Abstract
Extracellular vesicles are nano-sized vesicles surrounded by lipid bilayers, and all cells release them to the extracellular environment for communication. Extracellular vesicles consist of molecules with various biological activities and can play essential roles as therapeutics, so they attract much attention as next-generation modalities to treat various diseases. As extracellular vesicles are cell-derived nanovesicles, they are favorable to be developed as therapeutics, but they also have limitations. In addition, there are a number of things to consider in terms of manufacturing, quality control, non-clinical studies, and clinical trials during the development of extracellular vesicle-based therapeutics. Meanwhile, as much attention has been paid to the potentials of extracellular vesicles as therapeutics, many biopharmaceutical companies are trying to develop extracellular vesicle-based therapeutics. This review will introduce the advantages and limitations of extracellular vesicles as therapeutics. In addition, it will cover things to consider during developing extracellular vesicle-based therapeutics and development cases of extracellular vesicle-based therapeutics.
Extracellular vesicles are nano-sized vesicles (20–1,000 nm) surrounded by lipid bilayers, and all cells release extracellular vesicles to the extracellular environment for intercellular communication [12]. Conventionally, soluble factors, such as cytokines, hormones, and neurotransmitters, have been suggested to play crucial roles in intercellular communication. Recently, there has been increasing attention paid to extracellular vesicle-mediated intercellular communication. As extracellular vesicles have been observed to be released by cells of all domains of life (Bacteria, Archaea, and Eukarya), the release of extracellular vesicles is suggested to be an evolutionarily conserved phenomenon [34]. In addition, extracellular vesicles have been identified in various biological fluids, including cell-conditioned media, blood, urine, cerebrospinal fluid, tears, salivary fluid, breast milk, amniotic fluid, seminal fluid, ascites, and pleural effusion [5678].
Extracellular vesicles have been termed various names, but it is desirable to use the term “extracellular vesicles.” The various names referring to extracellular vesicles reflect diverse sizes, biogenesis mechanisms, and origins of extracellular vesicles [9]: exosomes, ectosomes, microvesicles, nanovesicles, membrane vesicles, membrane particles, outer membrane vesicles, oncosomes, dexosomes, and prostasomes. However, all these entities exist in the “extracellular” environment and are “vesicles.” The term “extracellular vesicles” is widely used in the field of extracellular vesicles. For instance, there are the International Society for Extracellular Vesicles (ISEV), the Korean Society for Extracellular Vesicles, and the Extracellular Vesicles Industry Association [1011]. In addition, the Ministry of Food and Drug Safety of Korea (MFDS) provides the guideline regarding extracellular vesicles, namely “Guideline on Quality, Non-clinical and Clinical Assessment of Extracellular Vesicles Therapy Products” [12].
Recently, extracellular vesicles have attracted much attention as next-generation modalities to treat various diseases. Extracellular vesicles consist of proteins, lipids, nucleic acids, and metabolites with various biological activities [1314], and can play diverse roles in tissue regeneration and immune regulation [1516]. The global extracellular vesicle market in therapeutics is expected to grow from 55.05 billion dollars in 2022 to 1.99 trillion dollars in 2030, with compound annual growth rate of 17.4% [17]. There are no commercially available extracellular vesicle-based therapeutics until now, but extracellular vesicles have a high potential to be therapeutics, so many biopharmaceutical companies are trying to develop extracellular vesicle-based therapeutics. This review will cover trends in developing extracellular vesicle-based therapeutics. It will introduce the advantages and limitations of extracellular vesicles as therapeutics. In addition, it will cover things to consider during developing extracellular vesicle-based therapeutics and development cases of extracellular vesicle-based therapeutics.
As extracellular vesicles are nano-sized vesicles derived from cells, they have many potential advantages as therapeutics for various diseases (Fig. 1). Nevertheless, it is necessary to overcome several limitations to develop extracellular vesicles as therapeutics.
Extracellular vesicles are derived from cells, but are not cells themselves, so they can compensate for many problems of cell-based therapeutics. Extracellular vesicles can fundamentally solve the safety issues of cell-based therapeutics. In the case of stem cell-based therapeutics, the cells may proliferate and transform into cancer cells in vivo, and there may be variability in differentiation ability [1819]. However, as extracellular vesicles are not cells, they cannot proliferate or differentiate themselves in vivo, eliminating the problems of stem cell-based therapeutics, and they can be stored stably for a long period of time [20].
Extracellular vesicles can also be used to load and deliver therapeutic drugs. Due to their nano-sized nature, they can deliver drugs specifically to tumor tissues. Because angiogenesis is active in tumor tissues, there are many blood vessels with high permeability, and there is a poor drainage via the lymphatic system [21]. Nanoparticles with a long circulation time in the blood penetrate into tumor tissues with a large amount and are then retained there; this phenomenon is called enhanced permeability and retention effect [22]. Therefore, extracellular vesicles can also utilize this effect to deliver drugs specifically to tumor tissues. Meanwhile, as extracellular vesicles are derived from cells, they are safer than other nanoparticles [23], and the cells can be engineered to produce extracellular vesicles with enhanced targeting [24]. Furthermore, since extracellular vesicles have both a lipid bilayer and lumen, they can be used to deliver therapeutic drugs to target cells or tissues by loading the drugs into the lipid bilayer or lumen [25].
Although extracellular vesicles have many advantages, there are several limitations in developing them as therapeutics. Cells secrete a limited amount of extracellular vesicles, but a sufficient amount of extracellular vesicles must be produced to develop the vesicles as therapeutics [26]. This is because a large amount of extracellular vesicles is required for conducting non-clinical studies and clinical trials during the development processes. In addition, if the productivity were not sufficiently high, the vesicles would have no market value. Furthermore, cells release various types of extracellular vesicles, and the vesicles consist of diverse molecules [5], but extracellular vesicles must be produced with consistency and equivalence to be developed as therapeutics. Especially, since extracellular vesicles can have different characteristics depending on the status of parental cells [27], characterization indices for each production batch must be managed to ensure consistency and equivalence within a certain range.
Several strategies have been employed to overcome these limitations. A large amount of extracellular vesicles can be produced by various approaches [28]. Mammalian cells can be grown in a high density in three-dimensional bioreactors [29]. The productivity can be improved by engineering parental cells [30], or by stimulating parental cells by shear stress [31]. In addition, non-human sources, such as milk and bacteria, are cost-effective for mass production of extracellular vesicles [28]. Furthermore, extracellular vesicle mimetics can be generated by several methods including serial extrusion, with a 100-fold higher yield than naturally released extracellular vesicles [32]. On the other hand, extracellular vesicles can be manufactured with consistency and equivalence by considering several items in terms of manufacturing and quality control. These items will be discussed in the following section, along with the items regarding non-clinical studies and clinical trials.
To develop extracellular vesicle-based therapeutics, there are many things to consider in terms of manufacturing, quality control, non-clinical studies, and clinical trials. Extracellular vesicles are biological products and can be currently classified as “other agents recognized by the Minister of MFDS” [1233]. MFDS established “Guideline on Quality, Non-clinical and Clinical Assessment of Extracellular Vesicles Therapy Products” for the first time in the world in 2018 [34], and amended it in 2023 [12]. MFDS also published “Information Collection for Quality and Non-clinical Assessment of Stem Cell-Derived Extracellular Vesicles Therapy Products” in 2021 [35], providing the guidelines for developing extracellular vesicles as therapeutics.
When developing extracellular vesicle-based therapeutics, there are things to consider in terms of manufacturing. Since cells or tissues are used as starting materials for manufacturing extracellular vesicles, it is necessary to analyze and record characteristics of the cells or tissues used for manufacturing extracellular vesicles [12]. When using immortalized clonal cell lines, the stability of genotypes and phenotypes should be rigorously assessed during extracellular vesicle manufacturing. Meanwhile, when collecting cells or tissues from donors, it is necessary to record collection procedures, donor eligibility criteria, health status, and medical history. Donor eligibility criteria follow “Guideline on Eligibility Determination for Donors of Cell Therapy Products,” and the blood of donors is collected and assessed from 7 days before to 7 days after collecting cells or tissues for producing extracellular vesicles [36]. Main study items include hepatitis B virus, hepatitis C virus, human immunodeficiency virus type 1/2, and syphilis. Depending on the cells or tissues, additional study items may include human T-lymphotropic virus, cytomegalovirus, chlamydia, and gonorrhea. All study results must be negative to pass the eligibility criteria. In addition, to consistently manage the quality of extracellular vesicle-based therapeutics, it is necessary to establish a cell bank and characterize the bank by referring to “Guideline for Cell Bank Evaluation of Cell Therapy Products,” except for autologous cells [37]. Test items to characterize the bank include cell identity (e.g., karyotyping, cell surface phenotype markers, and expression levels of certain genes), stability (e.g., morphology, growth characteristics), genetic stability (e.g., karyotyping), and adventitious agents (e.g., viruses, bacteria, fungi, mycoplasma, and transmissible spongiform encephalopathy factors).
Cell culture must be standardized for producing extracellular vesicles, as extracellular vesicles are mainly manufactured from cell culture conditioned media [12]. During the manufacturing process, it is necessary to standardize cell density, cell viability, cell morphology, subculture method, doubling time, oxygen/carbon dioxide concentrations, incubation temperature, media additives, media composition, and culture vessels, to demonstrate reproducibility among batches. In addition, it is necessary to record the origin of all reagents utilized in manufacturing extracellular vesicles, and it is recommended to use reagents that do not contain animal-derived components (e.g., fetal bovine serum), or recombinant proteins whenever possible.
Isolation and purification of extracellular vesicles must also be standardized, since the characteristics of extracellular vesicles vary depending on isolation and purification methods. Several methods can be used alone or combined to isolate and purify extracellular vesicles: ultracentrifugation, ultrafiltration, precipitation, and chromatography [38]. To demonstrate reproducibility of the production process, the isolation and purification method should be described in detail, and the extracellular vesicles manufactured by the process should be characterized in several aspects. It is necessary to analyze protein, RNA, or lipid profiles of extracellular vesicles using state-of-the-art technologies (e.g., LC-MS/MS [liquid chromatography-mass spectrometry/mass spectrometry]), and compare protein profiles with the previously identified vesicular proteins using extracellular vesicle databases (e.g., EVpedia [2]). Then, electron microscopy and/or nanoparticle tracking analysis should be employed to analyze lipid bilayered structures and size distribution of extracellular vesicles. In addition, it is required to quantitatively analyze dose-dependency in functional activity of extracellular vesicles with control groups, and to establish qualitative/quantitative test methods and allowable limits of impurities. The characterization data of extracellular vesicles are also important in proving comparative equivalence when altering the manufacturing process.
To develop extracellular vesicle-based therapeutics, it is also necessary to control the quality of extracellular vesicles after manufacturing the vesicles. Study items to control the quality of extracellular vesicles include appearance, number, and size of extracellular vesicles, as well as negative tests for contaminants, identity, purity, potency, and stability [1239]. Appearance is to visually inspect whether the basic appearance of the product is eligible, and depending on the formulation, it may include the color and shape of filling containers and drug substance/product. The number of extracellular vesicles is the index related to the content of extracellular vesicles, and can be determined by nanoparticle tracking analysis, resistive pulse sensing, and flow cytometry. To distinguish extracellular vesicles from other particles with similar size, it is necessary to quantify other indices such as protein amounts. The size of extracellular vesicles shows a distribution pattern, and can be measured using nanoparticle tracking analysis, dynamic light scattering, resistive pulse sensing, and flow cytometry.
During manufacturing, isolation, and purification processes, extracellular vesicles can be contaminated with mycoplasma, adventitious viruses, bacteria, fungi, and endotoxin from animal-derived materials and reagents or from facilities and environment [1240]. These contaminants can affect the quality of extracellular vesicles and patient safety upon clinical application. Therefore, it is necessary to set management standards for contaminants, and to demonstrate that the quality of extracellular vesicles is consistently controlled within these standards.
The identity test is to confirm whether extracellular vesicles are the major ingredients in drug substance/product of extracellular vesicle-based therapeutics. When conducting the identity test, it is necessary to select and measure several proteins, RNAs, or lipids, which are known to be enriched in extracellular vesicles. The purity test is to determine the purity of extracellular vesicles by measuring cellular proteins, which are known not to be enriched in extracellular vesicles and process impurities. The potency test is performed through biological assays, which can predict the functional activity of extracellular vesicles, and must reflect the expected mechanism of action of extracellular vesicles. In vitro tests should quantitatively analyze the dose-response relationship, and in vivo tests should describe the reason why the animal species was selected appropriately. Meanwhile, the stability of extracellular vesicles is assessed according to “Standard for Stability Study of Pharmaceuticals (MFDS Notice)” [41]. Stability studies can be divided into long-term testing, stress testing, and accelerated testing.
Non-clinical and clinical evaluations are also essential for developing extracellular vesicles as therapeutics, and there are things to consider during these processes. Non-clinical evaluation of extracellular vesicle-based therapeutics follows the basic principles of non-clinical evaluation of biopharmaceuticals, and requires data on pharmacological action and toxicity [12]. Data on pharmacological action include studies on efficacy, safety pharmacology, and adsorption/distribution/metabolism/excretion. Efficacy study requires dose escalation studies in animals appropriate to the intended use, and it is recommended to conduct comparative studies with proper controls. Safety pharmacology study is to determine whether extracellular vesicles have undesirable pharmacodynamic characteristics related to patient safety. Studies on adsorption/distribution/metabolism/excretion analyze pharmacodynamic characteristics of extracellular vesicles, such as adsorption/distribution/metabolism/excretion, including blood concentration and tissue distribution. The studies can be conducted by fluorescent labeling, immunohistochemistry, polymerase chain reaction, and radioisotope labeling. Additional data may be required to validate that biological activities of extracellular vesicles were not affected by labeling or genetic modifications used in the studies. In addition, the group size must be sufficiently large and appropriate control groups must be included in animal experiments.
Data on toxicity include studies on single/repeated dose toxicity, genotoxicity, tumorigenicity/carcinogenicity, and immunogenicity, and can be performed according to “Standard for Toxicity Study of Pharmaceuticals (MFDS Notice)” [42]. Single/repeated dose toxicity studies are to examine toxicity, which appears in the short/long term by single/repeated administration in animals appropriate to the intended use. In the case of extracellular vesicles, this should be done through a dose escalation study. Genotoxicity study is to assess damaging effects on genes or chromosomes. Tumorigenicity/carcinogenicity study is to determine whether cancer is induced by long-term administration in animals appropriate to the intended use. In the case of extracellular vesicles, it is not generally required, but may be required depending on the type of parental cells and introduced genes. Immunogenicity study is to examine abnormal immune responses when there are abnormalities in the immune system during repeated toxicity study. In the case of extracellular vesicles, the study is generally required because extracellular vesicles may induce immunogenicity. Meanwhile, as extracellular vesicles are free of cells, clinical trials can be conducted using the same criteria and methods as the clinical trials of existing pharmaceuticals.
There are currently no commercially available extracellular vesicle-based therapeutics in the world. However, there is a commercialized medicine based on extracellular vesicles, namely BEXSERO®, a vaccine against Neisseria meningitidis serogroup B, developed by Novartis in Switzerland and sold by GSK in the UK. BEXSERO® is composed of extracellular vesicles (outer membrane vesicles) derived from N. meningitidis serogroup B and three types of recombinant proteins [43]. Since its commercialization in 2013, it has recorded sales of 849 million pounds worldwide in 2023 [44]. Following the success of BEXSERO®, many biopharmaceutical companies continuously make efforts to develop extracellular vesicles as therapeutics, but the development of extracellular vesicle-based therapeutics is generally in non-clinical and early clinical phases.
As extracellular vesicles gain attention due to their potentials as therapeutics, many global biopharmaceutical companies are developing extracellular vesicle-based therapeutics (Table 1). Codiak Biosciences (USA) was the leader in developing extracellular vesicle-based therapeutics. Codiak Biosciences developed exoSTING™ (CDK-002), based on extracellular vesicles loaded with stimulator of interferon gene (STING) agonists, to treat solid tumors, and completed phase I/II clinical trials [45]. In the phase I/II clinical trials, exoSTING™ was well tolerated with repeated doses, and it was retained in tumor tissues with no systemic exposure of STING agonists [46]. In some patients, tumor regression was observed in both injected and non-injected lesions. In addition, exoIL-12™ (CDK-003) was devised to treat cutaneous T cell lymphoma, based on extracellular vesicles loaded with IL-12 [47]. In the phase I clinical trial, exoIL-12™ was shown to be safe and tolerable, with no systemic exposure of IL-12 and no treatment-related adverse events [46]. Two patients were treated with multiple injections of exoIL-12™, and tumor regression was observed in both injected and non-injected lesions. Furthermore, exoASO™-STAT6 (CDK-004) was developed to treat hepatocellular carcinoma, based on extracellular vesicles loaded with anti-sense oligonucleotides (ASO) against signal transducer and activator of transcription 6 (STAT6) [48]. Based on these results, Codiak Biosciences signed a contract, worth up to 1 billion dollars, with Jazz Pharmaceuticals in January 2019, to develop and commercialize extracellular vesicle-based therapeutics [49]. However, Codiak Biosciences went bankrupt in March 2023, due to financial difficulties while conducting clinical trials for several pipelines [50]. Codiak Biosciences went bankrupt due to its excessively high cost structure, so it is necessary to reduce or cover the cost for developing extracellular vesicle-based therapeutics. In order to reduce the cost, it is necessary to prioritize pipelines through choice and concentration, and to mass-produce extracellular vesicles at low cost. In addition, it is important to establish a business model, which generates real sales to cover the costs, and appropriate technology transfer or partnering is needed to enable clinical trials and global marketing.
Evox Therapeutics (UK) focuses on extracellular vesicle-mediated gene therapy, employing its own platform called DeliverEX®. Evox Therapeutics will collaborate with the Icahn School of Medicine at Mount Sinai (USA) to encapsulate adeno-associated virus (AAV) into extracellular vesicles, and to develop extracellular vesicle-encapsulated AAV vectors (exoAAV), a gene therapy technology to treat cardiac diseases [51]. Conventional AAV has drawn attention as a method to deliver genes to the heart due to its cardiotropism, long-term expression, and safety, but its therapeutic efficacy was reduced due to neutralizing antibodies [52]. exoAAV is a technology that aims to overcome the limitations of AAV by loading AAV into extracellular vesicles. In addition, Evox Therapeutics acquired engEx-AAV™ technology platform and related intellectual property rights, an AAV-related technology owned by Codiak Biosciences, in June 2023, thereby securing exclusive access to the related technology [53].
Aegle Therapeutics (USA) is conducting research to treat various diseases using mesenchymal stem cell-derived extracellular vesicles. Based on COL7A1, a gene involved in type VII collagen, Aegle Therapeutics developed AGLE-102™, which harbors COL7A1 mRNA/protein in extracellular vesicles [54]. Using AGLE-102™, Aegle Therapeutics is conducting a phase I/II clinical trial to treat a rare disease called dystrophic epidermolysis bullosa [55]. In addition, a phase I clinical trial is undergoing to confirm the potential of AGLE-102™ to treat burn wounds [56]. The first patient with severe second-degree burns was treated with a single topical dose of AGLE-102™ [57]. The patient showed 99% epithelialization of the burn wound within 7 days after the treatment. There was no evidence of ischemia reperfusion injury and burn wound conversion.
Direct Biologics (USA) is conducting research to treat various inflammatory diseases using extracellular vesicles derived from bone marrow-derived mesenchymal stem cells. Direct Biologics developed ExoFlo™, a mesenchymal stem cell-derived extracellular vesicle-based therapeutics [58]. Direct Biologics completed a phase II clinical trial to treat acute respiratory distress syndrome associated with coronavirus disease-19 [59]. ExoFlo™ was shown to reduce 60-day mortality in patients with severe coronavirus disease-19-associated respiratory distress syndrome, and there were no reports regarding treatment-related adverse events [60]. In addition, phase I clinical trials are undergoing to treat inflammatory bowel diseases, including Crohn’s disease and ulcerative colitis, using ExoFlo™ [6162].
Korean companies related to extracellular vesicles are also conducting a number of studies to develop extracellular vesicle-based therapeutics (Table 2). ILIAS Biologics developed exosome engineering for protein loading via optically reversible protein-protein interactions (EXPLOR®), a technology that loads desired proteins into extracellular vesicles by controlling reversible protein-protein interactions using blue light [63]. ILIAS Biologics developed Exo-srIκB (ILB-202), extracellular vesicle-based therapeutics loaded with super-repressor IκB, to treat inflammatory diseases, utilizing EXPLOR® technology [64]. ILB-202 has been shown to alleviate acute kidney injury induced by ischemia-reperfusion injury in animal models [65]. In addition, ILB-202 is currently a candidate therapeutics to treat cardiac surgery-associated acute kidney injury, and has completed a phase I clinical trial approved by Human Research Ethics Committee in Australia [66]. After injecting ILB-202 as a single intravenous injection to a total of 18 healthy adult volunteers, there were no serious adverse events across three different cohorts with low, medium, or high doses.
Brexogen has BG-Platform technology, a platform technology based on mesenchymal stem cell-derived extracellular vesicles [67]. BG-Platform technology consists of technology for constructing mesenchymal stem cell lines optimized for producing extracellular vesicles, technology for loading substances into extracellular vesicles, and technology for mass production of extracellular vesicles. Based on BG-Platform technology, Brexogen developed BxC-I17e (BRE-AD01), an atopic dermatitis therapeutics based on extracellular vesicles derived from IFN-γ-treated mesenchymal stem cells, which are differentiated from induced pluripotent stem cells [68]. BRE-AD01 has been shown to alleviate the symptoms of atopic dermatitis in animal models. In addition, BRE-AD01 is currently approved for phase I clinical trials by the United States Food and Drug Administration as a candidate therapeutics for treating atopic dermatitis [69].
MDimune has BioDrone™ platform based on cell-derived nanovesicles (CDVs), which are extracellular vesicle mimetics obtained by cell extrusion, as well as manufacturing and quality control processes for biopharmaceuticals [70]. CDVs have a production yield tens of times higher than that of naturally secreted extracellular vesicles. CDVs can be loaded with various pharmaceuticals including mRNAs, siRNAs, proteins, and synthetic drugs to deliver them to target tissues. Therefore, CDVs gain attraction as highly scalable drug delivery vehicles. Based on BioDrone™ platform, MDimune signed a technology transfer agreement with CaraVan Biologix (United States) in September 2022 for developing chimeric antigen receptor-natural killer cell-based anti-cancer drugs [71]. MDimune also won the Bronze Award in Health, Medical & Biotech section at the 2023 Edison Awards in April 2023 [72].
SL Bigen has two core technologies related to extracellular vesicles. First, it has ghost nanovesicle (GNV) platform. GNVs are extracellular vesicle mimetics with empty lumen, and are produced from cellular membrane obtained by treating nucleated cells with an alkaline solution [73]. GNVs have a production yield tens of times higher than that of naturally secreted extracellular vesicles. As GNVs are manufactured with the cellular membrane opened and the lumen emptied, more various drugs can be loaded with improved safety. It has been demonstrated that GNVs loaded with dexamethasone, an anti-inflammatory substance, alleviated the symptoms of systemic inflammatory response syndrome more effectively than when the same amount of dexamethasone was administered alone, in animal models of systemic inflammatory response syndrome [74]. In addition, SL Bigen developed REX101, a cancer immunotherapy candidate based on bacterial extracellular vesicles [75]. REX101 showed anti-tumor activity when administered alone or in combination with immune checkpoint inhibitors in animal models [76]. SL Bigen has secured the safety of REX101 through non-clinical studies in compliance with good laboratory practice, and completed production of REX101 in compliance with good manufacturing practice to prepare clinical trials as veterinary therapeutics [75].
Currently, extracellular vesicles attract much attention as next-generation therapeutics to treat various diseases. Especially, extracellular vesicles can overcome the limitations of cell-based therapeutics and other nanoparticles, as they are nano-sized vesicles derived from cells. However, in order to develop extracellular vesicle-based therapeutics, it must be possible to produce sufficient quantities, and manage characterization indices to be consistent and equivalent in each production batch. In addition, various issues must be considered in terms of production, quality control, non-clinical studies, and clinical trials. The development of extracellular vesicle-based therapeutics is generally in non-clinical and early clinical phases, but various biopharmaceutical companies continuously make efforts. It is expected that the quality of human life can be improved through the development of therapeutics using extracellular vesicles.
Acknowledgments
I thank Ho-Shin Gwak in the National Cancer Center for inviting us for the review. This review article used a manuscript published in the Biological Research Information Center (BRIC) after re-editing (BRIC VIEW 2024-T08).
Availability of Data and Material
Data sharing is not applicable to this article as no datasets were generated or analyzed during the study.
References
1. Théry C, Ostrowski M, Segura E. Membrane vesicles as conveyors of immune responses. Nat Rev Immunol. 2009; 9:581–593. PMID: 19498381.
2. Kim DK, Lee J, Kim SR, Choi DS, Yoon YJ, Kim JH, et al. EVpedia: a community web portal for extracellular vesicles research. Bioinformatics. 2015; 31:933–939. PMID: 25388151.
3. Kim JH, Lee J, Park J, Gho YS. Gram-negative and Gram-positive bacterial extracellular vesicles. Semin Cell Dev Biol. 2015; 40:97–104. PMID: 25704309.
4. Gill S, Catchpole R, Forterre P. Extracellular membrane vesicles in the three domains of life and beyond. FEMS Microbiol Rev. 2019; 43:273–303. PMID: 30476045.
5. Gho YS, Lee C. Emergent properties of extracellular vesicles: a holistic approach to decode the complexity of intercellular communication networks. Mol Biosyst. 2017; 13:1291–1296. PMID: 28488707.
6. Hirschberg Y, Valle-Tamayo N, Dols-Icardo O, Engelborghs S, Buelens B, Vandenbroucke RE, et al. Proteomic comparison between non-purified cerebrospinal fluid and cerebrospinal fluid-derived extracellular vesicles from patients with Alzheimer’s, Parkinson’s and Lewy body dementia. J Extracell Vesicles. 2023; 12:e12383. PMID: 38082559.
7. Cross T, Øvstebø R, Brusletto BS, Trøseid AS, Olstad OK, Aspelin T, et al. RNA profiles of tear fluid extracellular vesicles in patients with dry eye-related symptoms. Int J Mol Sci. 2023; 24:15390. PMID: 37895069.
8. Park JO, Choi DY, Choi DS, Kim HJ, Kang JW, Jung JH, et al. Identification and characterization of proteins isolated from microvesicles derived from human lung cancer pleural effusions. Proteomics. 2013; 13:2125–2134. PMID: 23585444.
9. Choi DS, Kim DK, Kim YK, Gho YS. Proteomics of extracellular vesicles: exosomes and ectosomes. Mass Spectrom Rev. 2015; 34:474–490. PMID: 24421117.
10. Lötvall J, Rajendran L, Gho YS, Thery C, Wauben M, Raposo G, et al. The launch of Journal of Extracellular Vesicles (JEV), the official journal of the International Society for Extracellular Vesicles – about microvesicles, exosomes, ectosomes and other extracellular vesicles. J Extracell Vesicles. 2012; 1:18514.
11. Korean Society for Extracellular Vesicles: Main page. Seoul: Korean Society for Extracellular Vesicles;Accessed April 24, 2024. at https://ksev.or.kr.
12. National Institute of Food and Drug Safety Evaluation, Ministry of Food and Drug Safety. Guideline on quality, non-clinical and clinical assessment of extracellular vesicles therapy products (guideline-0917-02) (partially amended on December 21, 2023). Available at: https://www.mfds.go.kr/brd/m_1060/down.do?brd_id=data0011&seq=15422&data_tp=A&file_seq=3.
13. Choi DS, Kim DK, Kim YK, Gho YS. Proteomics, transcriptomics and lipidomics of exosomes and ectosomes. Proteomics. 2013; 13:1554–1571. PMID: 23401200.
14. Mayr M, Grainger D, Mayr U, Leroyer AS, Leseche G, Sidibe A, et al. Proteomics, metabolomics, and immunomics on microparticles derived from human atherosclerotic plaques. Circ Cardiovasc Genet. 2009; 2:379–388. PMID: 20031610.
15. Murphy DE, de Jong OG, Brouwer M, Wood MJ, Lavieu G, Schiffelers RM, et al. Extracellular vesicle-based therapeutics: natural versus engineered targeting and trafficking. Exp Mol Med. 2019; 51:1–12.
16. Robbins PD, Morelli AE. Regulation of immune responses by extracellular vesicles. Nat Rev Immunol. 2014; 14:195–208. PMID: 24566916.
17. Global exosome therapeutics market – industry trends and forecast to 2030. Pune: Data Bridge Market Research;2023. Accessed April 24, 2024. at https://www.databridgemarketresearch.com/reports/global-exosome-therapeutic-market.
18. Ben-David U, Arad G, Weissbein U, Mandefro B, Maimon A, Golan-Lev T, et al. Aneuploidy induces profound changes in gene expression, proliferation and tumorigenicity of human pluripotent stem cells. Nat Commun. 2014; 5:4825. PMID: 25198699.
19. Buganim Y, Markoulaki S, van Wietmarschen N, Hoke H, Wu T, Ganz K, et al. The developmental potential of iPSCs is greatly influenced by reprogramming factor selection. Cell Stem Cell. 2014; 15:295–309. PMID: 25192464.
20. Zhang K, Cheng K. Stem cell-derived exosome versus stem cell therapy. Nat Rev Bioeng. 2023; 1:608–609.
21. Azzi S, Hebda JK, Gavard J. Vascular permeability and drug delivery in cancers. Front Oncol. 2013; 3:211. PMID: 23967403.
22. Shi J, Kantoff PW, Wooster R, Farokhzad OC. Cancer nanomedicine: progress, challenges and opportunities. Nat Rev Cancer. 2017; 17:20–37. PMID: 27834398.
23. Cecchin R, Troyer Z, Witwer K, Morris KV. Extracellular vesicles: the next generation in gene therapy delivery. Mol Ther. 2023; 31:1225–1230. PMID: 36698310.
24. Song H, Chen X, Hao Y, Wang J, Xie Q, Wang X. Nanoengineering facilitating the target mission: targeted extracellular vesicles delivery systems design. J Nanobiotechnology. 2022; 20:431. PMID: 36175866.
25. Fu S, Wang Y, Xia X, Zheng JC. Exosome engineering: current progress in cargo loading and targeted delivery. NanoImpact. 2020; 20:100261.
26. Grangier A, Branchu J, Volatron J, Piffoux M, Gazeau F, Wilhelm C, et al. Technological advances towards extracellular vesicles mass production. Adv Drug Deliv Rev. 2021; 176:113843. PMID: 34147532.
27. Zhang Y, Liu Y, Liu H, Tang WH. Exosomes: biogenesis, biologic function and clinical potential. Cell Biosci. 2019; 9:19. PMID: 30815248.
28. Paolini L, Monguió-Tortajada M, Costa M, Antenucci F, Barilani M, Clos-Sansalvador M, et al. Large-scale production of extracellular vesicles: report on the “massivEVs” ISEV workshop. J Extracell Biol. 2022; 1:e63. PMID: 38939213.
29. Bellani CF, Ajeian J, Duffy L, Miotto M, Groenewegen L, Connon CJ. Scale-up technologies for the manufacture of adherent cells. Front Nutr. 2020; 7:575146. PMID: 33251241.
30. Chen TS, Arslan F, Yin Y, Tan SS, Lai RC, Choo AB, et al. Enabling a robust scalable manufacturing process for therapeutic exosomes through oncogenic immortalization of human ESC-derived MSCs. J Transl Med. 2011; 9:47. PMID: 21513579.
31. Patel DB, Santoro M, Born LJ, Fisher JP, Jay SM. Towards rationally designed biomanufacturing of therapeutic extracellular vesicles: impact of the bioproduction microenvironment. Biotechnol Adv. 2018; 36:2051–2059. PMID: 30218694.
32. Jang SC, Kim OY, Yoon CM, Choi DS, Roh TY, Park J, et al. Bioinspired exosome-mimetic nanovesicles for targeted delivery of chemotherapeutics to malignant tumors. ACS Nano. 2013; 7:7698–7710. PMID: 24004438.
33. Ministry of Food and Drug Safety. Regulation on approval and review of biological products (notice no. 2024-31) (partially amended and enforced on June 25, 2024). Available at: https://www.mfds.go.kr/brd/m_207/down.do?brd_id=data0008&seq=14984&data_tp=A&file_seq=2.
34. National Institute of Food and Drug Safety Evaluation, Ministry of Food and Drug Safety. Guideline on quality, non-clinical and clinical assessment of extracellular vesicles therapy products (guideline-0917-01) (established on December 27, 2018). Available at: https://www.nifds.go.kr/brd/m_15/view.do?seq=12625.
35. National Institute of Food and Drug Safety Evaluation, Ministry of Food and Drug Safety. [Information collection for quality and non-clinical assessment of stem cell-derived extracellular vesicles therapy products]. Seoul: Jinhan M&B;2022. Korean.
36. National Institute of Food and Drug Safety Evaluation, Ministry of Food and Drug Safety. Guideline on eligibility determination for donors of cell therapy products (guideline 0311-02) (partially amended on June 7, 2021). Available at: https://www.mfds.go.kr/brd/m_1060/view.do?seq=14860&srchFr=&srchTo=&srchWord=&srchTp=&itm_seq_1=0&itm_seq_2=0&multi_itm_seq=0&company_cd=&company_nm=&page=90.
37. National Institute of Food and Drug Safety Evaluation, Ministry of Food and Drug Safety. Guideline for cell bank evaluation of cell therapy products (guideline 1141-02) (partially amended on October 31, 2023). Available at: https://www.mfds.go.kr/brd/m_1060/view.do?seq=15379.
38. Chen J, Li P, Zhang T, Xu Z, Huang X, Wang R, et al. Review on strategies and technologies for exosome isolation and purification. Front Bioeng Biotechnol. 2022; 9:811971. PMID: 35071216.
39. Lener T, Gimona M, Aigner L, Börger V, Buzas E, Camussi G, et al. Applying extracellular vesicles based therapeutics in clinical trials - an ISEV position paper. J Extracell Vesicles. 2015; 4:30087. PMID: 26725829.
40. Quah BJ, O’Neill HC. Mycoplasma contaminants present in exosome preparations induce polyclonal B cell responses. J Leukoc Biol. 2007; 82:1070–1082. PMID: 17698916.
41. Ministry of Food and Drug Safety. Standard for stability study of pharmaceuticals (notice 2019-132) (partially amended and enforced on December 17, 2019). Available at: https://www.mfds.go.kr/brd/m_211/view.do?seq=14421.
42. Ministry of Food and Drug Safety. Standard for toxicity study of pharmaceuticals (notice no. 2022-18) (partially amended and enforced on March 2, 2022). Available at: https://www.law.go.kr/LSW/admRulLsInfoP.do?chrClsCd=&admRulSeq=2100000209652.
43. BEXSERO (Meningococcal group B vaccine) suspension, for intramuscular injection. Durham: GSK;2023. Accessed April 24, 2024. at https://gskpro.com/content/dam/global/hcpportal/en_US/Prescribing_Information/Bexsero/pdf/BEXSERO.PDF.
44. GSK delivers strong 2023 performance and upgrades growth outlooks. Brentford: GSK;2024. Accessed April 24, 2024. at https://www.gsk.com/media/10928/fy-2023-results-announcement.pdf.
45. A first-in-human study of CDK-002 (exoSTING) in subjects with advanced/metastatic, recurrent, injectable solid tumors. Cambridge: Codiak BioSciences;Accessed April 24, 2024. at https://clinicaltrials.gov/study/NCT04592484.
46. Codiak announces platform-validating clinical data from phase 1 trials of ExoSTING and ExoIL-12. New York: Markets Insider;2022. Accessed April 24, 2024. at https://markets.businessinsider.com/news/stocks/codiak-announces-platform-validating-clinical-data-from-phase-1-trials-of-exosting-and-exoil-12-1031559977.
47. A phase 1/2a study of CDK-003 in patients with cutaneous T-cell lymphoma (CTCL). Bethesda: U.S. National Library of Medicine;2022. Accessed April 24, 2024. at https://clinicaltrials.gov/study/NCT05156229.
48. A study of exoASO-STAT6 (CDK-004) in patients with advanced hepatocellular carcinoma (HCC) and patients with liver metastases from either primary gastric cancer or colorectal cancer (CRC). Bethesda: U.S. National Library of Medicine;2023. Accessed April 24, 2024. at https://clinicaltrials.gov/study/NCT05375604.
49. Jazz Pharmaceuticals and Codiak BioSciences announce strategic collaboration to research, develop and commercialize engineered exosomes to create therapies for hard-to-treat cancers. Dublin: Jazz Pharmaceuticals;2019. Accessed April 24, 2024. at https://investor.jazzpharma.com/news-releases/news-release-details/jazz-pharmaceuticals-and-codiak-biosciences-announce-strategic-0.
50. Codiak files for bankruptcy after exosome-focused biotech unable to satisfy ‘financial needs’. New York: Fierce Biotech;2023. Accessed April 24, 2024. at https://www.fiercebiotech.com/biotech/codiak-biosciences-files-bankruptcy-after-unable-satisfy-financial-needs.
51. Evox Therapeutics enters into agreement to advance next generation exosome-delivered AAV gene therapy for the treatment of heart disease. Oxford: Evox Therapeutics;2023. Accessed April 24, 2024. at https://www.evoxtherapeutics.com/evox-therapeutics-enters-into-agreement-to-advance-next-generation-exosome-delivered-aav-gene-therapy-for-the-treatment-of-heart-disease.
52. Li X, La Salvia S, Liang Y, Adamiak M, Kohlbrenner E, Jeong D, et al. Extracellular vesicle-encapsulated adeno-associated viruses for therapeutic gene delivery to the heart. Circulation. 2023; 148:405–425. PMID: 37409482.
53. Evox Therapeutics acquires exosome AAV technology and intellectual property. Oxford: Evox Therapeutics;2023. Accessed April 24, 2024. at https://www.evoxtherapeutics.com/evox-therapeutics-acquires-exosome-aav-technology-and-intellectual-property.
54. Pipeline. Woburn: AEGLE Therapeutics. Accessed April 24, 2024. at https://aegletherapeutics.com/pipeline.
55. MSC EVs in dystrophic epidermolysis bullosa. Bethesda: U.S. National Library of Medicine;2024. Accessed April 24, 2024. at https://clinicaltrials.gov/study/NCT04173650.
56. Safety of extracellular vesicles for burn wounds. Bethesda: U.S. National Library of Medicine;2023. updated Mar 29, 2024. Accessed April 24, 2024. at https://clinicaltrials.gov/study/NCT05078385.
57. AEGLE Therapeutics Corp. announces positive data for the first patient in a phase 1/2a clinical trial dosed with AGLE-102™, a novel extracellular vesicle therapy. Woburn: AEGLE Therapeutics;2024. Accessed April 24, 2024. at https://www.prnewswire.com/news-releases/aegle-therapeutics-corp-announces-positive-data-for-the-first-patient-in-a-phase-12a-clinical-trial-dosed-with-agle-102-a-novel-extracellular-vesicle-therapy-302027568.html.
58. ExoFlo™. Austin: Direct Biologics;Accessed April 24, 2024. at https://directbiologics.com/exoflo.
59. Extracellular vesicle infusion treatment for COVID-19 associated ARDS (EXIT-COVID19). Bethesda: U.S. National Library of Medicine;2020. updated Feb 13, 2024. Accessed April 24, 2024. at https://clinicaltrials.gov/study/NCT04493242.
60. Lightner AL, Sengupta V, Qian S, Ransom JT, Suzuki S, Park DJ, et al. Bone marrow mesenchymal stem cell-derived extracellular vesicle infusion for the treatment of respiratory failure from COVID-19: a randomized, placebo-controlled dosing clinical trial. Chest. 2023; 164:1444–1453. PMID: 37356708.
61. Study of ExoFlo for the treatment of medically refractory Crohn’s disease. Bethesda: U.S. National Library of Medicine;2023. updated Feb 12, 2024. Accessed April 24, 2024. at https://clinicaltrials.gov/study/NCT05130983.
62. Study of ExoFlo for the treatment of medically refractory ulcerative colitis. Bethesda: U.S. National Library of Medicine;2022. updated Feb 12, 2024. Accessed April 24, 2024. at https://clinicaltrials.gov/study/NCT05176366.
63. Yim N, Ryu SW, Choi K, Lee KR, Lee S, Choi H, et al. Exosome engineering for efficient intracellular delivery of soluble proteins using optically reversible protein-protein interaction module. Nat Commun. 2016; 7:12277. PMID: 27447450.
64. Choi H, Kim Y, Mirzaaghasi A, Heo J, Kim YN, Shin JH, et al. Exosome-based delivery of super-repressor IκBα relieves sepsis-associated organ damage and mortality. Sci Adv. 2020; 6:eaaz6980. PMID: 32285005.
65. Kim S, Lee SA, Yoon H, Kim MY, Yoo JK, Ahn SH, et al. Exosome-based delivery of super-repressor IκBα ameliorates kidney ischemia-reperfusion injury. Kidney Int. 2021; 100:570–584. PMID: 34051264.
66. Ililas biologics and Brexogen’s exosome-based drug development trials. Seoul: HitNews;2023. Accessed April 24, 2024. at http://www.hitnews.co.kr/news/articleView.html?idxno=49243.
67. BREXOGEN. Platform - BG Platform. Seoul: BREXOGEN;Accessed April 24, 2024. at http://brexogen.com/m21.php.
68. Kim J, Lee SK, Jung M, Jeong SY, You H, Won JY, et al. Extracellular vesicles from IFN-γ-primed mesenchymal stem cells repress atopic dermatitis in mice. J Nanobiotechnology. 2022; 20:526. PMID: 36496385.
69. A study to investigate the safety, tolerability, and efficacy of BxC-I17e single-dose SC injection in patients with moderate to severe atopic dermatitis. Bethesda: U.S. National Library of Medicine;2023. Accessed April 24, 2024. at https://clinicaltrials.gov/study/NCT06055361.
70. Lau HC, Han DW, Park J, Lehner E, Kals C, Arzt C, et al. GMP-compliant manufacturing of biologically active cell-derived vesicles produced by extrusion technology. J Extracell Biol. 2022; 1:e70. PMID: 38938599.
71. Caravan Biologix, Inc. collaborates with MDimune on cell-derived nanovesicles for cancer. San Francisco: Business Wire;Accessed April 24, 2024. at https://www.businesswire.com/news/home/20220915005157/en/Caravan-Biologix-Inc.-Collaborates-with-MDimune-on-Cell-Derived-NanoVesicles-for-Cancer.
72. MDimune wins bronze at 2023 Edison Awards. Seoul: NewsWire;2023. Accessed April 24, 2024. at https://www.newswire.co.kr/newsRead.php?no=965697.
73. GNV. Incheon: SL Bigen;Accessed April 24, 2024. at http://slbigen.com/?page_id=532.
74. Go G, Lee J, Choi DS, Kim SS, Gho YS. Extracellular vesicle-mimetic ghost nanovesicles for delivering anti-inflammatory drugs to mitigate Gram-negative bacterial outer membrane vesicle-induced systemic inflammatory response syndrome. Adv Healthc Mater. 2019; 8:e1801082. PMID: 30549424.
75. REX101. Incheon: SL Bigen;Accessed April 24, 2024. at http://slbigen.com/?page_id=558/#.
76. Won S, Lee C, Bae S, Lee J, Choi D, Kim MG, et al. Mass-produced Gram-negative bacterial outer membrane vesicles activate cancer antigen-specific stem-like CD8+ T cells which enables an effective combination immunotherapy with anti-PD-1. J Extracell Vesicles. 2023; 12:e12357. PMID: 37563797.
Fig. 1
Advantages and limitations of extracellular vesicle-based therapeutics. Extracellular vesicle-based therapeutics have advantages such as safety, targeting, and drug scalability (white), whereas they have limitations in that they require mass production and consistency/equivalence (gray). EPR, enhanced permeability and retention.
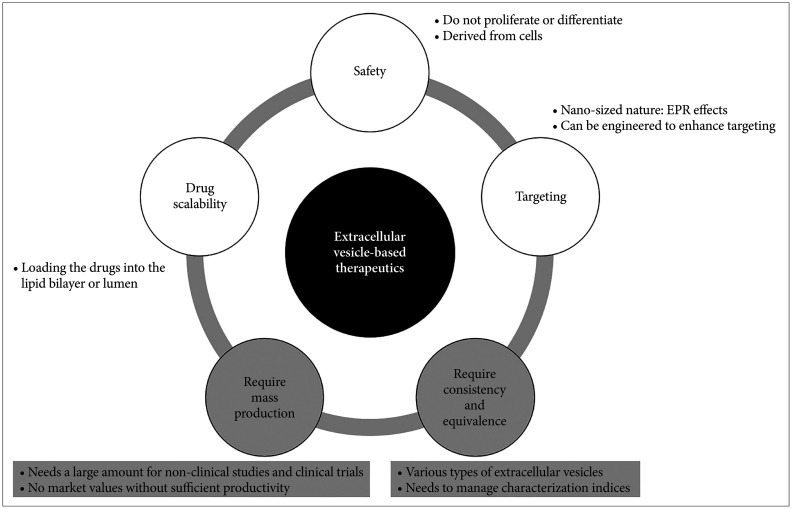
Table 1
Development cases of extracellular vesicle-based therapeutics by global biopharmaceutical companies
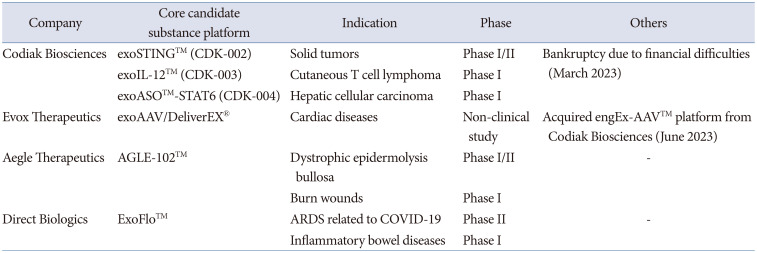
Table 2
Development cases of extracellular vesicle-based therapeutics by Korean biopharmaceutical companies
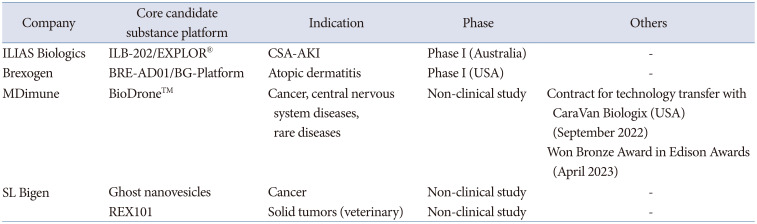