Abstract
Background
Pronase pretreatment can reduce rituximab (RTX) interference by degrading CD20 in B-cell flow cytometry crossmatch (FCXM) testing. However, it may also reduce the assay sensitivity by degrading HLA molecules. We investigated the effects of various pronase concentrations on RTX interference and the analytical sensitivity of B-cell FCXM testing.
Methods
Using 59 patient serum samples and 38 donor lymphocyte samples, we designed 97 recipient–donor pairs and divided them into three groups according to RTX use and the presence of weak-to-moderate donor HLA-specific antibody (DSA) reactions RTX+/DSA−, RTX+/DSA+, and RTX−/DSA+. FCXM was performed after pretreating lymphocytes with six different pronase concentrations (0, 0.5, 1, 2, 3, and 4 mg/mL).
Results
With B-FCXM testing, false-positive results due to RTX in the RTX+/DSA– group markedly decreased with increasing pronase concentrations. The median channel shift values in the RTX+/DSA+ and RTX−/DSA+ groups did not significantly decrease when the pronase concentration was increased from 1 mg/mL to 2 or 3 mg/mL. All eight RTX+/DSA+ cases that were positive at 1 mg/mL pronase but negative at 2 or 3 mg/mL had mean fluorescence intensity (MFI) DSA values of less than 3,000 except for DQ5 (MFI 5,226). With T-cell FCXM, false-positive results were observed in 2.9% of 315 FCXM tests with pronase pretreatment.
Conclusions
Higher concentrations (2 or 3 mg/mL) of pronase effectively eliminated RTX interference but still carried a risk for false negativity for weak DSA reactions in B-cell FCXM. Higher pronase concentrations can be used as an auxiliary method to detect moderate-to-strong DSA reactions in RTX-treated patients.
With organ transplantation, crossmatch testing is used to detect donor-specific antibodies in the recipient’s serum against HLA molecules expressed on the donor’s lymphocytes [1]. Flow cytometry crossmatch (FCXM) testing is more sensitive than complement-dependent cytotoxicity crossmatching and has demonstrated clinical utility in predicting graft rejection [2, 3]. However, owing to the technical nature of the FCXM assay (which utilizes an anti-human IgG secondary antibody), it is susceptible to interference from monoclonal antibody therapeutics used to treat patients undergoing organ transplantation [4-6]. For example, rituximab (RTX) in recipient serum binds to the CD20 antigen on donor B cells and reacts with fluorescently labeled anti-human IgG secondary antibodies, which generates false-positive results [7].
Pronase is a nonspecific protease that eliminates nonspecific immunoglobulin binding to B cells during FCXM [8, 9]. This technique is believed to be beneficial because pronases degrade Fc gamma receptors more quickly than other proteins, such as CD19, CD20, MHC class I, and MHC class II [10]. Some investigators have proposed using 1 mg/mL pronase to degrade CD20 on donor lymphocytes and eliminate interference by RTX-supplemented serum [7]. However, recent findings suggested that RTX accounts for most false-positive results in B-cell FCXM with 1 mg/mL pronase pretreatment [11]. Higher concentrations of pronase are needed, but this change can lead to HLA degradation and may cause false-negative FCXM results [10]. The potential for false-negative results due to higher pronase concentrations used during treatment should be thoroughly evaluated when performing FCXM for patients with weak-to-moderate levels of donor HLA-specific antibodies (DSAs).
We evaluated the ability of various pronase concentrations to eliminate RTX interference during B-cell FCXM testing for patients undergoing RTX treatment for desensitization. We also evaluated whether higher pronase concentrations (>1 mg/mL) might cause false-negative FCXM results in patients with weak-to-moderate DSA levels.
In contrast to B-cell FCXM, pronase treatment can cause false-positive results in T-cell FCXM, indicating that caution should be exercised in interpreting T-cell FCXM results when using pronase-treated lymphocytes [12]. Such false-positive results are thought to reflect the exposure of cryptic epitopes during enzymatic digestion and subsequent binding with non-HLA antibodies [12-14]. However, the effect of various concentrations of pronase on T-cell FCXM has not been comprehensively evaluated. Therefore, we also evaluated the effect of pronase treatment on T-cell FCXM testing.
From July 2022 to January 2023, we sequentially enrolled 38 lymphocyte donors who underwent HLA typing at Seoul National University Hospital, Seoul, Korea. We then screened the sera as follows: first, we recruited 23 patients who underwent kidney transplants, received RTX, and were referred to our department for desensitizing plasmapheresis as candidates between January 2017 and September 2022. The donor cells and RTX-treated sera were matched and found to have weak-to-moderate DSA levels, including specificities for HLA-A, HLA-B, and HLA-DR, with a frequency of >5% in Koreans. For HLA-DQ analysis, serum samples were spiked with RTX that only had anti-DQ antibodies due to the challenge of finding sera exposed to RTX treatment and positive for anti-DQ DSAs. These recipient–donor pairs displaying both RTX and DSA reactivity against donor HLAs were categorized into the RTX+/DSA+ group. DSAs with the same HLA specificity, similar mean fluorescence intensities (MFIs), and no history of RTX were then sought in cryopreserved sera with panel reactive antibody results from January 2017 to September 2022. These patients were categorized into the RTX−/DSA+ group. Subsequently, we searched for a group that had a similar number of days after RTX treatment as the RTX+/DSA+ group but did not have DSA (RTX+/DSA– group). Finally, we selected 59 cryopreserved serum samples from 54 patients who underwent HLA antibody identification with the LABScreen Single Antigen Assay (One Lambda, Canoga Park, CA, USA) at Seoul National University Hospital from January 2017 to September 2022 for use in this study. Of the 23 patients treated with RTX, 18 received RTX at a dose of 375 mg/m2 body surface area owing to HLA incompatibility, and five received 200 mg owing to ABO incompatibility. The median time from RTX administration to blood draw was 29 days (range: 11–42 days). Fifty-nine serum samples were used for FCXM testing either as is (N=50) or after spiking with RTX (Mabthera, Roche, Basel, Switzerland; 100 μg/mL) in cases where the patients had not undergone RTX treatment (N=9).
Peripheral blood mononuclear cells (PBMCs) were isolated from 38 donors using the Ficoll–Hypaque density-gradient method. By combining 59 serum samples and 38 donor cell samples, we designed 97 recipient–donor pairs and categorized them into three groups: RTX+/DSA– (patients with RTX treatment and no DSAs against the paired donor HLAs; N=36), RTX+/DSA+ (patients with RTX treatment and DSAs against paired donor HLAs; N=31), and RTX−/DSA+ (patients with no RTX treatment and DSAs against the paired donor HLAs; N=30) (Supplemental Data Fig. S1A). This study was approved by the Institutional Review Board (IRB) of Seoul National University Hospital (IRB approval number H-2202-058-1299). Written informed consent was obtained from all subjects.
All 97 recipient serum–donor cell pairs were treated with six different concentrations of pronase (0, 0.5, 1, 2, 3, and 4 mg/mL) and then subjected to FCXM testing. The 0 mg/mL condition was omitted for 11 of the serum–cell pairs owing to insufficient amounts of PBMCs. A total of 571 FCXM experiments were analyzed.
Isolated PBMCs were treated with pronase (type XIV; Sigma Chemical, St. Louis, MO, USA) before incubation with patient serum samples for flow cytometric analysis. Briefly, 0.9×106 cells were incubated in 1 mL of Iscove’s modified Dulbecco’s medium containing pronase (0, 0.5, 1, 2, 3, or 4 mg/mL) for 20 min at 37°C. After the cells were washed twice with wash buffer (phosphate-buffered saline [PBS] containing 0.2% bovine serum albumin), 3×105 cells (30 μL) were incubated with 30 μL of patient serum, negative-control serum (Inert AB Serum, Lorne Laboratories, Twyford, UK), or positive-control serum (rabbit anti-human lymphocyte serum, Cedarlane, Burlington, Canada) for 30 min at 20–25°C. After being washed thrice with wash buffer, the cells were incubated with 1 µL of CD3-PerCP (SK7; BD Biosciences, San Jose, CA, USA), 1.5 µL of CD19-PE (4G7; BD Biosciences), and 0.5 µL of goat F(ab’)2 anti-human IgG-FITC (Jackson ImmunoResearch Laboratories, West Grove, PA, USA) in 100 µL PBS for 20 min at 20–25°C. After three additional washes with wash buffer, the cells were resuspended in 100 µL PBS and analyzed using a FACSCanto II instrument and FACSDiva software (BD Biosciences). The median channel shift (MCS) value of the negative control was subtracted from the MCS value of each sample obtained via flow cytometry. The positive-cutoff MCS values for B-cell and T-cell FCXM testing were 120 and 90, respectively.
The positivity rates for the FCXM testing were compared using the chi-squared test; however, Fisher’s exact test was employed when more than 20% of cells had an expected frequency of less than 5. The MCS values for each pronase-concentration group were compared using the Kruskal–Wallis test, followed by Dunn’s multiple-comparison test if P<0.05. The 86 recipient–donor pairs with available data for all six pronase concentrations (FCXM experiments) were compared pairwise using Friedman’s test, followed by Dunn’s multiple-comparison test in cases where P<0.05. The MFI values for the DSAs in the RTX+/DSA+ and RTX−/DSA+ groups were compared using the Mann–Whitney U-test. P<0.05 was considered to reflect a statistically significant difference. Statistical analyses and graphical illustrations were generated using GraphPad Prism version 8 (GraphPad Software, Boston, MA, USA).
The DSAs in the RTX+/DSA+ group were against HLA-A (N=4), HLA-B (N=12), HLA-DR (N=10), and HLA-DQ (N=5). The median MFI values of the DSAs at each locus did not differ between the RTX−/DSA+ and RTX+/DSA+ groups (Supplemental Data Fig. S1B). The RTX dose, time from RTX administration to blood collection, and number of plasma exchanges during that time did not differ significantly between the RTX+/DSA– and RTX+/DSA+ groups (Supplemental Data Fig. S1B).
We first analyzed the effect of treating donor lymphocytes with pronase on T- and B-cell gating in flow cytometry histograms. The MFI values of CD3+ and CD19+ cells in 34 donor lymphocyte samples incubated with negative-control serum were significantly higher in cells treated with 0.5 mg/mL pronase than in nontreated cells (P<0.0001 for both; Supplemental Data Fig. S2). The mean CD3 MFI values of CD3+ cells did not differ significantly when treated with pronase concentrations above 0.5 mg/mL. In contrast, the mean CD19 MFI values of CD19+ cells were significantly lower with pronase concentrations of 1 mg/mL (1,433±281), 2 mg/mL (885±180), 3 mg/mL (568±132), and 4 mg/mL (374±83) than with a pronase concentration of 0.5 mg/mL (1,815±378) (P<0.0001 for all). At pronase concentrations of 1, 2, 3, and 4 mg/mL, the mean MFI values for CD19 expression were 21%, 51%, 69%, and 79% lower, respectively, than those at a pronase concentration of 0.5 mg/mL. The low MFI values of CD19+ cells treated with 4 mg pronase/mL (which were 79% lower than those observed with 0.5 mg pronase/mL) complicated their distinction from CD19– cells (Supplemental Data Fig. 2A and 2C).
In the RTX+/DSA– group, the false-positive rates of B-cell FCXM at pronase concentrations of 0, 0.5, and 1 mg/mL were 82%, 89%, and 81%, respectively (Fig. 1A), which possibly reflected RTX interference. The positive rates in the RTX+/DSA– group decreased significantly from 81% at 1 mg/mL to 28% at 2 mg/mL and to 0% at 3 and 4 mg/mL pronase (P<0.0001 for all). In contrast, the positive rate in the RTX+/DSA+ group showed relatively smaller decreases from 94% at 1 mg/mL pronase to 74% at 2 mg/mL (P=0.081), 71% at 3 mg/mL (P=0.043), and 65% at 4 mg/mL (P=0.011) (Fig. 1B). The MCS values in the RTX+/DSA+ group did not differ significantly at 2 mg/mL (P=0.425) or 3 mg/mL (P=0.216) from that at 1 mg/mL pronase, but they were significantly lower at 4 mg/mL (P=0.035).
We further analyzed the specificities and MFI values of the DSAs of RTX+/DSA+ cases that were positive at 1 mg/mL pronase but became negative at 2 or 3 mg/mL. Seven of eight of these cases had DSAs against MHC class I with weak intensity (MFI<3,000). The remaining DSA was anti-DQB1*05:02 (MFI: 5,226) (Supplemental Data Table S1). For these eight cases, we analyzed matched RTX−/DSA+ cases with similar MFI intensities and the same specificity. Six of these RTX−/DSA+ cases were negative at 1 mg/mL, but two (anti-B*44:03, MFI: 1,953 and anti-B*51:01, MFI: 2,573) were positive at 1 mg/mL, suggesting that MHC degradation by pronase may have occurred at 2 or 3 mg/mL in the RTX+/DSA+ group.
In the RTX−/DSA+ group, only 19% and 43% of the patients were positive at pronase concentrations of 0 and 0.5 mg/mL, respectively. The positive rate was highest (63%) at a pronase concentration of 1 mg/mL and did not change significantly at 2 (57%), 3 (60%), or 4 mg/mL (57%) (Fig. 1C).
Of the 97 recipient serum–donor cell pairs, 86 were tested via FCXM after pretreatment with all six pronase concentrations. Pairwise statistical analysis of these 86 pairs showed the same pattern of results as those found with the unpaired analysis (Fig. 1 and Supplemental Data Fig. S3).
We investigated whether the B-cell FCXM results that became negative at 2 or 3 mg/mL pronase (which were positive at 1 mg/mL) were due to MHC degradation by pronase or to low DSA levels undetectable by FCXM testing. First, we plotted the MFI values of all DSAs in the RTX+/DSA+ and RTX−/DSA+ groups at 1, 2, and 3 mg/mL pronase according to the B-cell FCXM results (positive or negative; Fig. 2A). To remove outlier effects, we excluded five samples with DSA MFIs of >15,000 in the FCXM-positive group. The median MFI values of the FCXM-negative cases were significantly lower than those of FCXM-positive cases. We further analyzed the FCXM-negative cases and subdivided them into groups according to the pronase concentration (1, 2, or 3 mg/mL) and RTX treatment (Fig. 2B). The median DSA MFIs in the RTX+/DSA+ group (which were negative at 2 and 3 mg/mL) were lower than or not significantly different from the median of DSA MFIs in the RTX−/DSA+ group (which were negative at 1 mg/mL), as shown in Fig. 2B. The median and range of the MFI in each group were 2,401 (1,736–2,987), 2,642 (1,736–5,226), and 3,754 (1,972–6,262), respectively. When further analyzing cases with only one DSA (i.e., excluding those with two DSAs), the DSA MFI values in the RTX+/DSA+ group that were negative at 2 and 3 mg/mL were not significantly different from those in the RTX−/DSA+ group that were negative at 1 mg/mL (Supplemental Data Fig. S4).
Next, we analyzed the effect of pronase treatment on the T-cell FCXM results. As T cells do not express CD20, they do not bind RTX. Concordantly, the RTX+/DSA– group was T-cell FCXM-negative at all pronase concentrations (Fig. 3A), although the MCS values at 0.5, 1, and 2 mg/mL pronase were slightly lower than those at 0 mg/mL pronase (P<0.01, P<0.01, and P<0.05, respectively). No significant difference was observed in the median MCS values according to the pronase concentration in the RTX+/DSA+ and RTX−/DSA+ groups (Fig. 3B–3E). We also performed pairwise statistical analysis of the results for 86 recipient–donor pairs with available data for all six pronase concentrations (Supplemental Data Fig. S5). RTX+/DSA+ and RTX−/DSA+ cases containing MHC class I DSA showed a small but statistically significant increase in MCS values at 1 or 2 mg/mL pronase compared with those at 0 mg/mL pronase (P<0.05 and P<0.01, respectively) (Supplemental Data Fig. S5B and S5C).
Regarding positivity rates, during our analysis of 16 and 18 recipient–donor pairs with MHC class I DSAs in the RTX+/DSA+ and RTX−/DSA+ groups, respectively, the positive rates did not differ significantly according to the pronase concentration (Fig. 3B and 3C). However, when analyzing 15 and 12 recipient–donor pairs with MHC class II DSAs in the RTX+/DSA+ and RTX−/DSA+ groups, respectively, nine crossmatches from four recipient–donor pairs showed positive results (three crossmatches at 0.5 mg/mL pronase, three at 1 mg/mL, two at 2 mg/mL, and one at 3 mg/mL), with the possibility of false-positive results given the rare expression of MHC class II molecules on T cells (Fig. 3D and 3E). Out of 315 crossmatches (180 in the RTX+/DSA– group and 135 in the MHC class II DSA-containing groups) performed with T cells treated with pronase at concentrations of ≥0.5 mg/mL, the false-positive rate was 2.9% (9 of 315 crossmatches; Fig. 3D and 3E).
Using pronase for B-cell FCXM testing reduced nonspecific reactions (possibly by degrading Fc gamma receptors) and helped reduce RTX interference by degrading CD20 [7]. However, concerns have been raised that employing higher pronase concentrations in B-cell FCXM testing may lead to false-negative results because pronase not only reduces the levels of the Fc gamma receptor, CD20, but also MHC class I and II molecules [10]. Increasing the pronase concentration to 3.0 mg/mL with samples from RTX-naive, DSA-positive patients did not change the median MCS value and only slightly decreased the positive rate for B-cell FCXM. This finding probably reflects the smaller reductive effect of 2–3 mg/mL pronase on MHC molecules than on the Fc gamma receptor [10].
We investigated the effects of various pronase concentrations on eliminating RTX interference and the sensitivity of the FCXM assay. We included weak-to-moderate DSA levels against HLA-A, HLA-B, HLA-DR, and HLA-DQ and tested a wide range of pronase concentrations (0–4 mg/mL). At 4 mg/mL pronase, the CD19 intensity was markedly reduced, rendering proper gating difficult in terms of distinguishing cells that were only positive for CD19. We believe that a pronase concentration of 4 mg/mL is unlikely to be applicable for routine B-cell FCXM testing.
For B-cell FCXM testing, the MCSs in the RTX+/DSA– group decreased significantly with increasing pronase concentrations. The number of false-positive results due to RTX gradually decreased with increasing pronase concentrations and disappeared completely at 3 mg/mL pronase. In contrast, the MCSs and positive rates in the RTX+/DSA+ and RTX−/DSA+ groups were not significantly lower at 2 or 3 mg/mL pronase than at 1 mg/mL pronase, suggesting that pronase concentrations up to 3 mg/mL do not considerably affect the sensitivity of DSA detection. Nevertheless, 2 or 3 mg/mL pronase still carried a risk for false-negative results due to HLA degradation.
We further analyzed eight RTX+/DSA+ cases that were positive at 1 mg/mL pronase but became negative at 2 or 3 mg/mL pronase. Seven of the eight cases had weak DSAs (MFI<3,000), and the remaining case had anti-DQB1*05:02 (MFI: 5,226). MFI values for anti-DQ antibodies obtained with a single-antigen bead assay are known to be exaggerated, as HLA-DQ expression is much lower than HLA-DR expression in cells; however, its antigen density on beads is similar to that of HLA-DR [15]. In addition, six of the eight RTX−/DSA+ cases matching these eight RTX+/DSA+ cases regarding DSA specificity and MFI were negative at 1 mg/mL pronase. However, two cases with anti-B*51:01 (MFI: 2,573) and anti-B*44:03 (MFI: 1,953) tested positive at 1 mg/mL pronase. The negative results at 2 or 3 mg/mL pronase likely reflected both weak DSA levels and HLA degradation by pronase.
In contrast to B-cell FCXM testing, where false-negative results are a major problem with pronase treatment, false-positive results attributed to the exposure of cryptic epitopes have been reported with T-cell FCXM testing [12, 13]. We observed nine false positives via T-cell FCXM in the MHC class II DSA+ groups. Positive results in the RTX+/DSA+ (MHC class II) and RTX−/DSA+ (MHC class II) groups were likely false positives, as T cells rarely express MHC class II molecules. The false-positive rate for pronase-treated T cells observed via FCXM testing was 2.9%, which was slightly lower than previously reported [12, 13]. In contrast to previous findings showing a significant decrease in the MFI for MHC class I molecules after 2 or 3 mg/mL pronase treatment [10], we observed that the MCS in the DSA-positive group did not significantly decrease with 2 or 3 mg/mL pronase, possibly owing to a concurrent decrease in the MFI of the negative control. These results should be validated with a larger number of patients.
A limitation of this study is that the experiments were performed by a single technician in a single laboratory. The reproducibility of these results in other laboratories has not been validated. Another limitation is that DSAs for HLA-C and HLA-DP were not included in this study. Since these antigens are expressed at low levels, which renders them more susceptible to pronase degradation and can lead to false-negative FCXM results [16], the possibility of false-negative results in the presence of DSAs for these antigens should be validated further.
Nevertheless, our findings demonstrated the potential efficacy of pretreatment with higher pronase concentrations (2 or 3 mg/mL) as a means for eliminating RTX interference in B-cell FCXM testing, particularly for patients with moderate-to-strong DSA. Current B-cell FCXM methods do not provide reliable results for patients receiving RTX, nor do they provide additional information for determining the timing of transplantation [11]. Using higher pronase concentrations (2 or 3 mg/mL) may be another option for detecting moderate-to-strong DSA and increasing the specificity of B-cell FCXM testing, which may aid in clinical decision-making for patients undergoing kidney transplantations. To begin employing higher pronase concentrations in clinical laboratories, each laboratory will need to find an optimal pronase concentration that enables adequate CD19 gating, minimizes RTX interference, and does not compromise the sensitivity of low-level DSA detection, which will require the training of technicians to ensure reproducible results.
Notes
AUTHOR CONTRIBUTIONS
Lee H and Song EY contributed to the conception and design of the study. Oh I and Choi YJ contributed to data acquisition. Oh I, Kim TS, and Nam M interpreted and analyzed the data. Kim TS and Song EY wrote the manuscript. Song EY supervised the study. All authors read and approved the final manuscript.
Appendix
SUPPLEMENTARY MATERIALS
Supplementary materials can be found via https://doi.org/10.3343/alm.2024.0132
References
1. Abbes S, Metjian A, Gray A, Martinu T, Snyder L, Chen DF, et al. 2017; Human leukocyte antigen sensitization in solid organ transplantation: a primer on terminology, testing, and clinical significance for the apheresis practitioner. Ther Apher Dial. 21:441–50. DOI: 10.1111/1744-9987.12570. PMID: 28880430. PMCID: PMC6890204.
2. Downing J. 2012; The lymphocyte crossmatch by flow cytometry for kidney transplantation. Methods Mol Biol. 882:379–90. DOI: 10.1007/978-1-61779-842-9_22. PMID: 22665246.
3. Nam M, Song EY. 2023; Impact of low-level donor-specific antibody determined with a positive luminex and negative flow cytometric crossmatch on kidney transplantation outcomes. Ann Lab Med. 43:325–7. DOI: 10.3343/alm.2023.43.4.325. PMID: 36843400. PMCID: PMC9989535.
4. Guillaume N. 2018; Improved flow cytometry crossmatching in kidney transplantation. HLA. 92:375–83. DOI: 10.1111/tan.13403. PMID: 30270577.
5. Lázár-Molnár E, Delgado JC. 2019; Implications of monoclonal antibody therapeutics use for clinical laboratory testing. Clin Chem. 65:393–405. DOI: 10.1373/clinchem.2016.266973. PMID: 30377156.
6. Kueht ML, Dongur LP, Mujtaba MA, Cusick MF. 2023; Antibody therapeutics as interfering agents in flow cytometry crossmatch for organ transplantation. J Pers Med. 13:1005. DOI: 10.3390/jpm13061005. PMID: 37373995. PMCID: PMC10301800.
7. Bearden CM, Agarwal A, Book BK, Sidner RA, Gebel HM, Bray RA, et al. 2004; Pronase treatment facilitates alloantibody flow cytometric and cytotoxic crossmatching in the presence of rituximab. Hum Immunol. 65:803–9. DOI: 10.1016/j.humimm.2004.06.001. PMID: 15336781.
8. Lobo PI, Spencer CE, Stevenson WC, McCullough C, Pruett TL. 1995; The use of pronase-digested human leukocytes to improve specificity of the flow cytometric crossmatch. Transpl Int. 8:472–80. DOI: 10.1111/j.1432-2277.1995.tb01558.x. PMID: 8579739.
9. Vaidya S, Cooper TY, Avandsalehi J, Barnes T, Brooks K, Hymel P, et al. 2001; Improved flow cytometric detection of HLA alloantibodies using pronase: potential implications in renal transplantation. Transplantation. 71:422–8. DOI: 10.1097/00007890-200102150-00015. PMID: 11233905.
10. Apithy MJ, Desoutter J, Gicquel A, Guiheneuf E, Westeel PF, Lesage A, et al. 2017; Pronase treatment improves flow cytometry crossmatching results. HLA. 90:157–64. DOI: 10.1111/tan.13073. PMID: 28660746.
11. Kang H, Yoo J, Lee SY, Oh EJ. 2021; Causes of positive pretransplant crossmatches in the absence of donor-specific anti-human leukocyte antigen antibodies: a single-center experience. Ann Lab Med. 41:429–35. DOI: 10.3343/alm.2021.41.4.429. PMID: 33536364. PMCID: PMC7884190.
12. Park H, Lim YM, Han BY, Hyun J, Song EY, Park MH. 2012; Frequent false-positive reactions in pronase-treated T-cell flow cytometric cross-match tests. Transplant Proc. 44:87–90. DOI: 10.1016/j.transproceed.2011.12.048. PMID: 22310587.
13. Hetrick SJ, Schillinger KP, Zachary AA, Jackson AM. 2011; Impact of pronase on flow cytometric crossmatch outcome. Hum Immunol. 72:330–6. DOI: 10.1016/j.humimm.2011.01.005. PMID: 21262310.
14. Szewczyk K, Barrios K, Magas D, Sieg K, Labuda B, Jendrisak MD, et al. 2016; Flow cytometry crossmatch reactivity with pronase-treated T cells induced by non-HLA autoantibodies in human immunodeficiency virus-infected patients. Hum Immunol. 77:449–55. DOI: 10.1016/j.humimm.2016.04.014. PMID: 27094930.
15. Tait BD, Süsal C, Gebel HM, Nickerson PW, Zachary AA, Claas FH, et al. 2013; Consensus guidelines on the testing and clinical management issues associated with HLA and non-HLA antibodies in transplantation. Transplantation. 95:19–47. DOI: 10.1097/TP.0b013e31827a19cc. PMID: 23238534.
16. Silva C, Aires P, Santo P, Xavier P. 2023; Low expression loci and the use of pronase in flow cytometry crossmatch. Transplant Proc. 55:1383–9. DOI: 10.1016/j.transproceed.2023.03.075. PMID: 37173263.
Fig. 1
B-cell flow cytometry crossmatch testing results. Sera from three groups, RTX+/DSA– (A), RTX+/DSA+ (B), and RTX−/DSA+ (C), were crossmatched with lymphocytes treated with the indicated pronase concentrations. The gray circles represent the results for each sample, and the black lines represent the median and interquartile range. The dotted line indicates the median channel shift (MCS) cutoff (120) for positive results. The median MCSs of the 2, 3, and 4 mg/mL pronase groups were compared with that of the 1 mg/mL pronase group using the Kruskal–Wallis test, followed by Dunn’s multiple-comparison test. The table below each graph shows the total number of samples tested (Total), the number of positive samples (Pos.), and the percentage of positive results (Pos. %). *P<0.05; ****P<0.0001.
Abbreviations: RTX, rituximab; DSA, donor HLA-specific antibody; ns, not significant.
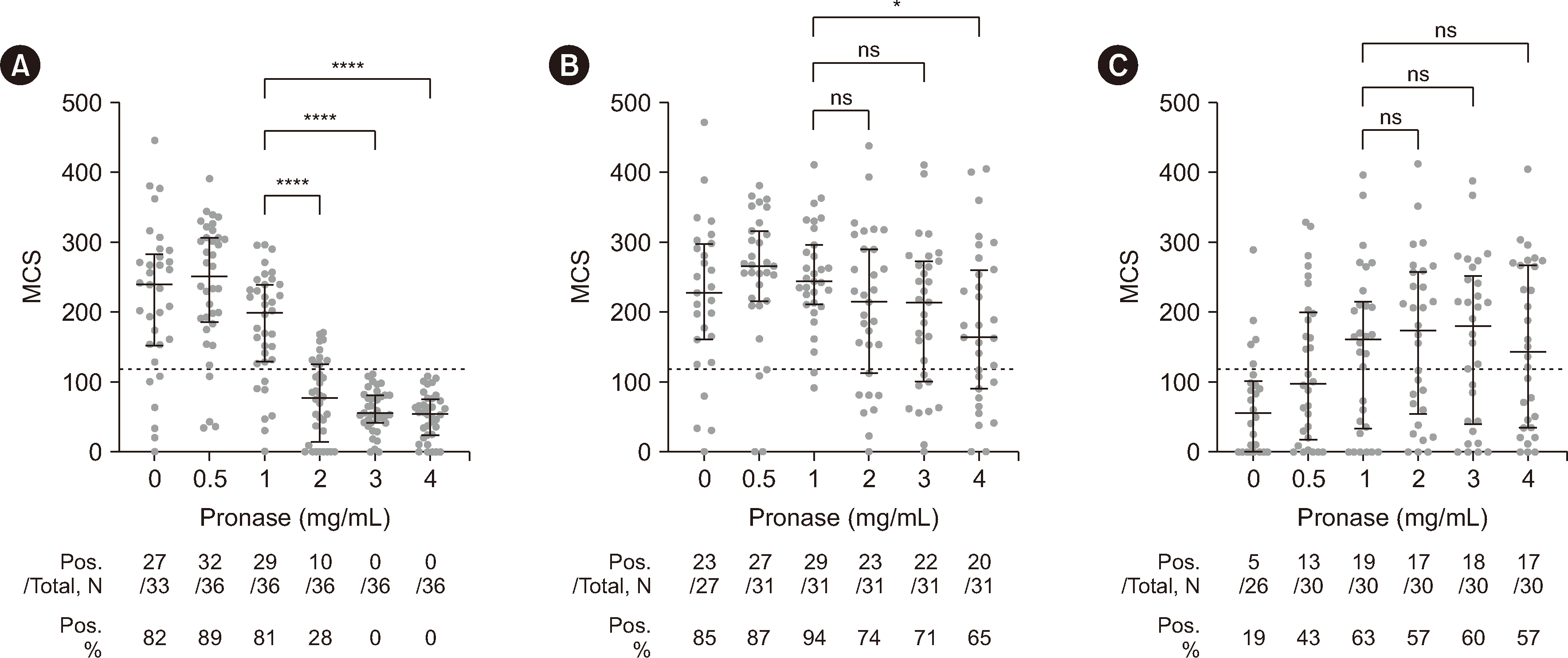
Fig. 2
Comparison of the mean fluorescence intensities (MFIs) of donor HLA-specific antibodies (DSAs) present in RTX+/DSA+ and RTX−/DSA+ sera. (A) The MFI values of all DSAs in the RTX+/DSA+ and RTX−/DSA+ groups at 1, 2, and 3 mg/mL pronase were plotted according to the B-cell flow cytometry crossmatch (FCXM) results (positive or negative). For cases with two DSAs, the MFI sum of both DSAs was used. (B) The FCXM-negative cases represented in (A) were divided into the RTX+/DSA+ and RTX−/DSA+ groups and subdivided according to 1, 2, and 3 mg/mL pronase treatment (P1, P2, and P3, respectively). The horizontal lines and error bars represent the median and interquartile range of each column, respectively.
Abbreviations: RTX, rituximab; XM, crossmatches; ns, not significant.
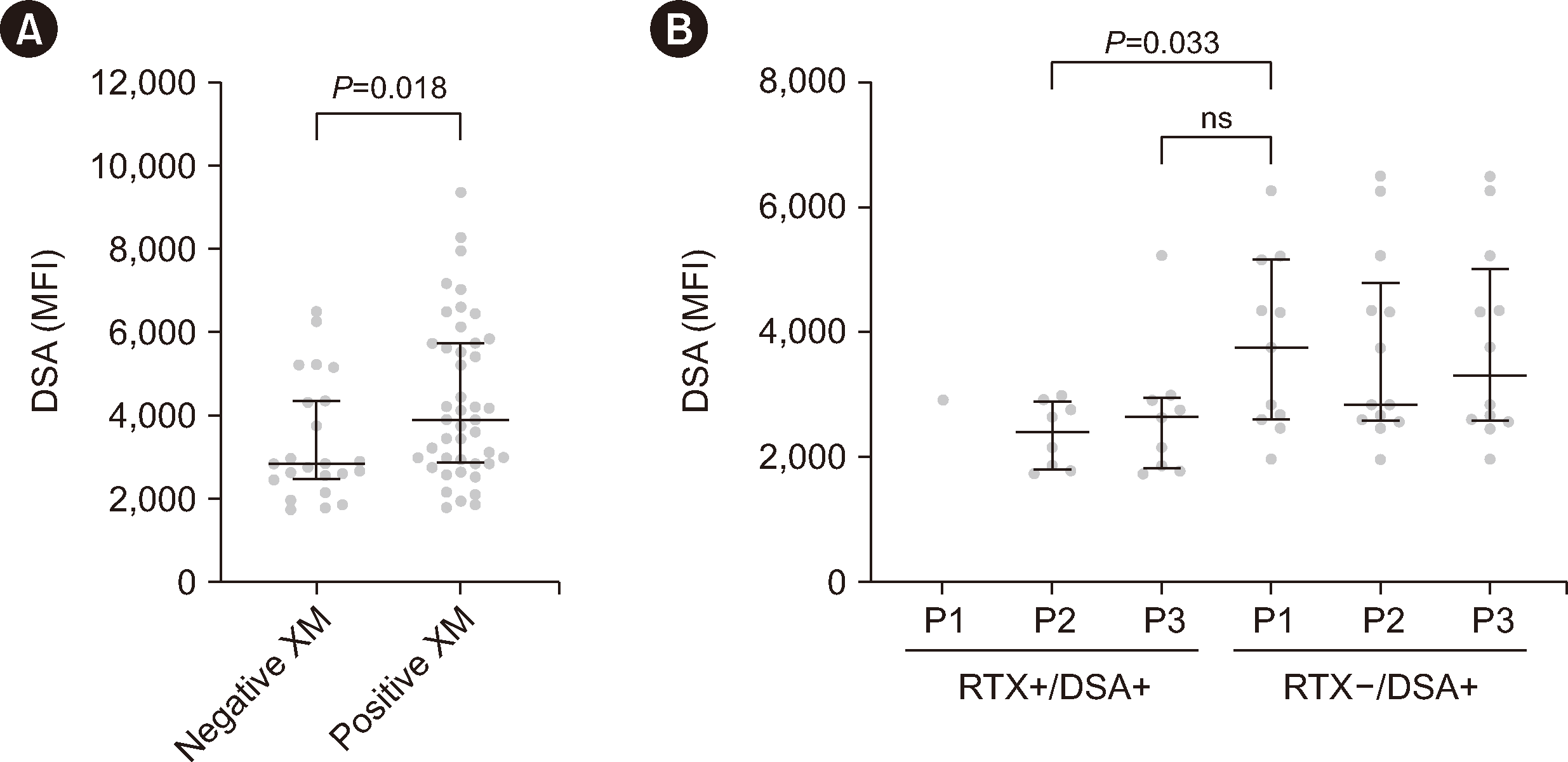
Fig. 3
T-cell flow cytometry crossmatch testing results. Sera from the RTX+/DSA– (A), RTX+/DSA+ (B and D), and RTX−/DSA+ (C and E) groups were crossmatched with lymphocytes treated with the indicated pronase concentrations. The crossmatching results for RTX+/DSA+ and RTX−/DSA+ sera were plotted in separate graphs based on the MHC class of the DSAs present in each serum sample because MHC class II molecules are usually not expressed on naive T cells (B and C for MHC class I; D and E for MHC class II). The dotted lines indicate the median channel shift (MCS) cutoff (90) for positive results. The median MCSs of each column were compared with that of the control column (0 mg/mL pronase) using the Kruskal–Wallis test, followed by Dunn’s multiple-comparison test. The table below each graph shows the total number of samples tested (Total), the number of positive samples (Pos.), and the percentage of positive results (Pos. %). *P<0.05; **P<0.01.
Abbreviations: RTX, rituximab; DSA, donor HLA-specific antibodies; ns, not significant.
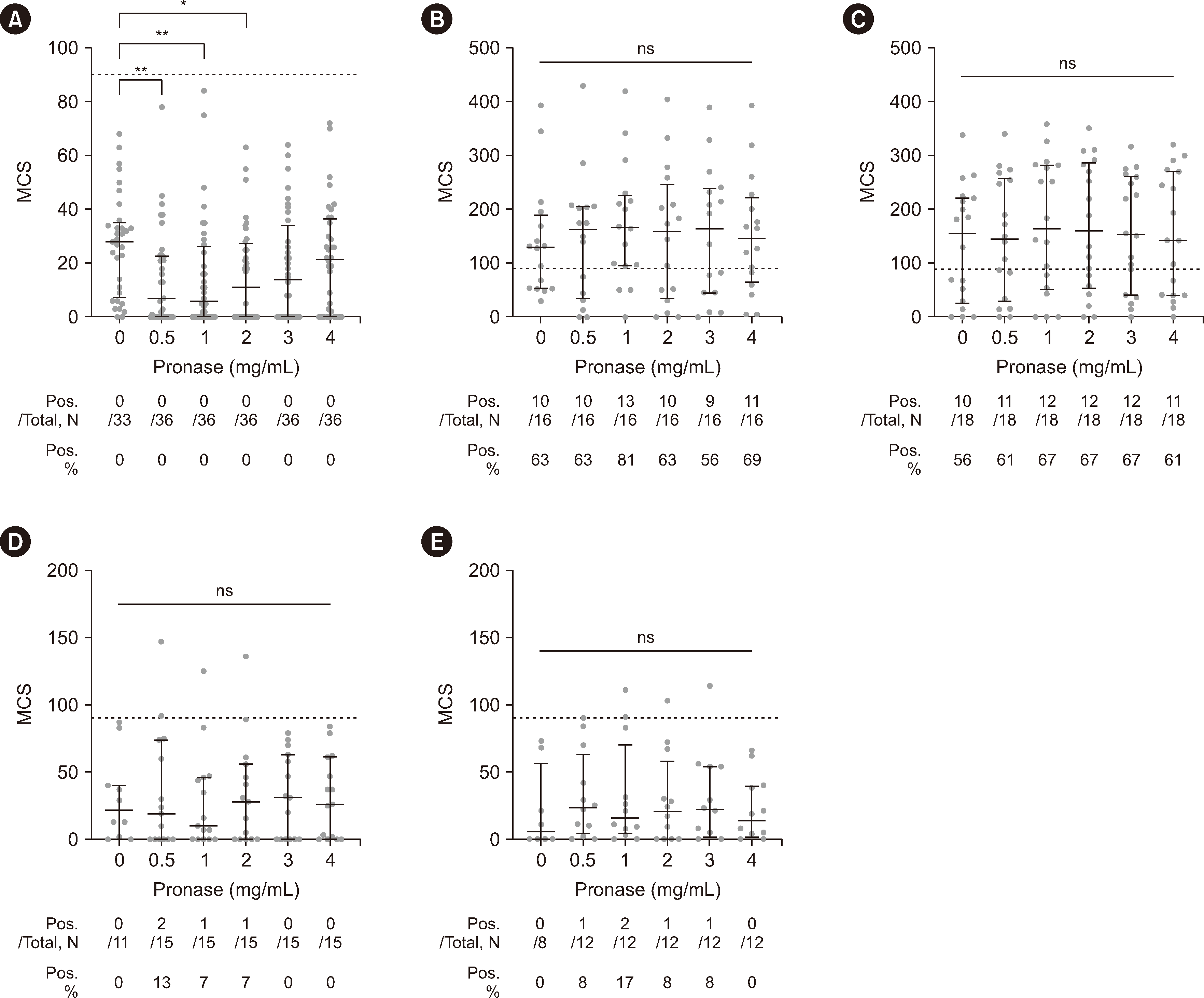