Abstract
Purpose
Whether remote ischemic preconditioning (RIPC), repeated bouts of ischemia and reperfusion, prevents arterial stiffening following an acute high-intensity resistance exercise (RE) is unknown. We tested the hypothesis that RIPC would attenuate arterial stiffening following acute high-intensity RE in healthy adults.
Methods
Using a randomized, single-blind, sham-controlled, crossover design, 14 adults (eight males and six females; age, 26±1 years) completed two testing sessions, separated by 1 week. Sessions consisted of either an RIPC intervention (4×5 minutes, 220 mm Hg, bilateral arm occlusion) or a sham condition (4×5 minutes, 20 mm Hg, bilateral arm occlusion) prior to RE (two sets, eight exercises, 80% of one repetition maximum). Carotid-femoral pulse wave velocity (cfPWV) and augmentation index at 75 beats per minute (AIx@75bpm) as indices of arterial stiffness and blood pressures were measured at baseline, immediately after RE, and 30 minutes, 1 hour after RE using an applanation tonometry.
It is known that increased arterial stiffness is associated with increased risks of cardiovascular events and mortality1. Although resistance exercise (RE) training has been recommended by the American Heart Association in the prevention and treatment of cardiovascular diseases2, acute high-intensity RE has been shown to transiently provoke detrimental effects on vascular function3. Evidence suggests that acute high-intensity RE increases arterial stiffness in young healthy adults4,5. The precise mechanisms underlying the arterial stiffening following acute high-intensity RE are not fully understood but may likely be mediated by elevated arterial pressure4, increased sympathetic nerve activity6, oxidative stress7, and muscle damage-induced inflammation with an increased creatine kinase activity7,8.
It is well established that ischemic preconditioning reduces myocardial damage during subsequent prolonged ischemia/reperfusion challenges9. Remote ischemic preconditioning (RIPC), controlled repeated bouts of ischemia and reperfusion in an extremity, has provided a strategy to prevent or attenuate cardiac cell damage caused by ischemia/reperfusion10. Interestingly, the protective effects of RIPC have been demonstrated in the vasculature, including blood pressure (BP) reduction11 and improved endothelial function in healthy individuals12 and in patients with coronary artery disease13. Other studies have shown that RIPC suppresses pro-inflammatory gene transcription of leukocytes14 and reduces creatine kinase activity15. Thus, it is possible that some of these cardioprotective mechanisms of RIPC may contribute to the prevention of the RE-induced detrimental effects on vascular function. Therefore, the purpose of this study was to test the hypothesis that RIPC would attenuate an acute RE-mediated increase in arterial stiffness in healthy adults.
Fourteen healthy male (n=8) and female subjects (n=6) participated in this randomized, single-blind, crossover study. Subjects were excluded if present with any of the following: cardiovascular diseases, metabolic syndrome, or musculoskeletal disorder.
All subjects were informed of the methods of the study and provided written informed consent prior to study participation. The study was approved by the Public Institutional Review Board of Korea Ministry of Health and Welfare and adhered to the Declaration of Helsinki16.
All subjects were instructed to remain fasted for 10 hours and to refrain from physical activity, nutritional supplements, alcohol, and caffeine for 24 hours before testing. Subjects reported twice to our laboratory at the same time of day from 9:00 to 11:00 am, each separated by at least 5–7 days to prevent any residual effect of the previous exercise bout. Subjects were randomized to either one of the two trials: RIPC or sham as a control trial. The experimental design is illustrated in Fig. 1. Following a 30-minute rest in the supine position, baseline measurement was collected for BP and central arterial stiffness, followed by either the RIPC or sham trial. Then, subjects performed two sets of 12 repetitions of RE at 60%–80% of one-repetition maximum (1-RM). Data collected was resumed in the supine position immediately following RE and at 30 minutes and 1 hour after RE. Heart rate (HR) was obtained using a Polar HR monitor (RS800CX, Polar Electro Oy). BP was obtained twice with a 3-minute interval using an automatic BP monitor (WatchBP Home 3MX-1, Microlife Corp), and the BP values of within ±5 mm Hg were recorded. Central arterial stiffness was obtained using radial artery pressure waveform (SphygmoCor, AtCor Medical).
Height and weight were measured using a stadiometer (to the nearest 0.1 cm) and a beam balance platform scale, respectively. Body mass index was calculated as weight (kg) divided by squared height (m).
RIPC consists of repeated bouts of ischemia, followed by reperfusion. RIPC was performed in the supine position using bilateral arterial occlusion of the upper limbs17,18. The automated occlusion cuffs were placed proximally around the upper arms and inflated to 220 mm Hg for 5 minutes to allow for a complete blockage of arterial blood flow to the upper limbs. This ischemic procedure was repeated four times bilaterally, each separated by 5 minutes of reperfusion (4×5 minutes, 220 mm Hg, bilateral occlusion). The sham trial followed the same protocol except the occlusion cuffs were inflated to 20 mm Hg (sham; 4×5 minutes, 20 mm Hg, bilateral occlusion)17,18.
RE consisted of five upper body exercises (chest press, lat pull-down, biceps curl, back extension, and torso rotation) and three lower body exercises (leg press, leg extension, and leg curl). The exercise intensity was 60% of 1-RM for the upper body and 80% for the lower body. These RE intensities from previous studies have been commonly used to successfully make acute vascular changes. Subjects completed two sets of 12 repetitions for each exercise. The 1-RM was determined according to a previously described protocol19 and was recommended by the National Strength and Conditioning Association. Subjects performed a warm-up with the weight up to 15 repetitions. After 1 minute of rest, subjects performed 3 to 5 repetitions with 75% of the predicted maximum weight. After another 2 minutes of rest, they repeated it with increasing weight by 5 to 10 kg and 10 to 20 kg from 75% of the predicted maximum weight for the upper and lower body, respectively. If they failed to repeat three to five times, they took 2 to 4 minutes of rest. Following this rest period, they repeated with a weight that was reduced by 5 kg for the upper body and 10 kg for the lower body from the previous weight. The 1-RM testing was measured by a trained specialist.
All measurements were conducted following guidelines of the Clinical Application of Arterial Stiffness, Task Force III20. The augmentation index (AIx) of the aorta was measured to estimate central arterial stiffness. Following a 30-minute rest in the supine position, radial artery pressure waveforms were obtained from a 10-second epoch using applanation tonometry and a high-fidelity strain gauge transducer (Millar Instruments). Using a generalized validated transfer function, a central aortic pressure waveform was reconstructed from the aforementioned radial artery pressure waveform. The use of a transfer function to synthesize a central pressure waveform from a peripheral radial waveform has been validated at rest and during exercise21. AIx was calculated as the ratio of amplitude of the pressure wave above its systolic shoulder (i.e. the difference between the early and late systolic peaks of the arterial waveform) to the total pulse pressure. The result was expressed as a percentage and used as an index of systemic arterial stiffness. Because AIx is influenced by changes in HR, AIx values were normalized to an HR of 75 beats per minute (AIx@75 bpm)20. Only high-quality recordings, defined as an in-device quality index ≥80%, were included in the analysis. Also derived from the aortic pressure waveform were central systolic BP (CSBP) and central diastolic BP (CDBP).
Aortic stiffness was measured using carotid to femoral pulse wave velocity (cfPWV)20. Distances from the carotid artery sampling site to the femoral artery and the carotid artery to the suprasternal notch were measured as straight lines with a tape measure. The distance from the carotid artery to the sternal notch was then subtracted from the carotid-femoral segment length to account for differences in the direction of pulse wave propagation. Pulse waves were obtained using applanation tonometry. Pulse wave velocity was determined from the foot-to-foot pressure wave velocity, which was determined as D/(Δt) (m/sec), where Δt is the time difference between carotid-femoral pressure waves, and D is the distance between the two arteries. The peak of an in-phase R wave, as attained from sequential electrocardiogram monitoring (CM5 configuration) was used as a timing marker. All measurements were made in duplicate, and the mean value was used for subsequent analysis. This technique has been shown to be highly reproducible5.
All data are reported as mean±standard error. A two trials×four time points analysis of variance with repeated measures was used to determine differences in all dependent variables over time. Whenever significant interactions were detected, post-hoc comparisons were conducted. All data analyses were carried out using IBM SPSS version 21.0 (IBM Corp.). A priori significance was set at an alpha level ≤0.05.
Subject characteristics are shown in Table 1. No baseline differences were observed for any dependent variables in both RIPC and sham trials (Table 2 and Fig. 2). HR and brachial and central BP responses to RE are presented in Table 2. Compared to baseline, HR, brachial systolic BP, brachial mean arterial pressure (BMAP), brachial pulse pressure, CSBP, central mean arterial pressure, and central pulse pressure increased immediately after RE and returned to baseline values thereafter (p<0.05). BMAP decreased from baseline following RE (p<0.05). No significant effects were observed for brachial diastolic BP and CDBP.
A time effect was observed for AIx@75bpm (p<0.05) (Fig. 2). During RIPC, AIx@75bpm went from –2.6±4.3 at baseline to 3.4±3.2 immediately after RE and to –0.3±3.6 and –1.4±3.9 at 30 minutes and 60 minutes after RE, respectively. During sham, AIx@75bpm went from 1.0±4.6 at baseline to 5.0±3.7 immediately after RE and to –1.5±3.6 and 0.3±4.4 at 30 minutes and 60 minutes after RE, respectively. However, no change was observed for cfPWV (Fig. 2). The cfPWV was 7.0±0.3 at baseline, 7.2±0.3 immediately after RE, 7.1±0.3 at 30 minutes after RE, and 7.0±0.3 at 60 minutes after RE, respectively. During the sham, cfPWV was 6.9±0.3 at baseline, 7.3±0.4 immediately after RE, 7.0±0.3 at 30 minutes after RE, and 6.9±0.3 at 60 minutes after RE, respectively.
The present study investigated whether RIPC before high-intensity RE protects against vascular deterioration following RE. The main findings of the present study were that acute high-intensity RE increased arterial stiffness, but this increase was not attenuated when RIPC of the upper limbs was applied before RE. To the best of our knowledge, this study is the first to demonstrate that RIPC did not attenuate the RE-mediated arterial stiffening when applied before RE in men.
In the present study, acute RE increased wave reflection and pulse wave velocity. This is consistent with recent findings that acute RE increases arterial stiffness in healthy populations4,5. The increased arterial stiffness following RE could be explained by mechanisms such as elevated sympathetic nerve activity22, or large increases in BP-mediated elastic fiber fatigue23. Also, acute RE may enhance oscillatory shear stress in conduit arteries that may be influenced by increases in BP24. Although it is not certain whether acutely increased arterial stiffness after high-intensity RE adversely affects cardiovascular health, an attenuation or prevention of the RE-associated increases in arterial stiffness should be needed. This is of particular importance since the number of young adults who participate in high-intensity RE is progressively increasing.
Interestingly, previous studies show that RIPC delays the development of exercise-associated fatigue25 and improves exercise performance18. A recent study reports that RIPC improves brachial artery flow-mediated dilation and skin microcirculation in healthy young individuals12, suggesting that RIPC may also have clinical relevance for vascular improvements. The mechanisms underlying these protective effects of RIPC have been attributed to the neural pathway, the release of circulating humoral factors, and the activation of a systemic protective effect26. Taken together, it is possible that RIPC may have a certain therapeutic strategy for protecting the systemic vasculature against transient vascular dysfunction induced by acute high-intensity RE. Despite the potential therapeutic effects of RIPC, we did not observe a reduction in arterial stiffness when RIPC was applied immediately before an acute bout of high-intensity RE. However, these observations do not rule out the possibility of vascular protective effects of RIPC.
One potential factor to consider contributing to this phenomenon could be the timing of the RIPC application. RIPC imposes a biphasic pattern, with the first wave of protection lasting up to 2 hours and the second window of protection covering 24 to 48 hours after the onset of the stimulus27. A recent study reports that RIPC was applied 100 minutes before exercise and prevented a reduction in brachial artery flow-mediated dilation after strenuous exercise17. Another study found that the application of RIPC 24 hours prior to angiography in patients with peripheral arterial disease significantly reduced the augmentation index, the same indices of arterial stiffness assessed in the present study28.
Although it is difficult to compare our findings with these findings due to differences in dependent variables, analysis of data regarding the relationship between RIPC and exercise performance suggests that the initial protective effects of RIPC appear within 3 hours. It was indicated that exercise performance improved when ischemic preconditioning was performed 30 to 90 minutes before exercise, rather than immediately prior to exercise29. Therefore, it is possible that the timing of RIPC application in this study was not optimal for demonstrating its vascular protective effects. It may also be considered a dose effect. Although it is somewhat difficult to determine without biological specimens or blood data, we can speculate that the dose of RIPC applied in this study was not sufficient to elicit a beneficial effect that would precede the effect of sympathoexcitation following RE. RIPC is generally repeated for three to four consecutive periods. However, different studies have made modifications in the protocol to attenuate injury or to induce protective effects30. Further research is needed to determine the optimal timing and protocol of RIPC to elucidate its protective effects against the decline in vascular function induced by high-intensity exercise.
Limitations to this study should be noted. Our sample included only healthy young subjects who were free from any clinical coronary heart disease, so we are unable to suggest that RIPC may not prevent the adverse effect of high-intensity RE in patients with cardiovascular disease in cardiac rehabilitation programs. Further studies are needed to test the association between RIPC and exercise in diseased populations. Although we had indices of arterial stiffness as the main variables, additional studies are needed to evaluate the effects of RIPC with other outcome variables. The present study investigated the acute effect of RIPC on RE-associated arterial stiffness, but the long-term effects of RIPC on arterial stiffness induced by repeated RE training are currently unknown. Further studies are needed to test whether repeated RIPC therapy can reduce high-intensity RE training-mediated arterial stiffening.
In conclusion, these findings demonstrate that acute RE increased arterial stiffness, but this increase was not attenuated by RIPC before RE. Our results suggest that RIPC prior to an acute bout of high-intensity RE may not protect against RE-induced arterial stiffening.
REFERENCES
1. Vlachopoulos C, Aznaouridis K, Stefanadis C. 2010; Prediction of cardiovascular events and all-cause mortality with arterial stiffness: a systematic review and meta-analysis. J Am Coll Cardiol. 55:1318–27. DOI: 10.1016/j.jacc.2009.10.061. PMID: 20338492.
2. Williams MA, Haskell WL, Ades PA, et al. 2007; Resistance exercise in individuals with and without cardiovascular disease: 2007 update: a scientific statement from the American Heart Association Council on Clinical Cardiology and Council on Nutrition, Physical Activity, and Metabolism. Circulation. 116:572–84. DOI: 10.1161/CIRCULATIONAHA.107.185214. PMID: 17638929.
3. Phillips SA, Das E, Wang J, Pritchard K, Gutterman DD. 2011; Resistance and aerobic exercise protects against acute endothelial impairment induced by a single exposure to hypertension during exertion. J Appl Physiol (1985). 110:1013–20. DOI: 10.1152/japplphysiol.00438.2010. PMID: 21252216. PMCID: PMC3075126.
4. DeVan AE, Anton MM, Cook JN, Neidre DB, Cortez-Cooper MY, Tanaka H. 2005; Acute effects of resistance exercise on arterial compliance. J Appl Physiol (1985). 98:2287–91. DOI: 10.1152/japplphysiol.00002.2005. PMID: 15718412.
5. Yoon ES, Jung SJ, Cheun SK, Oh YS, Kim SH, Jae SY. 2010; Effects of acute resistance exercise on arterial stiffness in young men. Korean Circ J. 40:16–22. DOI: 10.4070/kcj.2010.40.1.16. PMID: 20111648. PMCID: PMC2812793.
6. Failla M, Grappiolo A, Emanuelli G, et al. 1999; Sympathetic tone restrains arterial distensibility of healthy and atherosclerotic subjects. J Hypertens. 17:1117–23. DOI: 10.1097/00004872-199917080-00011. PMID: 10466467.
7. Hellsten Y, Frandsen U, Orthenblad N, Sjødin B, Richter EA. 1997; Xanthine oxidase in human skeletal muscle following eccentric exercise: a role in inflammation. J Physiol. 498(Pt 1):239–48. DOI: 10.1113/jphysiol.1997.sp021855. PMID: 9023782. PMCID: PMC1159248.
8. Barnes JN, Trombold JR, Dhindsa M, Lin HF, Tanaka H. 2010; Arterial stiffening following eccentric exercise-induced muscle damage. J Appl Physiol (1985). 109:1102–8. DOI: 10.1152/japplphysiol.00548.2010. PMID: 20671032.
9. Murry CE, Jennings RB, Reimer KA. 1986; Preconditioning with ischemia: a delay of lethal cell injury in ischemic myocardium. Circulation. 74:1124–36. DOI: 10.1161/01.CIR.74.5.1124. PMID: 3769170.
10. Hausenloy DJ, Yellon DM. 2008; Remote ischaemic preconditioning: underlying mechanisms and clinical application. Cardiovasc Res. 79:377–86. DOI: 10.1093/cvr/cvn114. PMID: 18456674.
11. Madias JE. 2011; Effect of serial arm ischemic preconditioning sessions on the systemic blood pressure of a normotensive subject. Med Hypotheses. 76:503–6. DOI: 10.1016/j.mehy.2010.12.002. PMID: 21194848.
12. Jones H, Hopkins N, Bailey TG, Green DJ, Cable NT, Thijssen DH. 2014; Seven-day remote ischemic preconditioning improves local and systemic endothelial function and microcirculation in healthy humans. Am J Hypertens. 27:918–25. DOI: 10.1093/ajh/hpu004. PMID: 24627443.
13. Manchurov V, Ryazankina N, Khmara T, et al. 2014; Remote ischemic preconditioning and endothelial function in patients with acute myocardial infarction and primary PCI. Am J Med. 127:670–3. DOI: 10.1016/j.amjmed.2014.02.012. PMID: 24565591.
14. Rassaf T, Totzeck M, Hendgen-Cotta UB, Shiva S, Heusch G, Kelm M. 2014; Circulating nitrite contributes to cardioprotection by remote ischemic preconditioning. Circ Res. 114:1601–10. DOI: 10.1161/CIRCRESAHA.114.303822. PMID: 24643960.
15. Jensen MD. 1999; Regional glycerol and free fatty acid metabolism before and after meal ingestion. Am J Physiol. 276:E863–9. DOI: 10.1152/ajpendo.1999.276.5.E863. PMID: 10329980.
16. World Medical Association. 2013; World Medical Association Declaration of Helsinki: ethical principles for medical research involving human subjects. JAMA. 310:2191–4. DOI: 10.1001/jama.2013.281053. PMID: 24141714.
17. Bailey TG, Birk GK, Cable NT, et al. 2012; Remote ischemic preconditioning prevents reduction in brachial artery flow-mediated dilation after strenuous exercise. Am J Physiol Heart Circ Physiol. 303:H533–8. DOI: 10.1152/ajpheart.00272.2012. PMID: 22730390.
18. de Groot PC, Thijssen DH, Sanchez M, Ellenkamp R, Hopman MT. 2010; Ischemic preconditioning improves maximal performance in humans. Eur J Appl Physiol. 108:141–6. DOI: 10.1007/s00421-009-1195-2. PMID: 19760432. PMCID: PMC2793394.
19. Baechle TR, Earle RW. National Strength & Conditioning Association. Essentials of strength training and conditioning. 3rd ed. Human Kinetics;2008. p. 641.
20. Van Bortel LM, Duprez D, Starmans-Kool MJ, et al. 2002; Clinical applications of arterial stiffness, Task Force III: recommendations for user procedures. Am J Hypertens. 15:445–52. DOI: 10.1016/S0895-7061(01)02326-3. PMID: 12022247.
21. Gallagher D, Adji A, O'Rourke MF. 2004; Validation of the transfer function technique for generating central from peripheral upper limb pressure waveform. Am J Hypertens. 17(11 Pt 1):1059–67. DOI: 10.1016/j.amjhyper.2004.05.027. PMID: 15533735.
22. Pratley R, Nicklas B, Rubin M, et al. 1994; Strength training increases resting metabolic rate and norepinephrine levels in healthy 50- to 65-yr-old men. J Appl Physiol (1985). 76:133–7. DOI: 10.1152/jappl.1994.76.1.133. PMID: 8175496.
23. Armentano RL, Levenson J, Barra JG, et al. 1991; Assessment of elastin and collagen contribution to aortic elasticity in conscious dogs. Am J Physiol. 260(6 Pt 2):H1870–7. DOI: 10.1152/ajpheart.1991.260.6.H1870. PMID: 1905490.
24. Padilla J, Young CN, Simmons GH, et al. 2010; Increased muscle sympathetic nerve activity acutely alters conduit artery shear rate patterns. Am J Physiol Heart Circ Physiol. 298:H1128–35. DOI: 10.1152/ajpheart.01133.2009. PMID: 20154260. PMCID: PMC2853421.
25. Barbosa TC, Machado AC, Braz ID, et al. 2015; Remote ischemic preconditioning delays fatigue development during handgrip exercise. Scand J Med Sci Sports. 25:356–64. DOI: 10.1111/sms.12229. PMID: 24731023.
26. Hausenloy DJ, Yellon DM. 2009; Preconditioning and postconditioning: underlying mechanisms and clinical application. Atherosclerosis. 204:334–41. DOI: 10.1016/j.atherosclerosis.2008.10.029. PMID: 19081095.
27. Loukogeorgakis SP, Panagiotidou AT, Broadhead MW, Donald A, Deanfield JE, MacAllister RJ. 2005; Remote ischemic preconditioning provides early and late protection against endothelial ischemia-reperfusion injury in humans: role of the autonomic nervous system. J Am Coll Cardiol. 46:450–6. DOI: 10.1016/j.jacc.2005.04.044. PMID: 16053957.
28. Kuusik K, Kepler T, Zilmer M, Eha J, Vähi M, Kals J. 2019; Effects of remote ischaemic preconditioning on arterial stiffness in patients undergoing lower limb angiographic procedures: a randomised clinical trial. Eur J Vasc Endovasc Surg. 58:875–82. DOI: 10.1016/j.ejvs.2019.06.004. PMID: 31648881.
29. Salvador AF, De Aguiar RA, Lisbôa FD, Pereira KL, Cruz RS, Caputo F. 2016; Ischemic preconditioning and exercise performance: a systematic review and meta-analysis. Int J Sports Physiol Perform. 11:4–14. DOI: 10.1123/ijspp.2015-0204. PMID: 26218309.
30. Randhawa PK, Bali A, Jaggi AS. 2015; RIPC for multiorgan salvage in clinical settings: evolution of concept, evidences and mechanisms. Eur J Pharmacol. 746:317–32. DOI: 10.1016/j.ejphar.2014.08.016. PMID: 25176179.
Fig. 1
Experimental design. RIPC: remote ischemic preconditioning, RE: resistance exercise, RM: repetition maximum, rep: repetition.
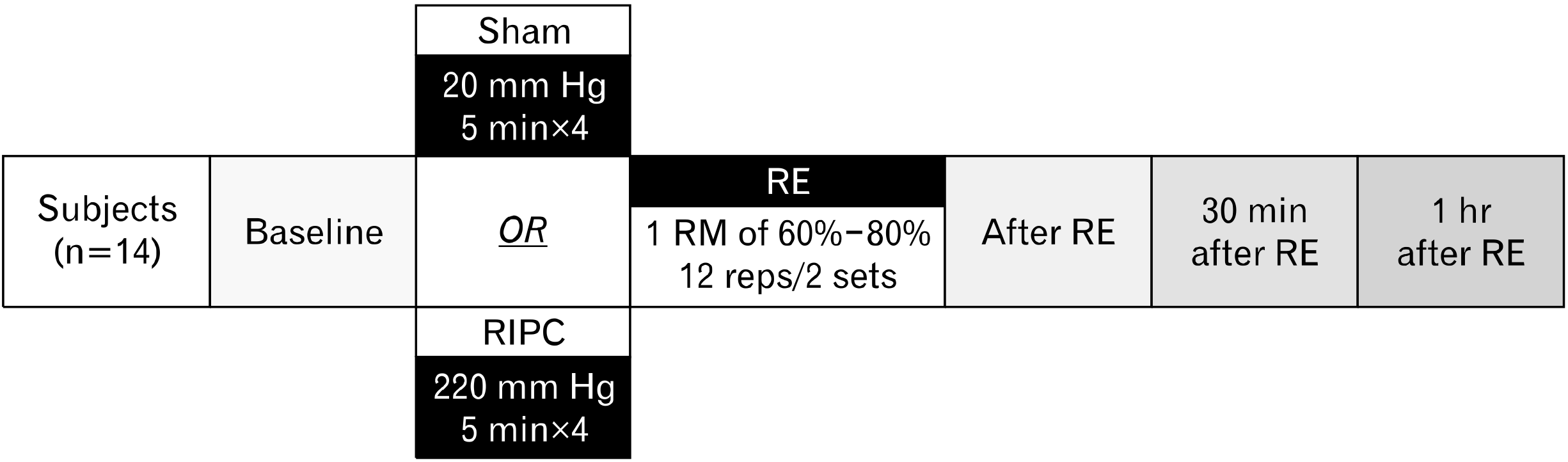
Fig. 2
Augmentation index at the heart rate of 75 beats per minute (AIx@75bpm) and carotid-femoral pulse wave velocity (cfPWV) at baseline, immediately after resistance exercise (RE), 30 minutes after RE, and 60 minutes after RE in remote ischemic preconditioning (RIPC) and sham trials. Values are mean±standard error. p<0.05, time effect.
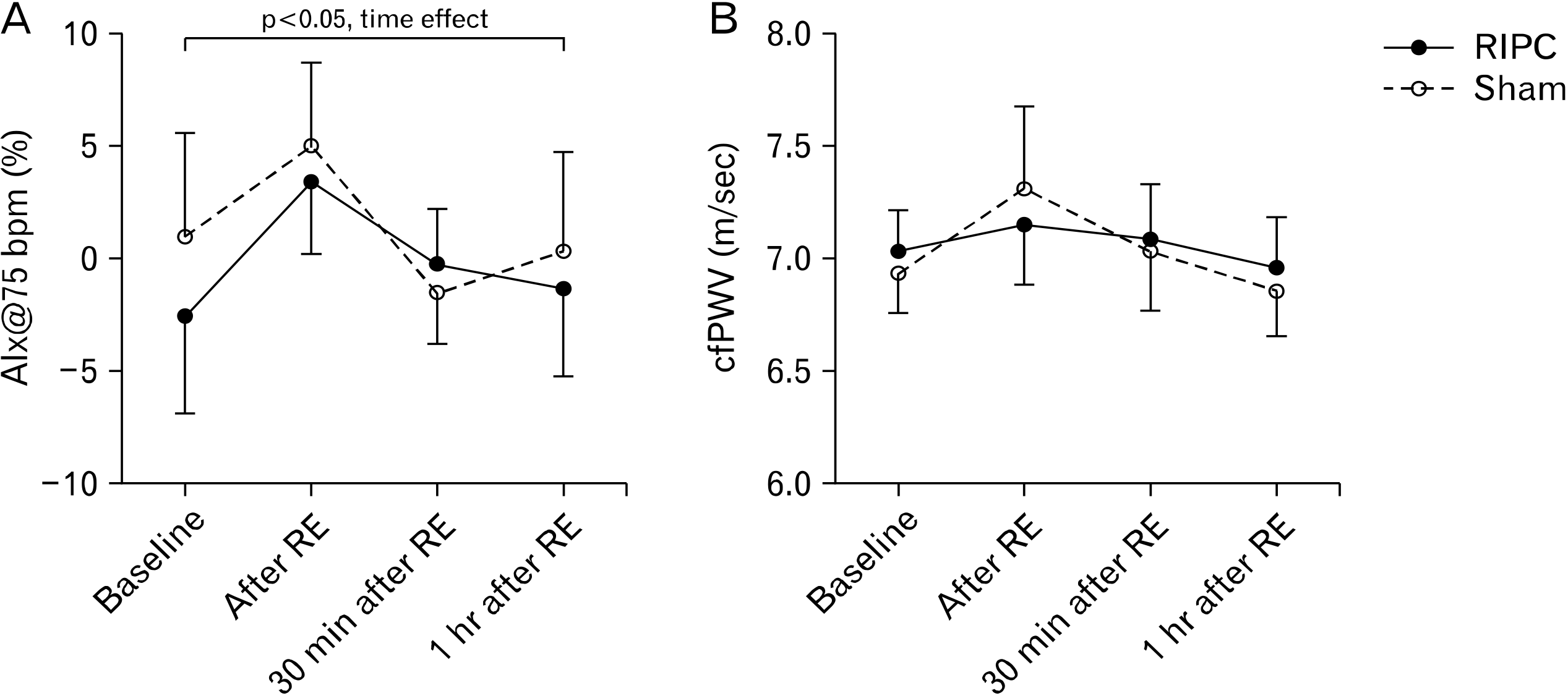
Table 1
Subject characteristics
Characteristic | Data |
---|---|
No. of subjects | 14 |
Sex, male:female | 8:6 |
Age (yr) | 26±1 |
Height (cm) | 170.2±2.0 |
Weight (kg) | 68.9±3.4 |
Body mass index (kg/m2) | 23.6±0.8 |
Table 2
HR and brachial and central blood pressure responses to RIPC and sham trials following RE
Variable | Baseline | Post-RE | ||
---|---|---|---|---|
Immediately | 30 min | 1 hr | ||
HR (beats/min)* | ||||
RIPC | 61±9 | 83±14 | 70±11 | 67±10 |
Sham | 64±10 | 82±15 | 73±13 | 66±11 |
BSBP (mm Hg)* | ||||
RIPC | 114±3 | 119±3 | 112±3 | 111±3 |
Sham | 115±3 | 118±3 | 111±3 | 111±2 |
BDBP (mm Hg) | ||||
RIPC | 71±2 | 69±2 | 69±2 | 70±2 |
Sham | 70±2 | 70±2 | 69±2 | 69±2 |
BMAP (mm Hg)* | ||||
RIPC | 86±2 | 85±2 | 83±2 | 83±2 |
Sham | 85±2 | 86±2 | 83±2 | 83±2 |
BPP (mm Hg)* | ||||
RIPC | 43±2 | 49±3 | 44±2 | 42±2 |
Sham | 45±2 | 48±2 | 42±3 | 43±2 |
CSBP (mm Hg)* | ||||
RIPC | 100±3 | 101±2 | 97±2 | 96±2 |
Sham | 100±3 | 103±2 | 96±2 | 96±2 |
CDBP (mm Hg) | ||||
RIPC | 72±2 | 72±3 | 70±2 | 70±2 |
Sham | 72±3 | 73±2 | 70±2 | 70±3 |
CMAP (mm Hg)* | ||||
RIPC | 81±2 | 82±2 | 79±2 | 79±2 |
Sham | 81±3 | 83±2 | 79±2 | 78±2 |
CPP (mm Hg)* | ||||
RIPC | 27±1 | 29±1 | 27±1 | 26±1 |
Sham | 28±1 | 30±1 | 26±1 | 26±1 |
HR: heart rate, RIPC: remote ischemic preconditioning, RE: resistance exercise, BSBP: brachial systolic blood pressure, BDBP: brachial diastolic blood pressure, BMAP: brachial mean arterial pressure, BPP: brachial pulse pressure, CSBP: central systolic blood pressure, CDBP: central diastolic blood pressure, CMAP: central mean arterial pressure, CPP: central pulse pressure.