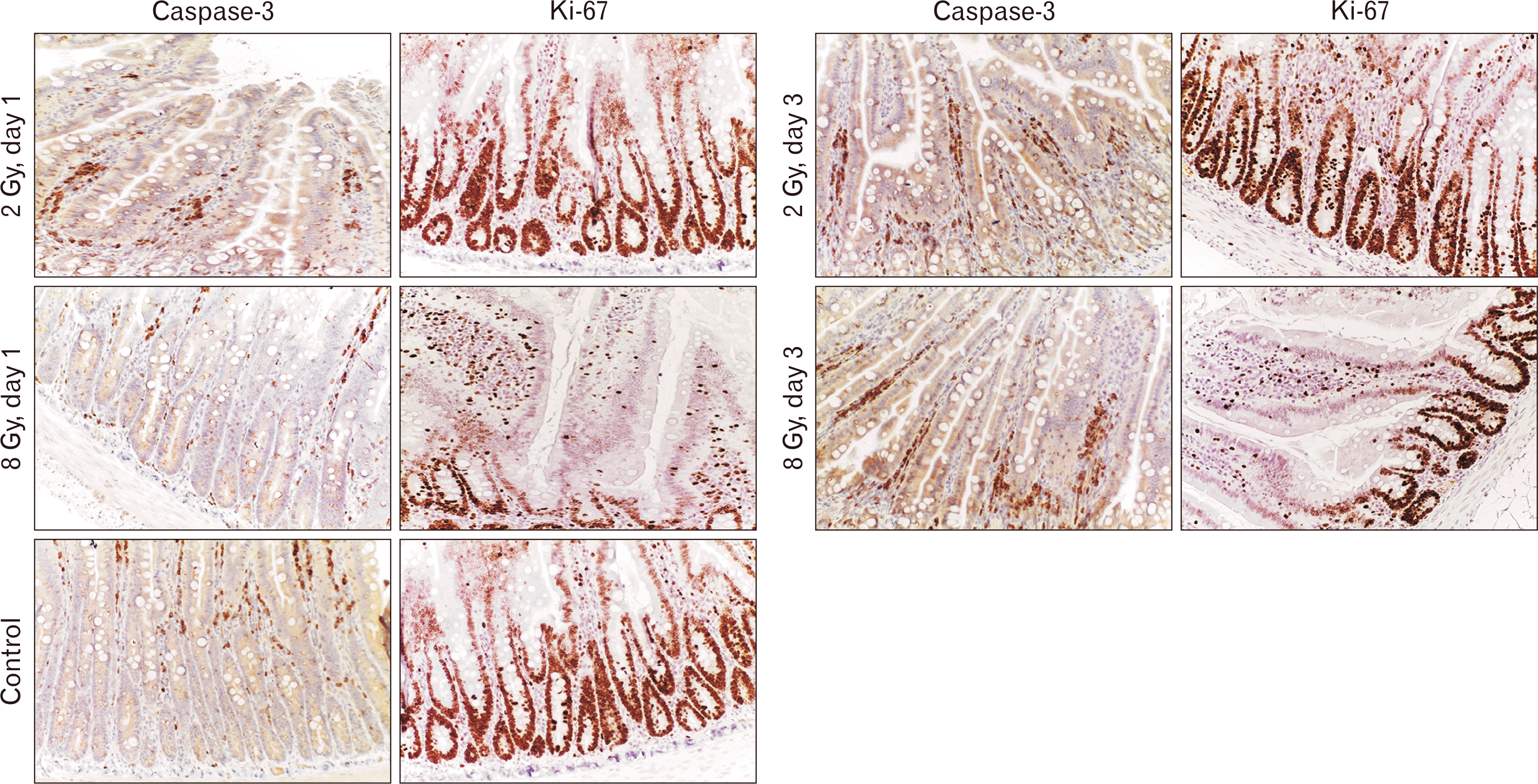
Fig. 4
Expression of genes of the PI3K/AKT and MAPK/ERK signaling pathways in the small intestinal of rats three days after local electron irradiation at a dose of 8 Gy and a dose of 2 Gy. The abscissa axis shows the studied genes in the control and experimental groups; the ordinate axis shows the relative gene expression. Data are presented as means and confidence intervals. Statistically significant differences between groups; P<0.01.
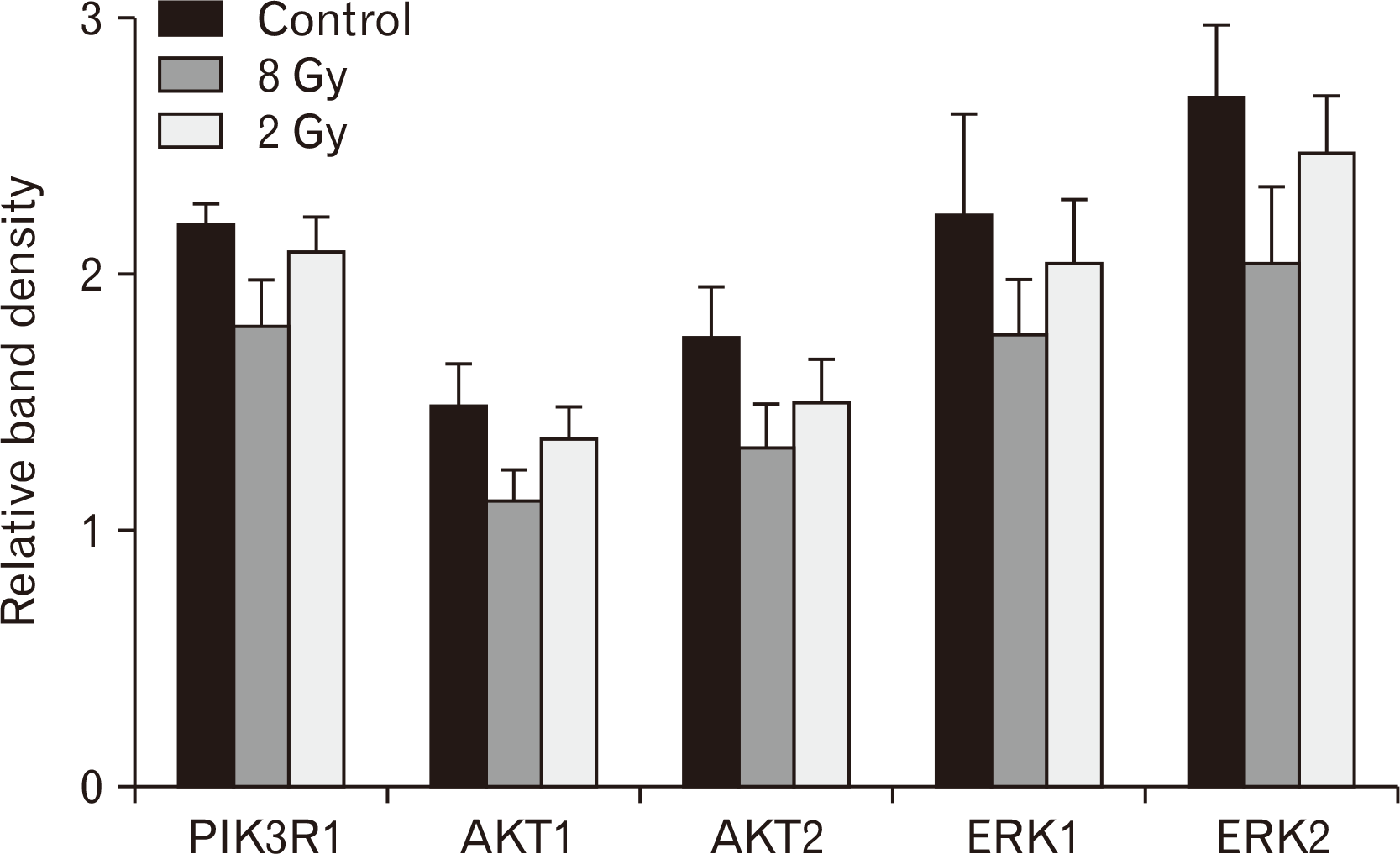
Table 1
Reverse transcription polymerase chain reaction primer sequence
Table 2
Results of histomorphometric studies of villus height and crypt depth in the control and experimental groups
2 Gy (II) | 8 Gy (III) | Control (I) | ||||
---|---|---|---|---|---|---|
Day 1 | Day 3 | Day 1 | Day 3 | |||
Goblet cells | 24.47±1.19 | 26.12±1.27 | 19.23±0.89b) | 23.57±1.36 | 26.34±1.38 | |
Paneth cells | 2.68±0.22a) | 2.97±0.17 | 1.96±0.33b) | 2.72±0.43 | 3.07±0.23 |
Table 3
Results of morphometric study of intestinal villi height and depth of intestinal crypts in the control and experimental groups
Group | Time | Villus height (μm) | Crypts depth (μm) |
---|---|---|---|
Control (I) | Day 1 | 380.9±52 | 135.4±16 |
Day 3 | 371.6±46 | 126.2±13 | |
2 Gy (II) | Day 1 | 353.1±43 | 101.4±10a) |
Day 3 | 363.4±37 | 119.7±15 | |
8 Gy (III) | Day 1 | 203.4±52b) | 50.3±27b) |
Day 3 | 212.3±63b) | 54.7±31b) |