Abstract
Intensive care unit (ICU) admissions in the United States exceed 5.7 million annually, often leading to complications such as post-intensive care syndrome and high mortality rates. Among these challenges, critical illness-related corticosteroid insufficiency (CIRCI) requires emphasis due to its complex, multiple-cause pathophysiology and varied presentations. CIRCI, characterized by adrenal insufficiency during critical illness, presents in up to 30% of ICU patients and may manifest as an exaggerated inflammatory response. Factors such as dysregulation of the hypothalamic-pituitary-adrenal (HPA) axis, altered cortisol metabolism, tissue corticosteroid resistance, and drug-induced suppression contribute to CIRCI. Diagnosis is a complex process, relying on a comprehensive assessment including clinical presentation, laboratory findings, and dynamic stimulatory testing. Treatment involves intensive medical care and exacting glucocorticoid therapy. Recent guidelines advocate for individualized approaches tailored to patient presentation and etiology. Understanding the pathophysiology and treatment of CIRCI is vital for clinicians managing critically ill patients and striving to improve outcomes. This research paper aims to explore the latest developments in the pathophysiology and management of CIRCI.
Critical illness encompasses a diverse range of severe conditions that require advanced organ support in the intensive care unit (ICU) and has an estimated prevalence of 45 million cases annually. Although it is a leading cause of death, critical illness is not a single condition and should be distinguished from the underlying disease diagnosis [1,2]. A proposed definition of critical illness is "a state of ill health with the potential for reversibility characterized by vital organ dysfunction and a high risk of imminent death if care is not provided" [3]. The duration and severity of critical illness are determined by its etiology, which includes systemic inflammatory response syndrome, compensatory anti-inflammatory response syndrome, cytokine release syndrome, acute respiratory distress syndrome (ARDS), multiple organ failure, persistent inflammation, immunosuppression, and catabolism syndrome [4]. Critical illnesses are marked by heightened systemic cortisol levels, an integral component of the stress response. In instances of acute stress, the risk of an adrenal crisis is elevated. This risk is increased further with underlying hypothalamic-pituitary-adrenal (HPA) axis pathology inadequately managed with stress doses of hydrocortisone [5]. Cortisol, a glucocorticoid hormone, is a primary product of the HPA axis that serves as a vital component of the biological stress response system. Cortisol dysregulation is implicated in various mental and physical health outcomes [6]. Glucocorticoids, including endogenous cortisol, exert predominantly anti-inflammatory and immunosuppressive effects by inhibiting the production and activity of inflammatory cells and promoting their redistribution to other body compartments, resulting in decreased circulating immune cells overall [7]. These mechanisms may contribute to mediating associations between stress exposure and subsequent developmental and health outcomes, impacting the onset and progression of both mental and physical health disorders. Corticosteroids are efficiently absorbed in the gastrointestinal tract, exhibit high protein binding, undergo hepatic metabolism, and are excreted by the kidneys [8].
Critical illness-related corticosteroid insufficiency (CIRCI) is characterized by adrenal insufficiency (AI) due to impaired functioning of the HPA axis, altered cortisol metabolism, and tissue corticosteroid resistance. These result in an exaggerated prolonged proinflammatory response, especially in ICU patients [9-13] in whom the incidence of CIRCI varies widely and ranges from 0% to 30%. In specific patient populations such as those with septic shock or trauma, the incidence can be as high as 25% to 40% and 50% to 70%, respectively [10,14,15]. In addition to an elevated 28-day mortality rate compared to non-CIRCI patients, a mortality rate of 34% among post-trauma CIRCI patients was reported. Non-survivors were older (65 years and older); had more severe base deficits at admission; required prolonged ventilatory days; and had increased rates of sepsis, bacteremia, and pneumonia [15-17].
CIRCI is a common and complex phenomenon, the understanding of which is evolving [10]. A study conducted in 2021 examined medical practitioners' approaches to CIRCI management, and 72% of respondents reported having to address CIRCI at least one weekly. However, the utilization of formal protocols for CIRCI diagnosis was limited, and a notable portion of practitioners forgo laboratory testing for this purpose. Moreover, a substantial majority expressed uncertainty regarding the therapeutic role of corticosteroids in CIRCI cases [14]. Therefore, given the CIRCI prevalence in various critical conditions, medical professional education on its pathophysiology and treatment is imperative [10].
Critical illness elicits a significant physiological stress response, prompting the body to activate various mechanisms to maintain homeostasis [18,19]. One crucial mechanism involves the heightened activity of the HPA axis, which rapidly increases systemic glucocorticoid levels that are essential for survival [20]. The classic model describes that the stressors in critically ill patients consist of neuronal and inflammatory signals that act on the hypothalamic nucleus paraventricularis to release corticotropin-releasing hormone (CRH) and arginine vasopressin (AVP). The CRH and AVP are transported to the anterior pituitary to trigger the release of adrenocorticotropic hormone (ACTH) into the systemic circulation from corticotrope cells [21]. Under the influence of ACTH, the adrenal glands synthesize glucocorticoids (cortisol), mineralocorticoids (aldosterone), and adrenal androgens (dehydroepiandrosterone) from cholesterol. Adrenal mitochondria also play a pivotal role in steroidogenesis within adrenocortical cells, facilitating the conversion of intracellular cholesterol into cortisol [22]. The cortisol then exerts its systemic effects on numerous cells and modulates inflammation, cardiovascular function, and metabolism. However, in recent years, this traditional model has faced scrutiny, and it has been shown that elevated cortisol level in critically ill patients does not necessarily coincide with high concentration of plasma ACTH [21,23-25]. Dysregulation of the HPA axis, altered cortisol metabolism, and tissue resistance to glucocorticoids are now thought to be the three main pathophysiologic events in CIRCI [12].
The classic activation model of the central HPA axis is characterized by elevated ACTH level driving heightened adrenocortical cortisol production in response to sepsis or critical illness [21]. An increase in plasma ACTH has only been observed temporarily in critically ill patients, after which, at least during the initial week in the ICU, plasma ACTH is consistently maintained below normal levels [26]. Recent research indicates that patients who spend four weeks or more in the ICU may experience suppression of the HPA axis that resembles central AI [27]. When the HPA axis stress response becomes dysregulated in intensity or duration, the equilibrium is disrupted, leading to CIRCI. Detrimental effects on fundamental bodily processes such as growth, metabolism, circulation, reproduction, immune response, cognition, and behavior follow [28]. Disruption of the HPA axis during stress also affects mitochondrial function, compromising their vital roles in energy production and synthesis of lipids, heme, purine, and steroids to exacerbate metabolic disturbances [29]. Under conditions of severe stress, responses typically deemed adaptive may prove to be inadequate or even detrimental [11].
The regulation of cortisol production during critical illness is complex and involves intricate interactions among the CRH/ACTH pathways, autonomic nervous system, vasopressin system, and immune system. This includes disruption of the normal circadian rhythm of cortisol secretion due to rapid ACTH release via CRH and AVP mechanisms [30]. Initially, there is reduced cortisol breakdown in the liver and kidneys during critical illness. This is attributed to suppressed expression and activity of cortisol-metabolizing enzymes with an extension of cortisol half-life and hypercortisolemia [26,31]. Additionally, cortisol is released from the plasma binding proteins transcortin and albumin due to decreased hepatic synthesis of and decreased cortisol binding to these proteins [32,33].
However, although there is an initial activation of the pituitary glucocorticoid receptor and increased circulating availability of free cortisol, this eventually results in impaired processing of pro-opiomelanocortin (POMC) into ACTH and decreased secretion of mature ACTH. This response is due to a negative feedback loop via expression of annexin A1 [26,34]. The negative feedback inhibition at the pituitary level may also involve the binding of free cortisol to glucocorticoid receptors or binding of other ligands of glucocorticoid receptors such as bile acids [5]. The reduced expression of the enzyme prohormone convertase 1 during sepsis also contributes to the decreased conversion of POMC into ACTH [26].
CIRCI is mediated by tissue resistance to glucocorticoids. A transient increase in the expression of glucocorticoid receptor beta (GRβ) is present in mononuclear cells from septic patients and is accompanied by a simultaneous increase in dominant negative GRβ expression. Additionally, serum obtained from septic patients induces glucocorticoid resistance in cell cultures, suggesting a potential mechanism for cell-level resistance to glucocorticoids in sepsis [35]. Furthermore, sepsis is characterized by a decline in glucocorticoid receptor alpha (GRα) levels in circulating, liver, and muscle tissues, with reduced GRα transcription in various tissues including circulating, lymph nodes/spleen, liver, kidney, and lung cells and tissues. This reduction in GRα is paralleled by an increase in the expression of GRβ isoforms, leading to an imbalance between GRα and GRβ [36]. Moreover, glucocorticoid therapy induces the expression of mitochondrial receptor-124, which downregulates GRα and limits the anti-inflammatory effects of glucocorticoids. These observations suggest that steroid treatment may exacerbate glucocorticoid resistance, particularly in patients with elevated GRβ [37]. These imbalances in GRα and GRβ expression are associated with increased sickness sensitivity and reduced glucocorticoid sensitivity, both of which contribute to corticosteroid resistance at the tissue level [38].
Certain medications administered during critical illnesses, including glucocorticoids, opioids, azole antifungals like ketoconazole, and psychoactive substances can potentially suppress the synthesis of ACTH [39]. Administration of exogenous glucocorticoids exerts negative feedback control on the HPA axis by suppressing the production of CRH in the hypothalamus and ACTH secretion in the pituitary gland. This suppression leads to hypotrophy and atrophy of the adrenal cortex, leading to a significant decrease in cortisol production while maintaining mineralocorticoid activity [40]. Consequently, this results in AI, with the adrenal glands becoming incapable of producing sufficient levels of cortisol after cessation of glucocorticoid therapy. Abrupt cessation or rapid withdrawal of glucocorticoid therapy may precipitate symptoms of AI [41,42]. Opioids may induce AI in a similar fashion by primarily inhibiting the HPA axis at the level of the hypothalamus, decreasing cortisol production [42-44]. Benzodiazepines contribute to the rapid decline in cortisol production by inhibiting functions across various central and peripheral points of the HPA axis. These points include the activities of adrenal microsomal 17- and 21-hydroxylase and 11-β-hydroxylase in the adrenal mitochondria [12]. Administration of etomidate inhibits the final enzymatic step in cortisol synthesis and markedly increases the likelihood of AI at both four-to-six-hour and 12-hour post-administration in critically ill patients [45]. Ketoconazole, an imidazole antifungal medication and a treatment option for Cushing’s syndrome, targets and inhibits crucial enzymes in adrenal steroidogenesis. Acute AI also has been observed in patients treated with fluconazole, another antifungal agent, especially among those who are critically ill (Figure 1) [46-49].
CIRCI in the context of prolonged critical illness poses significant challenges in diagnosis due to overlapping signs and symptoms with the expected clinical course in these patients. These signs and symptoms include fatigue, weight loss, syncope, fever, confusion, somnolence, back and leg cramps, postural dizziness, and hypotension. In severe cases, hypovolemic shock and coma may occur [5,50]. Features such as encephalopathy, poor neurological recovery after sedation cessation, and persistent need for vasopressors should raise suspicion for CIRCI [5]. Additionally, a reduction in adrenal gland volume to less than 10 cm3, found in some patients with septic shock, has been linked to increased mortality [51].
The Endocrine Society advises stimulatory testing for cortisol deficiency in suspected primary adrenal insufficiency (PAI) cases. Confirmation of PAI typically involves morning cortisol level <140 nmol/l with elevated plasma ACTH, followed by the 250 μg Synacthen stimulation test [26,52,53]. ACTH measurement aids in distinguishing primary from secondary or tertiary AI. An ACTH value > 66 pmol/l is suggestive of PAI in patients with confirmed hypocortisolemia [52]. Stimulatory testing, using both low and high Synacthen doses, aids in diagnosis. Simultaneous renin and aldosterone measurement is recommended for mineralocorticoid deficiency confirmation [52,54]. Secondary AI diagnosis involves dynamic stimulatory testing; the insulin tolerance test is the gold standard for this [55]. The American College of Critical Care Medicine suggests considering AI in patients with random cortisol <10 μg/dl (275.9 nmol/L) [11-12,54].
However, diagnosing CIRCI is more complex. While a low random plasma cortisol concentration may raise suspicion, there is no clearly established guidelines for definitive CIRCI diagnosis [5,52]. Critically ill patients requiring mechanical and pharmacological support in the ICU for four weeks or longer, especially those with hemodynamic instability despite adequate resuscitation and vasopressor support, should be considered at risk for CIRCI. Furthermore, exposure to medications that affect the HPA axis or cortisol synthesis should heighten suspicion for CIRCI [5,54]. Laboratory findings in CIRCI may include hyponatremia, elevated creatinine levels, normochromic anemia, lymphocytosis, eosinophilia, hypoglycemia, and mild hypercalcemia [50]. Patients with CIRCI may also exhibit normal or low cortisol levels, low albumin and cortisol-binding globulin levels, normal or low ACTH levels, and high bile acid and bilirubin levels [5,21,25,56]. Elevated inflammatory cytokine levels, such as those for tumor necrosis factor, interleukin (IL)-1β, and IL-6, at ICU admission and throughout the ICU stay correlate with disease severity and increased mortality risk [57].
A total serum cortisol of <9 μg/dl post-ACTH stimulation or a random total cortisol level <10 μg/dl was considered reliable for diagnosing AI in critically ill patients according to the 2008 task force guidelines by the American College of Critical Care Medicine [11]. However, these criteria were not widely adopted in clinical practice, and recent guideline updates in 2017 do not include the use of these specific tests. Instead, the Endocrine Society confirms that the high-dose (250 μg) ACTH stimulation test is superior to other tests such as plasma or serum free cortisol, salivary free cortisol, low-dose ACTH stimulation, hydrocortisone levels (50–300 mg), and corticotropin levels in diagnosing CIRCI. Peak plasma total cortisol level <18 μg/dL at 30 or 60 minutes on high-dose ACTH stimulation is considered confirmatory in diagnosing CIRCI. Despite these suggestions, new pathophysiological evidence of CIRCI has had diagnostic implications. For example, the increase in distribution cortisol volume and the suppression of cortisol-binding globulin have invalidated the use of the classic 250 µg ACTH stimulation test [26,53,58]. Due to these pathophysiological changes, the stimulation test result is confounded as the incremental response of total plasma cortisol to the ACTH injection is suppressed. Although the ACTH stimulation test cannot be used as a marker of AI, a low cortisol response does reflect increased severity of the illness [25-26,59]. Random total cortisol concentrations are also unlikely to provide useful information to clinicians for diagnosis as they vary widely between patients and even within a single patient [26,53]. Additionally, specific imaging techniques like bedside ultrasound, computed tomography, or magnetic resonance imaging can assist in diagnosing CIRCI by distinguishing it from rare complications of critical illnesses, such as adrenal hemorrhage [38].
Although there are many findings that suggest the diagnosis, there is no single diagnostic test or imaging technique to reliably diagnose CIRCI [5]. When diagnosing CIRCI in critically ill patients, a comprehensive approach that considers clinical presentation, laboratory findings, and dynamic stimulatory testing is required. Acknowledging the challenges posed by altered cortisol dynamics and the evolving understanding of CIRCI pathophysiology also are required [26,53,58,59]. A better understanding of the presentation and diagnosis of AI in critically ill patients can help clinicians manage patients effectively and improve outcomes.
Once CIRCI is accurately diagnosed, treatment with intensive medical care and hydrocortisone is recommended. However, the exact hydrocortisone dose that is needed to manage CIRCI and prevent mortality is uncertain [5,21,60]. Traditionally, the recommendation was to administer doses ranging from 200 to 400 mg per day to critically ill patients in the ICU. This is equivalent to approximately 10 to 20 times the standard substitution or replacement dose [5,61,62]. The assumption behind this was that the dose would meet the daily cortisol requirement necessary to generate the elevated plasma concentration associated with severe stress in healthy HPA axis individuals [5,63,64].
A 2008 study by Marik et al. [11] recommended a hydrocortisone management regimen for CIRCI in septic shock cases. This recommendation was to administer 200 mg/day hydrocortisone in four divided doses or a continuous infusion at a rate of 240 mg/day (10 mg/hr), for at least 7 days in cases of septic shock. The study also recommended methylprednisolone at a daily dose of 1 mg per kg of body weight for at least 14 days, specifically for patients with severe early ARDS. Gradual glucocorticoid tapering, rather than abrupt discontinuation, is necessary. If symptoms of sepsis, hypotension, or worsening oxygenation recur, treatment should be reinstated. Dexamethasone is not recommended for treatment of CIRCI [11].
In a 2017 study, these guidelines were updated by a multi-specialty task force of 16 international experts in critical care medicine and endocrinology. Their recommendations were based on the evidence cited in the 2008 study and more recent findings from an updated systematic review. The recommendations were formulated using the Grading of Recommendations Assessment, Development, and Evaluation methodology and required approval by 80% of the task force members. This study further emphasized the need for an individualized approach that considers the patient's presentation, etiology, vital signs, and responsiveness to vasopressors and fluids in various critical illness conditions. For patients suffering from septic shock and not responding to standard treatments such as fluid resuscitation and vasopressor therapy, intravenous (IV) hydrocortisone at a dosage below 400 mg/day for a minimum of 3 days is recommended [12]. A 2018 study found that a hydrocortisone plus fludrocortisone regimen resulted in lower 90-day all-cause mortality compared to placebo in patients with septic shock [65]. Similarly, for patients with early moderate to severe ARDS, IV methylprednisolone at a dose of 1 mg/kg/day is suggested. For patients hospitalized with community-acquired pneumonia, corticosteroids for 5–7 days at a daily dose less than 400 mg IV hydrocortisone or its equivalent is recommended. Corticosteroids are also recommended for patients with bacterial meningitis and those undergoing cardiopulmonary bypass surgery. In the context of cardiac arrest, the use of corticosteroids is suggested. However, caution is needed when using corticosteroids in cases of major trauma and in adult patients with sepsis without shock. Several trials have shown no clear benefit in these cases, and the risks may outweigh benefits in such patients. Corticosteroids should not be administered in adults with influenza [12,66]. A 2022 study by Téblick et al. [5] proposed a more generalized approach for managing CIRCI, advising a daily hydrocortisone regimen of 60 mg split into two IV boluses, 40 mg in the morning and 20 mg in the evening. This replicates the normal diurnal rhythm that is disrupted in critically ill patients. Once corticosteroid treatment for CIRCI is initiated, administration should be tapered to the lowest effective maintenance dose. Discontinuation of the medication should be achieved as soon as possible [5,31,67].
The administration of corticosteroids in critically ill patients is associated with significant adverse effects that necessitate careful consideration. These include hyperglycemia due to disrupted glucose homeostasis, hypernatremia and fluid retention from altered renal function, and increased susceptibility to nosocomial infections due to immunosuppression. Corticosteroid use has also been linked to neuropsychiatric complications such as delirium and the development of ICU-acquired weakness [68]. However, emerging evidence suggests that the risk of ICU-acquired weakness may be mitigated by lower steroid dosages, better glycemic control, and reduced sedation. Thus, the appropriate steroid dosage balancing the therapeutic benefits with these potential adverse effects is crucial (Table 1) [69-71].
The increasing rate of ICU admissions highlights the importance of recognizing complications such as CIRCI. CIRCI presents a difficult diagnostic and therapeutic challenge in ICUs, necessitating a comprehensive understanding of its latest pathophysiology and evolving treatment strategies. CIRCI is characterized by AI and is triggered by use of certain medications and by various pathophysiological changes that occur in critically ill patients. These pathophysiological changes include dysfunction of the HPA axis, altered cortisol metabolism, and increased tissue resistance to cortisol. Diagnosing CIRCI requires evaluation of the patient's clinical signs and symptoms, laboratory results, and dynamic testing results. Although there is controversy regarding the exact recommended doses, glucocorticoid treatment approaches have evolved from traditional and generalized to individualized regimens that are specifically tailored to the patient's condition. Updates in treatment guidelines for CIRCI demonstrate ongoing research by incorporating the latest pathophysiological evidence and insights from various clinical trials. The aim is to improve management of critically ill patients. However, further research is needed, particularly randomized clinical trials investigating the short- and long-term outcomes of suggested treatments. Optimization of dosing and tapering strategies is also required. By enhancing understanding of CIRCI, clinicians can optimize patient care and mitigate the detrimental effects of this complex condition in the ICU setting.
▪ Critical illness-related corticosteroid insufficiency (CIRCI), with an incidence of up to 30% in intensive care unit admissions, is defined as adrenal insufficiency in critically ill patients.
▪ The pathophysiology of CIRCI is characterized by dysfunction of the hypothalamic-pituitary-adrenal axis, altered cortisol metabolism, and increased tissue resistance to cortisol and may be associated with use of certain medications.
▪ Treatment should be individualized, tailored to patient presentation, and may include fluids, vasopressors, intravenous (IV) methylprednisolone, and IV hydrocortisone
REFERENCES
1. Stewart J, Bradley J, Smith S, McPeake J, Walsh T, Haines K, et al. Do critical illness survivors with multimorbidity need a different model of care? Crit Care. 2023; 27:485.
2. Adhikari NK, Fowler RA, Bhagwanjee S, Rubenfeld GD. Critical care and the global burden of critical illness in adults. Lancet. 2010; 376:1339–46.
3. Kayambankadzanja RK, Schell CO, Gerdin Wärnberg M, Tamras T, Mollazadegan H, Holmberg M, et al. Towards definitions of critical illness and critical care using concept analysis. BMJ Open. 2022; 12:e060972.
4. Parfenov AL, Razzhivin VP, Petrova MV. Chronic critical illness: current aspects of the problem (review). Sovrem Tekhnologii Med. 2022; 14:70–81.
5. Téblick A, Gunst J, Van den Berghe G. Critical illness-induced corticosteroid insufficiency: what it is not and what it could be. J Clin Endocrinol Metab. 2022; 107:2057–64.
6. Adam EK, Quinn ME, Tavernier R, McQuillan MT, Dahlke KA, Gilbert KE. Diurnal cortisol slopes and mental and physical health outcomes: a systematic review and meta-analysis. Psychoneuroendocrinology. 2017; 83:25–41.
7. Ericson-Neilsen W, Kaye AD. Steroids: pharmacology, complications, and practice delivery issues. Ochsner J. 2014; 14:203–7.
8. Kapugi M, Cunningham K. Corticosteroids. Orthop Nurs. 2019; 38:336–9.
9. Bagate F, Bedet A, Tomberli F, Boissier F, Razazi K, de Prost N, et al. Critical illness-related corticosteroid insufficiency during difficult weaning from mechanical ventilation. Ann Intensive Care. 2021; 11:65.
10. Qutob RA, Alaryni A, Alghamdi A, Alsolamy EN, Al Harbi K, Alammari Y, et al. A cross-sectional survey study on the diagnosis and management of critical illness-induced corticosteroid insufficiency in Saudi Arabia. Cureus. 2023; 15:e49982.
11. Marik PE, Pastores SM, Annane D, Meduri GU, Sprung CL, Arlt W, et al. Recommendations for the diagnosis and management of corticosteroid insufficiency in critically ill adult patients: consensus statements from an international task force by the American College of Critical Care Medicine. Crit Care Med. 2008; 36:1937–49.
12. Annane D, Pastores SM, Arlt W, Balk RA, Beishuizen A, Briegel J, et al. Critical illness-related corticosteroid insufficiency (CIRCI): a narrative review from a Multispecialty Task Force of the Society of Critical Care Medicine (SCCM) and the European Society of Intensive Care Medicine (ESICM). Intensive Care Med. 2017; 43:1781–92.
13. Chaudhuri D, Nei AM, Rochwerg B, Balk RA, Asehnoune K, Cadena R, et al. 2024 Focused update: guidelines on use of corticosteroids in sepsis, acute respiratory distress syndrome, and community-acquired pneumonia. Crit Care Med. 2024; 52:e219–33.
14. Huang CS, Miles T, Thomas B, Todd SR, Wilson CT, Suliburk J. A call for standardization: practice patterns and management of critical illness-related corticosteroid insufficiency in surgical intensive care units. Injury. 2021; 52:2522–5.
15. Yang Y, Liu L, Jiang D, Wang J, Ye Z, Ye J, et al. Critical illness-related corticosteroid insufficiency after multiple traumas: a multicenter, prospective cohort study. J Trauma Acute Care Surg. 2014; 76:1390–6.
16. Walker ML, Owen PS, Sampson C, Marshall J, Pounds T, Henderson VJ. Incidence and outcomes of critical illness-related corticosteroid insufficiency in trauma patients. Am Surg. 2011; 77:579–85.
17. Inaba M, Naito H, Yorifuji T, Nakamichi C, Maeyama H, Ishikawa H, et al. Impact of frailty on long-term mortality in older patients receiving intensive care via the emergency department. Sci Rep. 2023; 13:5433.
18. Bernard C. Leçons sur les phénomènes de la vie communs aux animaux et aux végétaux. Librairie J.-B. Baillière et fils;1878.
19. Ingels C, Vanhorebeek I, Van den Berghe G. Glucose homeostasis, nutrition and infections during critical illness. Clin Microbiol Infect. 2018; 24:10–5.
20. Vermes I, Beishuizen A. The hypothalamic-pituitary-adrenal response to critical illness. Best Pract Res Clin Endocrinol Metab. 2001; 15:495–511.
21. Téblick A, Peeters B, Langouche L, Van den Berghe G. Adrenal function and dysfunction in critically ill patients. Nat Rev Endocrinol. 2019; 15:417–27.
22. Midzak A, Papadopoulos V. Adrenal mitochondria and steroidogenesis: from individual proteins to functional protein assemblies. Front Endocrinol (Lausanne). 2016; 7:106.
23. Boonen E, Meersseman P, Vervenne H, Meyfroidt G, Guïza F, Wouters PJ, et al. Reduced nocturnal ACTH-driven cortisol secretion during critical illness. Am J Physiol Endocrinol Metab. 2014; 306:E883–92.
24. Peeters B, Meersseman P, Vander Perre S, Wouters PJ, Vanmarcke D, Debaveye Y, et al. Adrenocortical function during prolonged critical illness and beyond: a prospective observational study. Intensive Care Med. 2018; 44:1720–9.
25. Peeters B, Meersseman P, Vander Perre S, Wouters PJ, Debaveye Y, Langouche L, et al. ACTH and cortisol responses to CRH in acute, subacute, and prolonged critical illness: a randomized, double-blind, placebo-controlled, crossover cohort study. Intensive Care Med. 2018; 44:2048–58.
26. Van den Berghe G, Téblick A, Langouche L, Gunst J. The hypothalamus-pituitary-adrenal axis in sepsis- and hyperinflammation-induced critical illness: gaps in current knowledge and future translational research directions. EBioMedicine. 2022; 84:104284.
27. Jameson JL, De Groot LJ, de Kretser DM, Giudice LC, Grossman AB, Melmed S, et al. Endocrinology: adult and pediatric. Elsevier Saunders;2016.
28. Nicolaides NC, Kyratzi E, Lamprokostopoulou A, Chrousos GP, Charmandari E. Stress, the stress system and the role of glucocorticoids. Neuroimmunomodulation. 2015; 22:6–19.
29. Lee SR, Kim HK, Song IS, Youm J, Dizon LA, Jeong SH, et al. Glucocorticoids and their receptors: insights into specific roles in mitochondria. Prog Biophys Mol Biol. 2013; 112:44–54.
30. Vassiliadi DA, Dimopoulou I, Tzanela M, Douka E, Livaditi O, Orfanos SE, et al. Longitudinal assessment of adrenal function in the early and prolonged phases of critical illness in septic patients: relations to cytokine levels and outcome. J Clin Endocrinol Metab. 2014; 99:4471–80.
31. Boonen E, Vervenne H, Meersseman P, Andrew R, Mortier L, Declercq PE, et al. Reduced cortisol metabolism during critical illness. N Engl J Med. 2013; 368:1477–88.
32. Cameron A, Henley D, Carrell R, Zhou A, Clarke A, Lightman S. Temperature-responsive release of cortisol from its binding globulin: a protein thermocouple. J Clin Endocrinol Metab. 2010; 95:4689–95.
33. Jenniskens M, Weckx R, Dufour T, Vander Perre S, Pauwels L, Derde S, et al. The hepatic glucocorticoid receptor is crucial for cortisol homeostasis and sepsis survival in humans and male mice. Endocrinology. 2018; 159:2790–802.
34. Téblick A, Vander Perre S, Pauwels L, Derde S, Van Oudenhove T, Langouche L, et al. The role of pro-opiomelanocortin in the ACTH-cortisol dissociation of sepsis. Crit Care. 2021; 25:65.
35. Guerrero J, Gatica HA, Rodríguez M, Estay R, Goecke IA. Septic serum induces glucocorticoid resistance and modifies the expression of glucocorticoid isoforms receptors: a prospective cohort study and in vitro experimental assay. Crit Care. 2013; 17:R107.
36. van den Akker EL, Koper JW, Joosten K, de Jong FH, Hazelzet JA, Lamberts SW, et al. Glucocorticoid receptor mRNA levels are selectively decreased in neutrophils of children with sepsis. Intensive Care Med. 2009; 35:1247–54.
37. Ledderose C, Möhnle P, Limbeck E, Schütz S, Weis F, Rink J, et al. Corticosteroid resistance in sepsis is influenced by microRNA-124: induced downregulation of glucocorticoid receptor-α. Crit Care Med. 2012; 40:2745–53.
38. Cohen J, Pretorius CJ, Ungerer JP, Cardinal J, Blumenthal A, Presneill J, et al. Glucocorticoid sensitivity is highly variable in critically ill patients with septic shock and is associated with disease severity. Crit Care Med. 2016; 44:1034–41.
39. Bornstein SR. Predisposing factors for adrenal insufficiency. N Engl J Med. 2009; 360:2328–39.
40. Borresen SW, Klose M, Glintborg D, Watt T, Andersen MS, Feldt-Rasmussen U. Approach to the patient with glucocorticoid-induced adrenal insufficiency. J Clin Endocrinol Metab. 2022; 107:2065–76.
41. Charmandari E, Nicolaides NC, Chrousos GP. Adrenal insufficiency. Lancet. 2014; 383:2152–67.
42. Feng Y, Chang P, Liu J, Zhang WS. Effects and mechanisms of perioperative medications on the hypothalamic pituitary adrenal response to surgical injury: a narrative review. J Clin Anesth. 2024; 94:111367.
43. Daniell HW. Opioid contribution to decreased cortisol levels in critical care patients. Arch Surg. 2008; 143:1147–8.
44. Donegan D. Opioid induced adrenal insufficiency: what is new? Curr Opin Endocrinol Diabetes Obes. 2019; 26:133–8.
45. Bruder EA, Ball IM, Ridi S, Pickett W, Hohl C. Single induction dose of etomidate versus other induction agents for endotracheal intubation in critically ill patients. Cochrane Database Syst Rev. 2015; 1:CD010225.
46. Freyer CW, Loren AW. Fluconazole-Induced adrenal insufficiency following allogeneic hematopoietic cell transplant. J Oncol Pharm Pract. 2022; 28:1922–5.
47. Benitez LL, Carver PL. Adverse effects associated with long-term administration of azole antifungal agents. Drugs. 2019; 79:833–53.
48. Nieman LK, Biller BM, Findling JW, Murad MH, Newell-Price J, Savage MO, et al. Treatment of Cushing’s syndrome: an endocrine society clinical practice guideline. J Clin Endocrinol Metab. 2015; 100:2807–31.
49. Choo KS, Yew J, Tan EJ, Puar TH. Case report: hypercalcemia as a manifestation of acute adrenal crisis precipitated by fluconazole use, and a review of the literature. Front Endocrinol (Lausanne). 2023; 14:1168797.
50. Arlt W; Society for Endocrinology Clinical Committee. Society for Endocrinology Endocrine Emergency Guidance: emergency management of acute adrenal insufficiency (adrenal crisis) in adult patients. Endocr Connect. 2016; 5:G1–3.
51. Jung B, Nougaret S, Chanques G, Mercier G, Cisse M, Aufort S, et al. The absence of adrenal gland enlargement during septic shock predicts mortality: a computed tomography study of 239 patients. Anesthesiology. 2011; 115:334–43.
52. Bornstein SR, Allolio B, Arlt W, Barthel A, Don-Wauchope A, Hammer GD, et al. Diagnosis and treatment of primary adrenal insufficiency: an Endocrine Society Clinical Practice Guideline. J Clin Endocrinol Metab. 2016; 101:364–89.
53. Cohen J, Venkatesh B. Relative adrenal insufficiency in the intensive care population: background and critical appraisal of the evidence. Anaesth Intensive Care. 2010; 38:425–36.
54. Martin-Grace J, Dineen R, Sherlock M, Thompson CJ. Adrenal insufficiency: physiology, clinical presentation and diagnostic challenges. Clin Chim Acta. 2020; 505:78–91.
55. Bancos I, Hahner S, Tomlinson J, Arlt W. Diagnosis and management of adrenal insufficiency. Lancet Diabetes Endocrinol. 2015; 3:216–26.
56. Jenniskens M, Güiza F, Oorts M, Vander Perre S, Derde S, Dufour T, et al. On the role of illness duration and nutrient restriction in cholestatic alterations that occur during critical illness. Shock. 2018; 50:187–98.
57. Meduri GU, Muthiah MP, Carratu P, Eltorky M, Chrousos GP. Nuclear factor-kappaB- and glucocorticoid receptor alpha- mediated mechanisms in the regulation of systemic and pulmonary inflammation during sepsis and acute respiratory distress syndrome. Evidence for inflammation-induced target tissue resistance to glucocorticoids. Neuroimmunomodulation. 2005; 12:321–38.
58. Venkatesh B, Cohen J. The utility of the corticotropin test to diagnose adrenal insufficiency in critical illness: an update. Clin Endocrinol (Oxf). 2015; 83:289–97.
59. Meyer EJ, Nenke MA, Davies ML, Chapman M, Rankin W, Rushworth RL, et al. Corticosteroid-binding globulin deficiency independently predicts mortality in septic shock. J Clin Endocrinol Metab. 2022; 107:1636–46.
60. Peeters B, Langouche L, Van den Berghe G. Adrenocortical stress response during the course of critical illness. Compr Physiol. 2017; 8:283–98.
61. Purnell JQ, Brandon DD, Isabelle LM, Loriaux DL, Samuels MH. Association of 24-hour cortisol production rates, cortisol-binding globulin, and plasma-free cortisol levels with body composition, leptin levels, and aging in adult men and women. J Clin Endocrinol Metab. 2004; 89:281–7.
62. Mah PM, Jenkins RC, Rostami-Hodjegan A, Newell-Price J, Doane A, Ibbotson V, et al. Weight-related dosing, timing and monitoring hydrocortisone replacement therapy in patients with adrenal insufficiency. Clin Endocrinol (Oxf). 2004; 61:367–75.
63. Kehlet H. A rational approach to dosage and preparation of parenteral glucocorticoid substitution therapy during surgical procedures: a short review. Acta Anaesthesiol Scand. 1975; 19:260–4.
64. Lamberts SW, Bruining HA, de Jong FH. Corticosteroid therapy in severe illness. N Engl J Med. 1997; 337:1285–92.
65. Annane D, Renault A, Brun-Buisson C, Megarbane B, Quenot JP, Siami S, et al. Hydrocortisone plus Fludrocortisone for Adults with Septic Shock. N Engl J Med. 2018; 378:809–18.
66. Pastores SM, Annane D, Rochwerg B; Corticosteroid Guideline Task Force of SCCM and ESICM. Guidelines for the diagnosis and management of critical illness-related corticosteroid insufficiency (CIRCI) in critically ill patients (Part II): Society of Critical Care Medicine (SCCM) and European Society of Intensive Care Medicine (ESICM) 2017. Intensive Care Med. 2018; 44:474–7.
67. Culver A, Coiffard B, Antonini F, Duclos G, Hammad E, Vigne C, et al. Circadian disruption of core body temperature in trauma patients: a single-center retrospective observational study. J Intensive Care. 2020; 8:4.
68. Young A, Marsh S. Steroid use in critical care. BJA Educ. 2018; 18:129–34.
69. Yang T, Li Z, Jiang L, Xi X. Corticosteroid use and intensive care unit-acquired weakness: a systematic review and meta-analysis. Crit Care. 2018; 22:187.
70. Yasir M, Goyal A, Sonthalia S. Corticosteroid adverse effects [Internet]. StatPearls Publishing;2023. [cited 2024 Aug 1]. Available from: https://pubmed.ncbi.nlm.nih.gov/30285357/.
71. Ocejo A, Correa R. Methylprednisolone [Internet]. StatPearls Publishing;2022. [cited 2024 Aug 1]. Available from: https://pubmed.ncbi.nlm.nih.gov/31335060/.
Figure 1.
Pathophysiology of critical illness-related corticosteroid insufficiency (CIRCI). (A) Variation in hypothalamic-pituitary-adrenal (HPA) axis response during critical illness. (B) Altered cortisol metabolism, HPA axis inhibition and peripheral resistance to cortisol. (C) Tissue resistance to corticosteroids. (D) Drug-induced CIRCI. GRα: glucocorticoid receptor alpha; GRβ: glucocorticoid receptor beta.
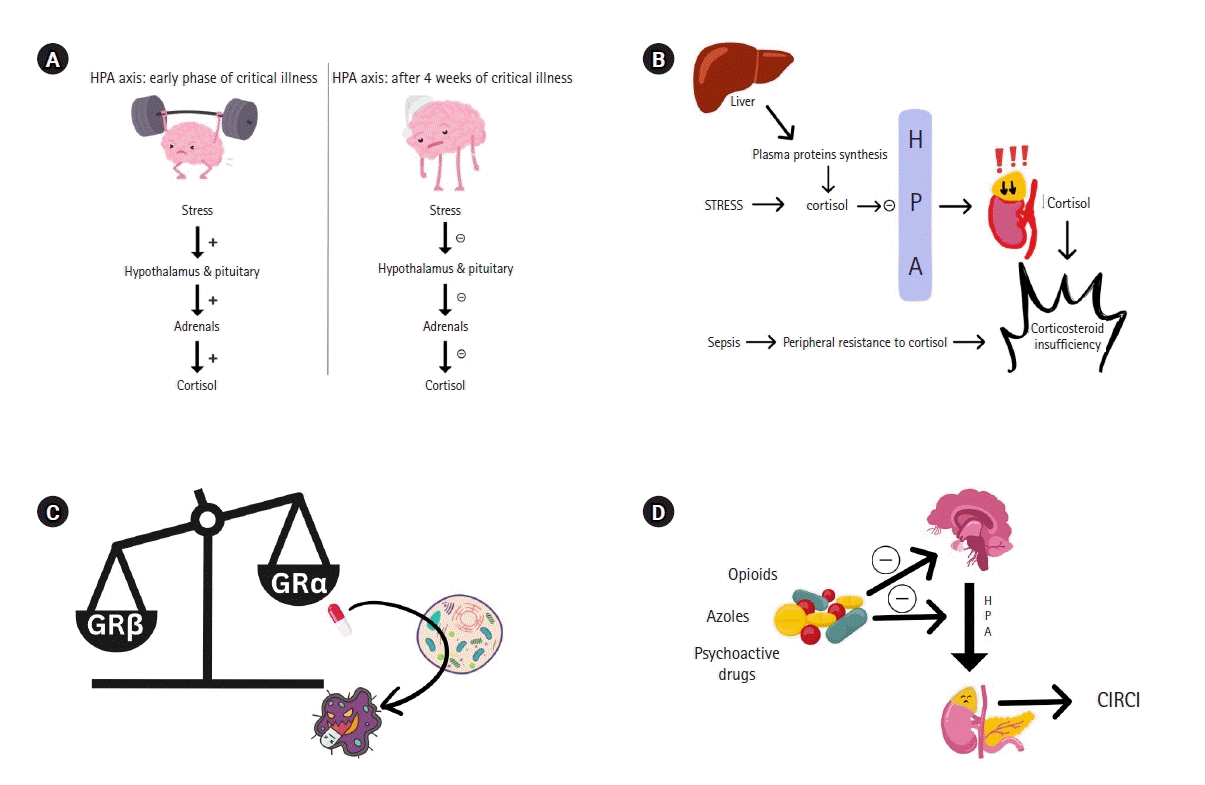
Table 1.
Treatment guidelines for CIRCI: 2017 and beyond
Mechanism of action: hydrocortisone and methylprednisolone bind to the glucocorticoid receptor and blocks the function of transcription factors such as nuclear factor-kappa-B and phospholipase A2 which leads to inhibition of the promoter sites of proinflammatory genes, promotes expression of anti-inflammatory gene products, and inhibits the synthesis of inflammatory cytokines [5,12,31,66,67,70,71].
CIRCI: critical illness-related corticosteroid insufficiency; IV: intravenous; ARDS: acute respiratory distress syndrome;