Abstract
Objective
We sought to investigate how priming the tube between air versus air mixed with saline ex vivo influenced suction force. We examined how priming the tube influenced peak suction force and time to achieve peak suction force between both modalities.
Methods
Using a Dwyer Instruments (Dwyer Instruments Inc., Michigan City, IN, USA), INC Digitial Pressure Gauge, we were able to connect a .072 inch aspiration catheter to a rotating hemostatic valve and to aspiration tubing. We recorded suction force measured in negative inches of Mercury (inHg) over 10 iterations between having the aspiration tube primed with air alone versus air mixed with saline. A test was used to compare results between both modalities.
Results
Priming the tube with air alone compared to air mixed with saline was found to have an increased average max suction force (-28.60 versus -28.20 in HG, p<0.01). We also identified a logarithmic curve of suction force across time in which time to maximal suction force was more prompt with air compared with air mixed with saline (13.8 seconds versus 21.60 seconds, p<0.01).
Conclusions
Priming the tube with air compared to air mixed with saline suggests that not only is increased maximal suction force achieved, but also the time required to achieve maximal suction force is less. This data suggests against priming the aspiration tubing with saline and suggests that the first pass aspiration primed with air may have the greatest suction force.
Thrombectomy is the standard of care for reperfusion of large vessel occlusion as established by numerous randomized trials [4,5,8,12]. Intra-arterial treatment leads to reperfusion with a success rate between 70-90% [4-6,15]. Decreases in femoral artery puncture to revascularization time are correlated with positive patient outcomes and explain the clinical effectiveness seen in newer generation intra-arterial therapy devices. First pass reperfusion in addition to prompt intervention strongly improves clinical outcomes [1,5,10]. Suction force can be one of many factors in determining thrombectomy rate, efficacy, and decrease the likelihood of distal emboli formation [2,9,15].
When performing aspiration thrombectomy, the aspiration catheter regardless of size and diameter must be connected to aspiration tubing which in turn connects to a suction cannister and engine. During connection and testing of the equipment, the catheter is inherently filled with air, but could instead be primed with saline prior to insertion into the femoral artery access site. When performing thrombectomy, there is controversy as to whether a priming medium of saline or air impacts suction force which in turn can impact first pass revascularization.
To date, the physical properties of saline or air for the purposes of producing maximum static suction force and associated first derivative inside an aspiration catheter have not been investigated. Our group conducted the first investigation comparing the physical properties of saline and air as catheter priming mediums and the impact this has on static suction force. Higher suction forces would theoretically lead to higher probability of first pass embolectomy and subsequent reperfusion, with associated improved clinical outcomes.
The Penumbra ENGINE suction thrombectomy device, and RED 72 aspiration catheter (Penumbra, Alameda, CA, USA) was used for this study. Our set up (Fig. 1) consisted of a Dwyer instrument (Dwyer Instruments Inc., Michigan City, IN, USA) which measures pressure in negative in/HG was connected to a rotating hemostatic value (RHV). The free port of the RHV was sealed with a flow switch in the off position. The RHV was additionally connected to the RED 72 aspiration catheter. The RED 72 aspiration catheter was then connected to the Penumbra aspiration tubing. The tubing was connected in series to the Penumbra cannister and ENGING suction thrombectomy device.
We performed ten consecutive trials using air as the fluid within the aspiration tubing and recorded serial pressure measurements. Suction values at 1, 5, and 10 seconds, maximum suction value, and time to maximum suction value (Tmax) were compared between trials and priming mediums. Maximum suction value was defined as the average of the last ten pressure values at the end of the trial, when pressure was nearly constant. Tmax was defined as the amount of time until the suction value fell within the error of maximum suction. Following our ten trials using air as the fluid within the aspiration tubing, we then injected saline into the aspiration tubing and performed additional trials. Following each trial with saline within the aspiration tubing, we re-introduced saline to ensure the aspiration tubing was appropriately primed. For all trials, we confirmed pressure readings were appropriately zeroed prior to testing suction force.
The suction forces with respect to time were plotted and a best fit curve was calculated. T-tests were used to determine the statistical significance of differences between priming medium pressure values. F-tests were used to determine which t-test was appropriate for the given variable measured.
The best fit curve for the suction data was a logarithmic function (Fig. 2). There were statistically significant differences between air and saline as priming mediums for maximum suction (p<10-15), Tmax (p<10-5), and pressures recorded at 5 (p<10-12) and 10 (p<10-7) seconds. Suction values at 1 second had large standard deviations compared to the other time-specific variables and were not found to be statistically significant (p=0.06). A Grubbs test was performed on the least negative values in both the air and saline data sets for the 1 second time value and determined that both sets contained an outlier. Discarding these values, there was a statistically significant difference between the average air and saline suction forces at 1 second (p<0.0005). Overall, suction values for air were more negative than saline, with greater suction force achieved at all time points after one second. Averages with corresponding standard deviations can be found in Table 1.
Our data demonstrates increased suction force with priming the aspiration tube with air over saline at specific time points. The time required for an air primed catheter to achieve maximal suction force was nearly half the time of a saline primed catheter, resulting in a more negative first derivative of pressure with respect to time. This data would suggest that when performing aspiration thrombectomy, having the aspiration tube primed with air will allow for increased suction force and a shorter duration of time to achieve greater suction forces. Suction dynamic differences between gas and liquid phase mediums could potentially be related to the compressibility of the medium [13].
This data also suggests that when aspiration thrombectomy in vivo clinical scenarios is performed with the tubing primed air and a second pass attempt is required – the tubing which may be filled with a mixture of air, blood, and saline may have both reduced suction force and require greater durations of time to achieve that reduced force. This data may suggest that the physical properties of air assist in the first pass effect which improves reperfusion in some cases [1,5,10].
The scope of this study was limited to 072 catheter and air and saline priming mediums. Further work investigating the physical properties of static vacuum force aspiration would include smaller catheters to see if diameter further increases or mitigates the physical effect between air and saline due to resistance forces. The geometrical subtleties of vessels encountered in vivo are also more complex than what is simulated in this study, and the differences in suction dynamics due to vessel architecture are not known. Additional work should investigate suction force dynamics of blood due to both viscosity differences and coagulating properties of blood and heparin within the fluid medium, each of which may impact suction force. Investigation regarding the risk of air micro embolism when using a catheter primed with air as opposed to saline would also be warranted prior to in vivo application. However, given the directionality of aspiration withdrawing fluid away from the body, micro emboli should not advance into the patient. The results of this data suggest that further clinical experience is required to examine whether the difference in suction force and duration to achieve maximal suction will in turn yield the benefit of a greater incidence of immediate total reperfusion.
Our data appears to contradict a prior study which suggests that saline is the optimal priming medium [13]. Simon, et al demonstrated that cyclic aspiration of artificial thrombi was more effective with water as the priming medium versus air. The frequencies investigated included 1, 2 and 6.3 Hz. Additionally, the catheter diameter used in their model was a smaller diameter than our study (1.37 mm vs 1.8 mm), with fluid resistance forces impacted heavily by radius as demonstrated by Poiseuille’s Law and thus demonstrated effects on clinical outcomes [7,11]. Potential explanations for the observed differences are the fact that our study simulates complete adherence of the catheter tip to the thrombus and thus flow is near zero, nullifying fluid resistance as a factor once the vacuum is established in the catheter [3]. This demonstrates static aspiration as opposed to cyclic aspiration, with time scales much greater than those afforded by the cycling frequencies as the vacuum increases to its maximum value. Additionally, tubing diameter effect on fluid velocities while the vacuum is increasing, and limitations of the suction engines used may contribute to the observed differences [14]. More accurate measurements of pressure and multiple catheter diameters should be used in order to elucidate the details of these discrepancies.
The physical properties of aspiration would benefit from further investigation in both in vivo and in vitro studies. Understanding ideal properties of a medium can in turn optimize suction force to improve first pass aspiration. We hope this physical examination of the properties of suction force dynamics allows us to further question fundamental aspects of routine procedures and critically reflect on the standard assumption that all fluids possess identical suction force dynamics.
REFERENCES
1. Abbasi M, Liu Y, Fitzgerald S, Mereuta OM, Arturo Larco JL, Rizvi A, et al. Systematic review and meta-analysis of current rates of first pass effect by thrombectomy technique and associations with clinical outcomes. J Neurointerv Surg. 2021; Mar. 13(3):212–6.


2. Boisseau W, Escalard S, Fahed R, Lapergue B, Smajda S, Maier B, et al. Direct aspiration stroke thrombectomy: A comprehensive review. J Neurointerv Surg. 2020; Nov. 12(11):1099–106.
3. Froehler MT. Comparison of vacuum pressures and forces generated by different catheters and pumps for aspiration thrombectomy in acute ischemic stroke. Interv Neurol. 2017; Oct. 6(3-4):199–206.
4. Goyal N, Tsivgoulis G, Frei D, Turk A, Baxter B, Froehler MT, et al. Comparative safety and efficacy of modified TICI 2b and TICI 3 reperfusion in acute ischemic strokes treated with mechanical thrombectomy. Neurosurgery. 2019; Mar. 84(3):680–6.
5. Jang KM, Choi HH, Nam TK, Byun JS. Clinical outcomes of first-pass effect after mechanical thrombectomy for acute ischemic stroke: A systematic review and meta-analysis. Clin Neurol Neurosurg. 2021; Dec. 211:107030.


6. Jeon JP, Kim SE, Kim CH. Primary suction thrombectomy for acute ischemic stroke: A meta-analysis of the current literature. Clin Neurol Neurosurg. 2017; Dec. 163:46–52.


7. Kim S, Lee JY. Comparison of vacuum pressures and suction forces generated by different pump systems for aspiration thrombectomy. Front Neurol. 2022; Oct. 13:978584.
8. Lapergue B, Blanc R, Gory B, Labreuche J, Duhamel A, Marnat G, et al. Effect of endovascular contact aspiration vs stent retriever on revascularization in patients with acute ischemic stroke and large vessel occlusion: The ASTER randomized clinical trial. JAMA. 2017; Aug. 318(5):443–52.
9. Long TD, Kallmes DF, Hanel R, Shigematsu T, Halaszyn AM, Wolter J, et al. Novel aspiration catheter design for acute stroke thrombectomy. J Neurointerv Surg. 2019; Feb. 11(2):190–5.


10. Nikoubashman O, Dekeyzer S, Riabikin A, Keulers A, Reich A, Mpotsaris A, et al. True first-pass effect. Stroke. 2019; Aug. 50(8):2140–6.
11. Nikoubashman O, Nikoubashman A, Büsen M, Wiesmann M. Necessary catheter diameters for mechanical thrombectomy with ADAPT. AJNR Am J Neuroradiol. 2017; Dec. 38(12):2277–81.
12. Rennert RC, Wali AR, Steinberg JA, Santiago-Dieppa DR, Olson SE, Pannell JS, et al. Epidemiology, natural history, and clinical presentation of large vessel ischemic stroke. Neurosurgery. 2019; Jul. 85(suppl 1):S4–8.


13. Simon S, Grey CP, Massenzo T, Simpson DG, Longest PW. Exploring the efficacy of cyclic vs static aspiration in a cerebral thrombectomy model: An initial proof of concept study. J Neurointerv Surg. 2014; Nov. 6(9):677–83.
14. Yaeger K, Iserson A, Singh P, Wolf J, Vidal E, Oxley T, et al. A technical comparison of thrombectomy vacuum aspiration systems. J Neurointerv Surg. 2020; Jan. 12(1):72–6.
15. Yoo AJ, Andersson T. Thrombectomy in acute ischemic stroke: Challenges to procedural success. J Stroke. 2017; May. 19(2):121–30.
Fig. 2.
Suction force data at key time points (1, 5, 10 seconds and Tmax) and corresponding best fit curves with equation and Pearson Correlation Coefficients.
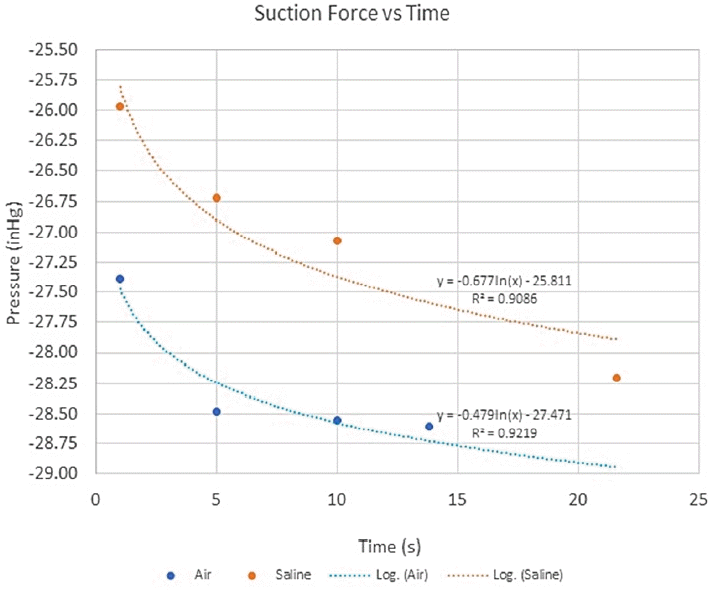
Table 1.
Average suction force values at key time points, average Tmax, and corresponding t-test p-values