Abstract
Background
Cynara scolymus has bioactive constituents and has been used for therapeutic actions. The present study was undertaken to investigate the mechanisms underlying pain-relieving effects of the hydroethanolic extract of C. scolymus (HECS).
Methods
The antinociceptive activity of HECS was assessed through formalin and acetic acid-induced writhing tests at doses of 50, 100 and 200 mg/kg intraperitoneally. Additionally, naloxone (non-selective opioid receptors antagonist, 2 mg/kg), atropine (non-selective muscarinic receptors antagonist, 1 mg/kg), chlorpheniramine (histamine H1-receptor antagonist, 20 mg/kg), cimetidine (histamine H2-receptor antagonist, 12.5 mg/kg), flumazenil (GABAA/BDZ receptor antagonist, 5 mg/kg) and cyproheptadine (serotonin receptor antagonist, 4 mg/kg) were used to determine the systems implicated in HECS-induced analgesia. Impact of HECS on locomotor activity was executed by open-field test. Determination of total phenolic content (TPC) and total flavonoid content (TFC) was done. Evaluation of antioxidant activity was conducted employing 2,2-diphenyl-1-picrylhydrazyl (DPPH) free radical scavenging assay.
Results
HECS (50, 100 and 200 mg/kg) significantly indicated dose dependent antinociceptive activity against pain-related behavior induced by formalin and acetic acid (P < 0.001). Pretreatment with naloxone, atropine and flumazenil significantly reversed HECS-induced analgesia. Antinociceptive effect of HECS remained unaffected by chlorpheniramine, cimetidine and cyproheptadine. Locomotor activity was not affected by HECS. TPC and TFC of HECS were 59.49 ± 5.57 mgGAE/g dry extract and 93.39 ± 17.16 mgRE/g dry extract, respectively. DPPH free radical scavenging activity (IC50) of HECS was 161.32 ± 0.03 µg/mL.
Pain is a complex and multifactorial state that is characterized as a displeasing sensory and emotional encounter linked with harm to body tissues. Pain represents a pathological state, and being the prevailing indication of various illnesses, has the potential to impact the well-being of individuals [1,2]. Pain arises as a result of an injurious stimulus either from the environment or within the body and is subsequently disseminated by the activation of nociceptors by inflammatory mediators. Then, these receptors convey the pertinent information via afferent fibers to the central nervous system (CNS) [3]. Recently, in light of the deleterious consequences caused by a multitude of synthetic analgesics, including non-steroidal anti-inflammatory drugs (NSAIDs) and opioids, there has been a growing demand for the discovery of innovative analgesic medications that offer improved effectiveness and safety. In this regard, medicinal plants and their biologically active constituents have a significant impact on the treatment and regulation of pain [4].
Artichoke (Cynara scolymus L.) is cultivated in numerous nations, notably in Iran, Turkey, and China, and it falls under the Asteraceae botanical family. Investigations into the phytochemical properties of C. scolymus have revealed the existence of bioactive compounds, which encompass phenolic acids including chlorogenic acid and cynarin. Furthermore, the composition of the artichoke extract also comprises flavonoids such as apigenin, luteolin, and quercetin [5,6]. Experimental studies have reported multiple pharmacological effects associated with C. scolymus and its active constituents, including antioxidant [7], hypoglycemic [8], antiatherogenic, and hypolipidemic [9] activities. Additionally, the anti-inflammatory effect of artichoke extract has been proven through various demonstrations [10]. Moreover, previous studies have shown the hepatoprotective effect [11] and the anti-anemic potency [12] of the hydroethanolic extract of C. scolymus (HECS) against the development of hemolytic anemia induced by phenylhydrazine in rats.
Despite the wide range of biological impacts exerted by C. scolymus, there has been no documentation regarding the analgesic properties of this botanical specimen. Therefore, the present investigation sought to ascertain the antinociceptive efficacy of the hydroethanolic extract derived from C. scolymus, through the implementation of formalin and writhing tests in mice. Another objective of this study was to further investigate the potential mechanism(s) implicated in the analgesic effects of C. scolymus, with particular focus on the participation of opioidergic, cholinergic, histaminergic, GABAergic, and serotonergic pathways.
In this investigation, adult male albino NMRI mice, ranging in age from 8 to 10 weeks and weighing between 25 and 30 grams, were procured from the Animal Care Unit affiliated with the Faculty of Veterinary Medicine at Razi University situated in Kermanshah, Iran. The animals were accommodated within standard plastic enclosures (six mice per enclosure) within controlled environmental conditions at a temperature range of 22°C ± 2°C, accompanied by a relative humidity range of 55% ± 5%. This is further accompanied by a light-dark cycle of 12:12 hours, where the animals are exposed to equal periods of light and darkness. All animals were provided with a standard diet consisting of rodent pellets from Pars Dam Co. Additionally, fresh water was made available to them without restriction. The animals underwent a period of seven days for acclimatization before the initiation of the research investigation. Following the acclimatization period, the mice were employed in formalin and writhing tests. The utilization of animal experiments within this particular study underwent a thorough examination and received endorsement from the Animal Ethics Committee of Razi University (approved number: 98/581/1:98.11.27). Furthermore, the execution of said experiments adhered to the prescribed guidelines pertaining to the management and utilization of research animals for the purpose of exploring the concept of experimental pain in conscious animals [13]. To mitigate the potential impact of circadian variability on the nociceptive sensitivity, all experiments were carried out within the timeframe of 9–12 a.m. [14]. Diligent measures were implemented to alleviate pain and distress, as well as to minimize the quantity of experimental animals employed. Every individual mouse was tested only once.
Morphine sulfate was acquired from Temad Co. Indomethacin, naloxone hydrochloride, atropine, chlorpheniramine, cimetidine, flumazenil, cyproheptadine, Folin-Ciocalteu reagent, gallic acid (GA), rutin, 2,2-diphenyl-1-picrylhydrazyl (DPPH), and ascorbic acid were purchased from Sigma Chemical Co. Formaldehyde (37%), acetic acid, sodium carbonate (with the chemical formula Na2CO3), sodium nitrite (with the chemical formula NaNO2), aluminum chloride (with the chemical formula AlCl3), and sodium hydroxide (with the chemical formula NaOH) were obtained from Merck Co. All drugs and chemicals were dissolved in normal saline (0.9% NaCl) as the solvent. All solutions were newly prepared directly prior to intraperitoneal (i.p.) administration at a dosage of 10 mL/kg body weight. All the test doses of the drugs used in this study and drug administration schedules were determined based on the previous reports and the authors’ unpublished pilot studies [2,3,11,15,16].
The recently harvested leaves of C. scolymus were obtained from the Dineh Pharmaceutical Research Center, spanning the months of April to July in the year 2022. The expert in the field of botany carried out the process of identifying and authenticating the plant material. The leaves of the artichoke were subjected to a cleaning process, followed by slicing into smaller fragments. These fragments were then exposed to air-drying at room temperature while being shaded. Subsequently, the dried fragments were subjected to grinding with a laboratory grinder, resulting in the production of a powdered form. The powder underwent three rounds of extraction by employing a blend of ethanol and water (at a ratio of 70:30, v/v) over a period of 72 hours. The hydroethanolic extract effectively traversed the filter paper, specifically using Whatman No. 1 filter paper. The filtrate underwent a process of evaporation using a rotary evaporator within a vacuum setting, with the intention of removing the solvent, at a temperature of 40°C. The dried extract was preserved at a temperature of 4°C until it was necessary for implementation in the conducted investigations. Normal saline, a solution containing 0.9% sodium chloride, was introduced into the desiccated extract for pharmacological studies. The concentrations of C. scolymus at 50, 100, and 200 mg/kg were achieved just before use [11].
The quantification of the TPC present in the HECS was performed utilizing the Folin-Ciocalteu assay, as depicted in a previous study [17] with a few alterations. Briefly, the mixture was prepared by combining 1 mL of various concentrations of the HECS with 0.5 mL of the Folin-Ciocalteu reagent (1:10 v/v distilled water). After a period of incubation at ambient temperature lasting 3 minutes, a volume of 1 mL of Na2CO3, which possessed a concentration of 20% was introduced into the blend and permitted to remain undisturbed for a duration of 60 minutes in a location devoid of light. Following this, the absorbance was quantified at a wavelength of 725 nm utilizing a spectrophotometer (Human crop, Xma-2000). The calibration curve was devised employing GA within the concentration range of 12.5–200 µg/mL. The quantification of TPC was articulated in terms of mg of gallic acid equivalents (GAE) per gram of desiccated extract.
The assessment of the C. scolymus extract's TFC was conducted through the utilization of the aluminum chloride colorimetric assay [18], incorporating minor adjustments in the process. Initially, a combination of 1 mL of extract and 4 mL of distilled water was prepared. Following this, an additional volume of 0.3 mL of a solution containing 5% sodium nitrite (NaNO2) was introduced to a volumetric flask with a capacity of 10 mL. After 5 minutes, a volume of 0.3 mL of a solution containing 10% aluminium chloride (AlCl3) was introduced. After a duration of 6 minutes, an additional 2 mL of a 1 M solution of sodium hydroxide (NaOH) were introduced into the mixture, followed by the subsequent incorporation of 2.4 mL of distilled water. Subsequent to the incubation of the resulting mixture at ambient temperature for a period of 15 minutes, the absorbance of said mixture was assessed at a wavelength of 510 nm. The quantification of the TFC was then expressed as mg of rutin equivalents (RE) per gram of the desiccated extract.
The assessment of the antioxidant characteristic of the extract was conducted by means of the utilization of the DPPH assay for the eradication of free radicals [19,20], with a few alterations implemented. Briefly, 1 mL of different concentrations of the HECS were combined with 1 mL of DPPH solution, which contained 0.135 mM DPPH in methanol. The mixtures were shaken and maintained in darkness at room temperature for a duration of 30 minutes. The absorbance was measured at 517 nm on a visible light spectrophotometer. To act as a benchmark, ascorbic acid was employed as a positive control. The experiment was conducted three times independently, and an average of the outcomes was documented. The calculation of the DPPH radical scavenging activity (RSA) of the extract was determined by applying a mathematical equation to obtain the percentage inhibition.
Where the absorbance of the DPPH radical + methanol is denoted as Absorbance of control while the absorbance of the DPPH radical + sample extract is referred to as Absorbance of sample. The IC50 value was defined as a concentration (expressed in µg/mL) of sample needed to eliminate 50% of DPPH free radical.
The previous study [8] assessed the i.p. administration of HECS for acute toxicity. A total of 7 groups, each consisting of 6 mice, were randomly assigned. The mice were housed in controlled environments, where they were provided with ample amounts of food and water without any restrictions, except for a brief fasting period prior to the extract administration. The control group was treated with a dosage of 10 mL/kg of normal saline, while groups 2–6 were given increasing doses (50, 100, 200, 400, 600 and 1,000 mg/kg) of the artichoke extract. The behavior and neurological changes of the treated mice were continuously monitored for 1 hour after dosing, at regular intervals during the first 24 hours, and then at 72 hours to assess lethality.
The open-field test (OFT) was utilized to evaluate the potential impact of HECS on spontaneous locomotor activity and exploratory behavior in mice, as previously outlined [4,21]. The OFT was conducted within a transparent glass enclosure with dimensions precisely measured at 40 cm × 60 cm × 50 cm, featuring a floor divided into 12 equal squares. To allow for adaptation, mice from each respective group were individually introduced to the apparatus and given 30 minutes of acclimation time. Subsequently, the mice were administered either normal saline (10 mL/kg) or varying dosages of HECS (50, 100, and 200 mg/kg) via i.p. injection, and after a 30-minute interval, they were positioned in the center of the enclosure. The quantity of squares traversed with all paws and the frequency of rearings were meticulously documented over a 10-minute period.
The formalin test is a pain assessment procedure in mice [22]. To mitigate the activation of pain suppression mechanisms caused by stress, the mice were subjected to a pain test after being confined in an observation chamber made of plexiglas. This chamber had dimensions of 30 cm × 30 cm × 30 cm and featured a mirror positioned at a 45° angle beneath it. This confinement lasted for a duration of 30 minutes and was repeated for three consecutive days [23]. The current examination was carried out in conformity with the procedure delineated by Mohammadifard and Alimohammadi [3]. After a period of adaptation lasting 30 minutes, the mice were subjected to an injection of a 2% diluted formalin solution, measuring 50 µL, into the subcutaneous layer of the plantar surface of their right hind paw, using a 30-gauge needle. Subsequent to the formalin injection, the mice were promptly reintroduced into the observation chamber. The duration devoted to the action of licking and biting the paw that had been injected was documented as a nociceptive reaction within two designated time intervals: the initial phase, known as the neurogenic phase, spanning from 0–5 minutes, and the subsequent phase, referred to as the inflammatory phase, encompassing a timeframe of 15–45 minutes post formalin injection.
Seven different sets of experiments were examined in the formalin test in the subsequent manner. In experiment 1, animals were segregated into 5 distinct treatment groups with each group consisting of 6 animals and afterwards, for evaluating the antinociceptive effects of artichoke extract, vehicle (normal saline, 10 mL/kg), HECS (50, 100 and 200 mg/kg) and morphine (5 mg/kg) were administered intraperitoneally (i.p.) in each group 30 minutes before induction of formalin pain. The time which the animal engaged in the act of licking or biting the paw that had been injected was assessed in two separate stages subsequent to the administration of formalin, as previously stated. Subsequently, the optimal dosage of HECS was selected for the remaining experimental procedures.
In experiments 2 to 7, in order to investigate the various mechanisms through which HECS induces antinociception, the mice were partitioned into 4 groups, with each group consisting of 6 mice and subjected to different drug treatments. In experiment 2, a study was conducted to explore the potential involvement of the opioidergic system in the antinociceptive effects of the HECS. In order to achieve this, mice were pretreated with naloxone (a non-selective antagonist of opioid receptors, 2 mg/kg, i.p.) and 15 minutes later received HECS (200 mg/kg, i.p.) followed by the formalin test after 15 minutes. Other groups of animals received only vehicle (normal saline, 10 mL/kg), naloxone (2 mg/kg, i.p.), or HECS (200 mg/kg, i.p.). After 30 minutes, the animals were subjected to the formalin test. Experiments 3, 4, 5, 6, and 7 were similar to experiment 2 except that to investigate the possible involvement of the cholinergic, H1-histaminergic, H2-histaminergic, GABAergic, and serotonergic systems in the HECS-induced analgesic effect, mice were pretreated with atropine (a non-selective muscarinic cholinergic antagonist, 1 mg/kg, i.p.), chlorpheniramine (a histamine H1-receptor antagonist, 20 mg/kg, i.p.), cimetidine (a histamine H2-receptor antagonist, 12.5 mg/kg, i.p.), flumazenil (a GABAA/BDZ receptor antagonist, 5 mg/kg, i.p.), and cyproheptadine (a serotonin receptor antagonist, 4 mg/kg, i.p.) in place of naloxone, respectively, and 15 minutes later received HECS (200 mg/kg, i.p.) followed by the formalin test after 15 minutes. Similarly, as mentioned earlier in experiment 2, the remaining animal groups were administered with only vehicle (normal saline, 10 mL/kg), atropine (1 mg/kg, i.p.), chlorpheniramine (20 mg/kg, i.p.), cimetidine (12.5 mg/kg, i.p.), flumazenil (5 mg/kg, i.p.), and cyproheptadine (4 mg/kg, i.p.), respectively, or HECS (200 mg/kg, i.p.). After 30 minutes, the animals were subjected to the formalin test.
To assess the peripheral analgesic effect of HECS in a chemical visceral pain model, this experiment was conducted utilizing the previously documented technique [24,25]. The mice were introduced into a plexiglas observation chamber with dimensions of 40 cm × 30 cm × 20 cm for a period of 30 minutes in order to familiarize them with the experimental environment. Subsequently, the mice were subjected to the administration of the test preparations. After a period of 30 minutes, each mouse was administered with a dose of 10 mL/kg of acetic acid solution with a concentration of 0.6%, intraperitoneally. This administration was done using a 30-gauge injection needle. Following the immediate administration of acetic acid, the occurrence of abdominal writhing in each mouse was meticulously observed and documented for a duration of 30 minutes. Abdominal constriction, elongation of the body, and extension of at least one hind limb serve as distinct indicators of a writhe.
Seven sets of experiments in the acetic acid-induced writhing test were considered as follows. In experiment 1, animals were segregated into five treatment groups with each group consisting of 6 mice. To ascertain the antinociceptive impact of artichoke extract, the administration of vehicle, HECS (at a dosage equivalent to that of the formalin test), and indomethacin (at a dosage of 5 mg/kg) was performed via i.p. injection, 30 minutes prior to the acetic acid injection. Subsequently, the tally of writhes observed within a 30 minutes timeframe was recorded, and the most efficacious dosage of HECS was chosen for the subsequent experiments. Experiments 2 to 7 were formulated to evaluate the mechanisms implicated in the analgesic impact of artichoke extract. In order to achieve this objective, the mice were partitioned into 4 groups, each consisting of 6 mice, and subjected to various compounds. In experiment 2, the objective was to examine the potential involvement of the opioidergic system in the analgesic effects of HECS. To achieve this, mice were given a pretreatment of naloxone (2 mg/kg, i.p.) 15 minutes prior to the administration of HECS (200 mg/kg, i.p.). The calculation of the writhing nociceptive response, which arises from the injection of acetic acid with a concentration of 0.6%, was conducted after a time interval of 30 minutes subsequent to the administration of HECS. Additional animal groups were subjected to solely vehicle, naloxone (2 mg/kg, i.p.), or HECS (200 mg/kg, i.p.) 30 minutes prior to the injection of acetic acid. In experiment 3, the aim was to evaluate the interplay between the HECS compound and the cholinergic system. To achieve this, mice were pre-exposed to atropine (1 mg/kg, i.p.) 15 minutes prior to the administration of HECS (200 mg/kg, i.p.). The calculation of the nociceptive response, induced by the introduction of acetic acid (0.6%), was performed after a period of 30 minutes subsequent to the administration of HECS. Additional groups of animals were subjected solely to the administration of the vehicle, atropine (1 mg/kg, i.p.), or HECS (200 mg/kg, i.p.) 30 minutes before the injection of acetic acid. In order to explore the potential mediation of the histaminergic system in the antinociceptive effect of HECS, mice in experiments 4 and 5 were pre-administered with chlorpheniramine (20 mg/kg, i.p.) or cimetidine (12.5 mg/kg, i.p.), respectively. After a 15 minutes interval, the mice were subsequently administered HECS (200 mg/kg, i.p.). The calculation of the nociceptive response, which is characterized by writhing, was performed 30 minutes following the administration of HECS subsequent to the injection of acetic acid at a concentration of 0.6%. Other animal groups were administered with the vehicle, chlorpheniramine (20 mg/kg, i.p.), cimetidine (12.5 mg/kg, i.p.), or HECS (200 mg/kg, i.p.) just 30 minutes before the injection of acetic acid. In experiment 6, the purpose was to investigate the potential role of the GABAergic system in the antinociceptive effect of HECS. To accomplish this, mice were given a pretreatment of flumazenil (5 mg/kg, i.p.) 15 minutes prior to the administration of HECS (200 mg/kg, i.p.). The calculation of the nociceptive response, which is characterized by writhing, resulting from the injection of acetic acid (0.6%), was conducted after a period of 30 minutes subsequent to the administration of HECS. Groups of animals belonging to different categories were administered with either a control substance, flumazenil (5 mg/kg, i.p.), or HECS (200 mg/kg, i.p.), 30 minutes prior to the injection of acetic acid. In experiment 7, to investigate the possible contribution of the serotoninergic system in the analgesic effect of HECS, mice were pretreated with cyproheptadine (4 mg/kg, i.p.) 15 minutes before administration of HECS (200 mg/kg, i.p.). The calculation of the writhing nociceptive response, which is a result of the injection of acetic acid at a concentration of 0.6%, was performed after a time period of 30 minutes following the administration of HECS. Other categories of creatures were administered solely with vehicle, cyproheptadine (4 mg/kg, i.p.), or HECS (200 mg/kg, i.p.). After a time lapse of 30 minutes, these creatures were evaluated through the utilization of the acetic acid-induced writhing test.
The acquired data were meticulously prepared in Microsoft Excel software and consequently subjected to rigorous scrutiny by means of one-way analysis of variance and employing Tukey’s HSD post-hoc examination, utilizing the statistical software package SPSS Version 21 for Windows (SPSS, Inc.). The outcomes of the analysis were appropriately conveyed as means ± standard error of mean. It is worth noting that a P value that is lower than 0.05 was deemed indicative of a significant disparity between the various groups under investigation.
In the experiment evaluating the acute toxicity of the HECS on the mice, it was noted that there were no noteworthy alterations in behavior or mortality rates among them when subjected to increasing doses of the HECS (50–1,000 mg/kg) following a singular i.p. administration, within the initial 72 hours observation period. Therefore, LD50 of the C. scolymus extract was deemed to exceed 1,000 mg/kg.
The administration of formalin 2% via intraplantar injection elicited a biphasic pain-related response encompassing both the first and the second phases. The administration of HECS at a dosage of 50 mg/kg did not exhibit any discernible impact on the first phase (P = 0.158), conversely, it notably inhibited the second phase of formalin-induced pain [F(4,25) = 42.87, P = 0.011]. However, HECS (100 and 200 mg/kg) produced significant antinociception in a dose-dependent manner in the first phase [F(4,25) = 52.27, P < 0.001] as well as the second phase [F(4,25) = 42.87, P < 0.001]. As anticipated, the conventional medication morphine exhibited a substantial decrease in the nociceptive reaction during both the first [F(4,25) = 52.27, P < 0.001] and second [F(4,25) = 42.87, P < 0.001] stages of the formalin test (Fig. 1A).
Since the dose of 200 mg/kg of the HECS exhibited the highest rate of efficacy, all subsequent experiments pertaining to the exploration of the mechanisms governing the analgesic impact of the extract were conducted using this dose.
The administration of naloxone (2 mg/kg) alone did not yield any statistically significant outcome during both the first phase (P = 0.971) and second phase (P = 0.962). HECS, administered at a dose of 200 mg/kg, exhibited a significant reduction in the duration of licking and biting of the paw upon injection during both the first [F(3,20) = 9.73, P < 0.001] and second [F(3,20) = 17.17, P < 0.001] pain phases. Furthermore, prior administration of naloxone (2 mg/kg) effectively counteracted the antinociceptive effects induced by the administration of HECS (200 mg/kg) during both the first [F(3,20) = 9.73, P = 0.015] and second [F(3,20) = 17.17, P = 0.009] pain phases (Fig. 1B).
Atropine administered at a dose of 1 mg/kg did not induce any significant alteration in pain intensity during both the first (P = 0.960) and second (P = 0.851) phases. The administration of HECS at a dose of 200 mg/kg led to a notable reduction in pain induced by formalin during both the first [F(3,20) = 16.47, P < 0.001] and second [F(3,20) = 11.73, P < 0.001] phases of the experiment. Furthermore, the pretreatment with atropine at a dose of 1 mg/kg significantly impeded the analgesic effect of HECS at a dose of 200 mg/kg, but specifically during the second phase of pain [F(3,20) = 11.73, P = 0.027] (Fig. 1C).
Injection of chlorpheniramine (20 mg/kg) alone did not elicit any significant change in the pain response during both the first and second phases (P = 0.986 and P = 0.910, respectively). The administration of HECS (200 mg/kg) effectively suppressed nociception in both the first [F(3,20) = 20.70, P < 0.001] and second [F(3,20) = 32.43, P < 0.001] phases of the formalin test. Interestingly, the antinociceptive activity of HECS (200 mg/kg) remained unaffected by prior treatment with chlorpheniramine in both the first (P = 0.976) and second (P = 0.998) phases of the test (Fig. 1D). Similarly, the injection of cimetidine (12.5 mg/kg) alone had no significant impact on the pain response observed during the first (P = 0.910) and second (P = 0.979) phases. Moreover, a notable suppression of formalin-induced nociception was observed with the administration of HECS (200 mg/kg) in both the first [F(3,20) = 19.25, P < 0.001] and second [F(3,20) = 28.11, P < 0.001] phases of the test. Notably, pretreatment with cimetidine failed to reverse the analgesic effect of HECS (200 mg/kg) in both the first (P = 0.994) and second (P = 0.997) phases of the test (Fig. 1E).
Flumazenil (5 mg/kg) did not yield any substantial impact on the pain response during the first phase (P = 0.680) or the second phase (P = 0.712). Conversely, the administration of HECS (200 mg/kg) considerably impeded the pain experienced during both the first phase [F(3,20) = 10.96, P < 0.001] and the second phase [F(3,20) = 13.32, P < 0.001] of formalin-induced pain. Furthermore, prior treatment of mice with flumazenil (5 mg/kg) significantly counteracted the antinociceptive response induced by HECS (200 mg/kg) during both the first phase [F(3,20) = 10.96, P = 0.004] and the second phase [F(3,20) = 13.32, P = 0.003] of pain behavior (Fig. 1F).
The administration of cyproheptadine (4 mg/kg) did not result in any considerable changes in pain throughout either the first (P = 0.829) or second (P = 0.985) phases. However, when HECS (200 mg/kg) was administered, there was a notable decrease in pain behaviors caused by formalin in both the first [F(3,20) = 12.98, P < 0.001] and second [F(3,20) = 24.79, P < 0.001] phases of the test. Interestingly, pretreatment with cyproheptadine (4 mg/kg) did not reverse the observed antinociception after the administration of HECS (200 mg/kg) in both the first (P = 0.992) and second (P = 0.869) phases of the test (Fig. 1G).
The extract derived from the C. scolymus exhibited a substantial and dose-dependent decline in the count of acetic acid-induced writhing episodes in response to doses of 50, 100, and 200 mg/kg, in contrast to the control group [F(4,25) = 19.36, P = 0.041, P = 0.013, P < 0.001, respectively]. Furthermore, the utilization of indomethacin (5 mg/kg) exhibited a notable reduction in the occurrence of writhing episodes in contrast to the control group [F(4,25) = 19.36, P < 0.001] (Fig. 2A).
Given that the HECS (200 mg/kg) was shown to be the most efficacious, all subsequent inquiries into the elucidation of the underlying mechanisms of the extract's antinociceptive effect were done using this dose.
Naloxone administered at a dose of 2 mg/kg did not elicit any notable alteration in the acetic acid-induced writhing response (P = 0.960). The administration of HECS (200 mg/kg) resulted in a marked decrease in the occurrence of abdominal writhes in comparison to the control group [F(3,20) = 14.88, P < 0.001]. Furthermore, the analgesic effect caused by HECS was markedly diminished when naloxone (2 mg/kg) was given before the HECS (200 mg/kg) [F(3,20) = 14.88, P = 0.002] (Fig. 2B).
Atropine (1 mg/kg) exhibited no discernible effect on pain intensity when administered independently (P = 0.994). Conversely, the administration of HECS (200 mg/kg) led to a significant reduction in the number of writhings induced by acetic acid [F(3,20) = 14.64, P < 0.001]. Furthermore, pretreatment with atropine (1 mg/kg) antagonized the HECS (200 mg/kg)-induced antinociception [F(3,20) = 14.64, P = 0.033] (Fig. 2C).
Chlorpheniramine (20 mg/kg) did not elicit any discernible impact on the quantity of abdominal writhes (P = 0.999). In contrast, the administration of HECS (200 mg/kg) significantly diminished the number of abdominal writhes when compared to the control group [F(3,20) = 26.19, P < 0.001]. It is noteworthy to mention that the analgesic effect produced by HECS (200 mg/kg) remained unaffected by prior treatment with chlorpheniramine (20 mg/kg) (P = 0.989) (Fig. 2D). On the other hand, the administration of cimetidine (12.5 mg/kg) alone did not yield any noteworthy alteration in nociceptive behavior (P = 0.737). In contrast, HECS (200 mg/kg) displayed a substantial antinociceptive effect against acetic acid-induced nociception [F(3,20) = 18.42, P < 0.001]. Furthermore, pretreatment with cimetidine (12.5 mg/kg) did not impede the antinociceptive activity of HECS (200 mg/kg) (P = 0.859) (Fig. 2E).
Flumazenil (5 mg/kg) alone did not yield any impact on the magnitude of pain experienced (P = 0.515). Conversely, when HECS (200 mg/kg) was administered, it successfully alleviated the writhing responses observed in mice due to the introduction of acetic acid [F(3,20) = 16.37, P < 0.001]. Furthermore, the HECS-induced antinociception was completely nullified with the prior administration of flumazenil at a dose of 5 mg/kg [F(3,20) = 16.37, P = 0.019] (Fig. 2F).
Cyproheptadine (4 mg/kg) did not elicit any effect on the nociceptive response associated with writhing (P = 0.998). Conversely, the administration of HECS (200 mg/kg) demonstrated a notable and considerable decrease in the occurrence of abdominal writhes [F(3,20) = 22.45, P < 0.001]. In spite of that, it is noteworthy to mention that the HECS's ability to inhibit the writhing response exhibited no alteration whatsoever, even in situations where the mice were administered cyproheptadine as a pretreatment at a dosage of 4 mg/kg (P = 0.910) (Fig. 2G).
In the current investigation, the administration of HECS using doses of 50, 100, and 200 mg/kg, intraperitoneally, did not elicit any discernible impact on the locomotor activity observed in the OFT. The number of crossings [F(3,20) = 1.582, P = 0.225] and rearings [F(3,20) = 2.058, P = 0.138] in the groups treated with HECS did not exhibit any remarkable divergence when comparing with the control group during a duration of 10 minutes (Fig. 3).
To the best of the authors’ understanding, this study presents the initial account of the analgesic attribute exhibited by the C. scolymus and its potential interplay with the opioidergic, cholinergic, histaminergic, GABAergic, and serotonergic mechanisms. These interactions were explored by means of chemical-induced pain models, specifically the formalin and acetic acid-induced writhing tests conducted on mice.
Pain is defined as a distressing sensation that is associated with harmful external stimuli, which may induce tissue damage [26]. In recent times, a multitude of thorough and comprehensive inquiries have been conducted in relation to the efficacious botanical species because of their advantageous impact on pain management. When formalin is injected into the plantar region of the hind paw of rodents, it results in the manifestation of a distinct and recognizable two-phase pattern of nociceptive behaviors, wherein different mechanisms of pain modulation contribute. The first phase, known as the neurogenic phase, occurs immediately following formalin administration and is a consequence of direct activation of C-fiber nociceptors located in the dorsal horn region of the spinal cord. The suppression of this particular phase is a possibility that can be achieved through the administration of centrally acting analgesics. The second phase, referred to as the inflammatory phase, arises from the liberation of various inflammatory mediators exemplified by bradykinin, histamine, prostaglandins, and serotonin subsequent to formalin-induced tissue damage. The use of NSAIDs and steroids can effectively alleviate this particular phase, alongside compounds that act centrally [27]. The well-documented writhing test induced by acetic acid provides evidence for the engagement of peripheral mechanisms. This phenomenon can be attributed to the fact that the presence of acetic acid has been found to elicit a response in the form of the activation and subsequent release of various inflammatory mediators and cytokines including interleukin (IL)-1β, IL-8, and TNF-α. It has been observed that resident peritoneal macrophages and mast cells are the primary sources of these chemical messengers [28]. Consequently, this test is utilized to evaluate the effectiveness of novel compounds possessing peripheral analgesic activity [29].
The results of the current investigation clearly demonstrated that the i.p. delivery of the HECS exhibited a considerable decline in both the length of time spent licking and biting the injected paw, thereby highlighting the noteworthy impact and dependency on the dosage of this extract during both phases of the formalin test. Additionally, the HECS also demonstrated a remarkable reduction in the quantity of abdominal writhes noted during the acetic acid-induced writhing test. Given the antinociceptive effect observed in these two nociceptive models, it can be inferred that the HECS operates through both central and peripheral mechanisms.
Based on the results of the present research, TPC and TFC of the artichoke extract were 59.49 ± 5.57 (mgGAE/g dry extract) and 93.39 ± 17.16 (mgRE/g dry extract), respectively. In addition, the DPPH RSA (a method to measure the antioxidant activity) of the extract, as indicated by IC50 value, was 161.32 ± 0.03 µg/mL. Based on literature data, numerous phenolic acids and flavonoid compounds have been detected in the chemical composition of C. scolymus [5,6]. The exploration of these substances as agents with anti-inflammatory, antinociceptive, and antioxidant properties in the context of medicinal plants has been the subject of investigation [30]. For example, an antinociceptive effect was observed when chlorogenic acid was administered in the experimental model of neuropathic pain induced by streptozotocin in rats [31]. Furthermore, chlorogenic acid demonstrated its antinociceptive and anti-edematogenic properties in animal models of inflammation evoked by carrageenin and pain induced by formalin in rats [32]. By inhibiting the stimulation of NF-κB and JNK/AP-1 signaling routes, chlorogenic acid was able to suppress lipopolysaccharides-induced COX-2 expression, thereby exhibiting its anti-inflammatory action. Additionally, it was found that chlorogenic acid exerts a potent inhibitory effect on the synthesis of PGE2, further supporting its anti-inflammatory action [33]. Apigenin, a naturally occurring flavonoid, demonstrated its efficacy in alleviating the abdominal constrictions elicited by acetic acid and also in mitigating the licking and biting response evoked by formalin during the early and late stages of the experiment [34]. Furthermore, luteolin, another flavonoid compound, exhibited its anti-inflammatory properties [35] and elicited analgesic effects when administered to mice suffering from acetic acid-induced abdominal writhing. In addition, this compound has also been shown to alleviate the second phase of pain behavior induced by formalin administration [36]. Ethnopharmacological investigations have additionally documented that quercetin possesses the ability to impede nociceptive responses in pain scenarios induced by the presence of acetic acid, capsaicin, formalin, glutamate, as well as p-benzoquinone, thereby inhibiting the ensuing pain and preventing the elicitation of the writhing reflex in mice [37,38]. Considering the aforementioned data, it can be deduced that the existence and availability of phenolic acids and flavonoid compounds within HECS may potentially contribute, to a certain extent, to the antinociceptive property observed in the current study. The HECS administration at varying doses of 50, 100, and 200 mg/kg did not demonstrate any discernible impact on the locomotor activity during OFT compared with mice receiving normal saline solution. This finding implies that the pain-relieving activity observed in this study was not linked to any nonspecific disruptions in motor performance or sedative repercussions.
Based on the objective of the current investigation, a further exploration of the potential mechanisms contributing to the analgesic properties of HECS was conducted. In this context, an assessment was made on the pain-alleviating impact triggered by HECS, with a specific focus on the involvement of opioidergic, cholinergic, histaminergic, GABAergic, and serotonergic pathways in formalin- and acetic acid-induced pain-related behaviors. It has been documented that the engagement of diverse neurotransmitter systems and signaling pathways in the antinociceptive activity of novel therapeutic agents, including medicinal plants, has been previously documented [39].
The endogenous opioidergic system, along with its receptor subtypes, including mu (µ), delta (δ), and kappa (κ), is situated in both the CNS and peripheral tissues. It actively participates in a variety of functions, such as pain modulation, analgesia, tolerance, and dependence [40]. Naloxone, an opioid receptor antagonist that exhibits non-selectivity towards these receptors, effectively hinders the binding of opioid receptors in both the spinal and supraspinal regions [3]. The current investigation showed that the preliminary administration of naloxone to the experimental mice effectively and remarkably reversed the antinociceptive and pain-relieving properties elicited by HECS, not only during the first stage but also during the second phase of the formalin test, in addition to the acetic acid-induced writhing test. This finding strongly suggests the active participation of opioid receptors in the profound analgesic properties of HECS.
Cholinergic receptors hold significant importance in the intricate process of transmission and modulation of nociceptive information in both the central and peripheral nervous system [41,42]. It is evident that the administration of atropine, a pharmacological compound that acts as a non-selective antagonist of muscarinic receptors, in mice resulted in the notable suppression of the antinociceptive effect of HECS only during the second phase of the formalin test as well as the acetic acid-induced writhing test. However, the antinociceptive effect of HECS during the initial phase of the formalin pain model remained unaltered. This observation implies that the engagement of cholinergic receptors plays a pivotal role in the analgesic mechanism of action of the HECS.
Another crucial pain regulatory system in the CNS is the GABAergic system. GABA serves as the primary inhibitory neurotransmitter within the CNS. GABA receptors are distributed extensively throughout various levels of the pain pathway, where they play a crucial role in facilitating inhibitory actions and modulating the processing of nociceptive information [43,44]. The authors’ findings demonstrate that the analgesic effects of HECS were significantly reduced when mice were pretreated with flumazenil, an antagonist of GABAA/BDZ receptor, in models of chemically-induced nociception involving formalin and acetic acid. Reversal of HECS-induced analgesia following pretreatment with flumazenil suggested the potential engagement of GABA receptors in mediating the analgesic properties of HECS. These results align with a previous study that reported a partial reversal of the analgesic efficacy of chlorogenic acid through the administration of bicuculline, an antagonist of GABAA receptor, in neuropathic pain in an experimental rat model [45].
The involvement of histaminergic [46] and serotonergic [47,48] systems in the nociceptive transmission and modulation process in rodents has been extensively documented in previous research. However, the findings discovered in the current study offer empirical evidence which strongly suggest that pretreatment of mice with chlorpheniramine (a medication that acts as an antagonist of histamine H1-receptor), cimetidine (a type of drug that works by blocking the action of histamine at the H2 receptor), and cyproheptadine (a serotonin receptor antagonist) did not have any impact on the HECS-induced analgesia. This suggests that the analgesic activity of the HECS is not mediated through the histaminergic and serotonergic pathways.
The current study had several important findings and distinctive strengths, such as using various pharmacological experiments to elucidate neurotransmitter systems and signaling pathways involved in the analgesic mechanism exerted by HECS and also the determination of TPC, TFC and antioxidant activity of the HECS. It is noteworthy, however, that there exist some limitations when employing HECS in daily life. For example, further clinical investigations and long-termuseof C. scolymus are necessary to establish the effectiveness of HECS in the alleviation of pain in human subjects. Moreover, further fundamental research is warranted to explore the involvement of alternate neurotransmitter pathways and neuronal receptors in the analgesic impact of HECS, with the utilization of specific agonists and antagonists at these receptor sites.
Conclusively, the findings of the current investigation establish, in a pioneering manner, that the HECS elicits a potent antinociceptive response in mice subjected to chemical-induced nociception models, and this effect is contingent upon the dosage administered. Furthermore, based on these findings, it can be inferred that the aforementioned antinociceptive effect is, at least partially, influenced by the participation of opioidergic, cholinergic, and GABAergic pathways. However, additional research endeavors are necessary in order to provide further clarification regarding the complex and multifaceted nature of the intricate cellular and molecular signaling routes that underlie these observed phenomena.
ACKNOWLEDGMENTS
This study received financial backing from a grant provided by the Research Council of the Faculty of Veterinary Medicine at Razi University, located in Iran. The authors hereby extend their gratitude to Dr. Alireza Abdolmohammadi for his valuable aid.
Notes
DATA AVAILABILITY
The datasets that provide the necessary support for the discovery and conclusion of this particular study are readily accessible and obtainable from the author who is responsible for this research. This availability is contingent upon the condition that a reasonable and justified request is made.
REFERENCES
1. eekeesoon DP Sr, Mahomoodally MF. 2014; Ethnopharmacological analysis of medicinal plants and animals used in the treatment and management of pain in Mauritius. J Ethnopharmacol. 157:181–200. DOI: 10.1016/j.jep.2014.09.030. PMID: 25261690.


2. Olorukooba AB, Odoma S. 2019; Elucidation of the possible mechanism of analgesic action of methanol stem bark extract of Uapaca togoensis pax in mice. J Ethnopharmacol. 245:112156. DOI: 10.1016/j.jep.2019.112156. PMID: 31415847.


3. Mohammadifard F, Alimohammadi S. 2018; Chemical composition and role of opioidergic system in antinociceptive effect of Ziziphora Clinopodioides essential oil. Basic Clin Neurosci. 9:357–66. DOI: 10.32598/bcn.9.5.357. PMID: 30719250. PMCID: PMC6360493.
4. Koohsari S, Sheikholeslami MA, Parvardeh S, Ghafghazi S, Samadi S, Poul YK, et al. 2020; Antinociceptive and antineuropathic effects of cuminaldehyde, the major constituent of Cuminum cyminum seeds: possible mechanisms of action. J Ethnopharmacol. 255:112786. DOI: 10.1016/j.jep.2020.112786. PMID: 32222574.
5. Ben Salem M, Affes H, Ksouda K, Dhouibi R, Sahnoun Z, Hammami S, et al. 2015; Pharmacological studies of artichoke leaf extract and their health benefits. Plant Foods Hum Nutr. 70:441–53. DOI: 10.1007/s11130-015-0503-8. PMID: 26310198.


6. Mejri F, Baati T, Martins A, Selmi S, Luisa Serralheiro M, Falé PL, et al. 2020; Phytochemical analysis and in vitro and in vivo evaluation of biological activities of artichoke (Cynara scolymus L.) floral stems: towards the valorization of food by-products. Food Chem. 333:127506. DOI: 10.1016/j.foodchem.2020.127506. PMID: 32679417.


7. Salekzamani S, Ebrahimi-Mameghani M, Rezazadeh K. 2019; The antioxidant activity of artichoke (Cynara scolymus): a systematic review and meta-analysis of animal studies. Phytother Res. 33:55–71. DOI: 10.1002/ptr.6213. PMID: 30345589.


8. Ben Salem M, Ben Abdallah Kolsi R, Dhouibi R, Ksouda K, Charfi S, Yaich M, et al. 2017; Protective effects of Cynara scolymus leaves extract on metabolic disorders and oxidative stress in alloxan-diabetic rats. BMC Complement Altern Med. 17:328. DOI: 10.1186/s12906-017-1835-8. PMID: 28629341. PMCID: PMC5477270.


9. Mocelin R, Marcon M, Santo GD, Zanatta L, Sachett A, Schönell AP, et al. 2016; Hypolipidemic and antiatherogenic effects of Cynara scolymus in cholesterol-fed rats. Rev Bras Farmacognosia. 26:233–9. DOI: 10.1016/j.bjp.2015.11.004.
10. Ben Salem M, Affes H, Athmouni K, Ksouda K, Dhouibi R, Sahnoun Z, et al. 2017; Chemicals compositions, antioxidant and anti-inflammatory activity of Cynara scolymus leaves extracts, and analysis of major bioactive polyphenols by HPLC. Evid Based Complement Alternat Med. 2017:4951937. DOI: 10.1155/2017/4951937. PMID: 28539965. PMCID: PMC5429947.


11. Alahmoradi M, Alimohammadi S, Cheraghi H. 2019; Protective effect of Cynara scolymus L. on blood biochemical parameters and liver histopathological changes in phenylhydrazine-induced hemolytic anemia in rats. Pharm Biomed Res. 5:53–62. DOI: 10.18502/pbr.v5i4.2397.
12. Allahmoradi M, Alimohammadi S, Cheraghi H. 2020; Amelioration of lipid peroxidation and antioxidant enzymes status in the serum and erythrocytes of phenylhydrazine-induced anemic male rats: the protective role of artichoke extract (Cynara scolymus L.). Iran J Vet Med. 14:315–28.
13. Zimmermann M. 1983; Ethical guidelines for investigations of experimental pain in conscious animals. Pain. 16:109–10. DOI: 10.1016/0304-3959(83)90201-4. PMID: 6877845.


14. Kavaliers M, Hirst M. 1983; Daily rhythms of analgesia in mice: effects of age and photoperiod. Brain Res. 279:387–93. DOI: 10.1016/0006-8993(83)90216-0. PMID: 6315182.


15. Montiel-Ruiz RM, González-Trujano ME, Déciga-Campos M. 2013; Synergistic interactions between the antinociceptive effect of Rhodiola rosea extract and B vitamins in the mouse formalin test. Phytomedicine. 20:1280–7. DOI: 10.1016/j.phymed.2013.07.006. PMID: 23920277.


16. Zendehdel M, Torabi Z, Hassanpour S. 2015; Antinociceptive mechanisms of Bunium persicum essential oil in the mouse writhing test: role of opioidergic and histaminergic systems. Vet Med. 60:63–70. DOI: 10.17221/7988-VETMED.


17. Oliveira AS, Cercato LM, de Santana Souza MT, Melo AJO, Lima BDS, Duarte MC, et al. 2017; The ethanol extract of Leonurus sibiricus L. induces antioxidant, antinociceptive and topical anti-inflammatory effects. J Ethnopharmacol. 206:144–51. DOI: 10.1016/j.jep.2017.05.029. PMID: 28549861.


18. Zhishen J, Mengcheng T, Jianming W. 1999; The determination of flavonoid contents in mulberry and their scavenging effects on superoxide radicals. Food Chem. 64:555–9. DOI: 10.1016/S0308-8146(98)00102-2.


19. Blois MS. 1958; Antioxidant determinations by the use of a stable free radical. Nature. 181:1199–200. DOI: 10.1038/1811199a0.
20. Khalil M, Khalifeh H, Baldini F, Salis A, Damonte G, Daher A, et al. 2019; Antisteatotic and antioxidant activities of Thymbra spicata L. extracts in hepatic and endothelial cells as in vitro models of non-alcoholic fatty liver disease. J Ethnopharmacol. 239:111919. DOI: 10.1016/j.jep.2019.111919. PMID: 31029756.


21. Tamaddonfard E, Hamzeh-Gooshchi N. 2010; Effect of crocin on the morphine-induced antinociception in the formalin test in rats. Phytother Res. 24:410–3. DOI: 10.1002/ptr.2965. PMID: 19653196.


22. Dubuisson D, Dennis SG. 1977; The formalin test: a quantitative study of the analgesic effects of morphine, meperidine, and brain stem stimulation in rats and cats. Pain. 4:161–74. DOI: 10.1016/0304-3959(77)90130-0. PMID: 564014.


23. Abbott FV, Bonder M. 1997; Options for management of acute pain in the rat. Vet Rec. 140:553–7. DOI: 10.1136/vr.140.21.553. PMID: 9185312.


24. Koster R, Anderson M, De Beer EJ. 1959; Acetic acid for analgesic screening. Fed Proc. 18:412–7.
25. Hishe HZ, Ambech TA, Hiben MG, Fanta BS. 2018; Anti-nociceptive effect of methanol extract of leaves of Senna singueana in mice. J Ethnopharmacol. 217:49–53. DOI: 10.1016/j.jep.2018.02.002. PMID: 29421592.


26. Yam MF, Loh YC, Tan CS, Khadijah Adam S, Abdul Manan N, Basir R. 2018; General pathways of pain sensation and the major neurotransmitters involved in pain regulation. Int J Mol Sci. 19:2164. DOI: 10.3390/ijms19082164. PMID: 30042373. PMCID: PMC6121522.


27. Hunskaar S, Hole K. 1987; The formalin test in mice: dissociation between inflammatory and non-inflammatory pain. Pain. 30:103–14. DOI: 10.1016/0304-3959(87)90088-1. PMID: 3614974.


28. Ribeiro RA, Vale ML, Thomazzi SM, Paschoalato AB, Poole S, Ferreira SH, et al. 2000; Involvement of resident macrophages and mast cells in the writhing nociceptive response induced by zymosan and acetic acid in mice. Eur J Pharmacol. 387:111–8. DOI: 10.1016/S0014-2999(99)00790-6. PMID: 10633169.


29. Karbab A, Mokhnache K, Ouhida S, Charef N, Djabi F, Arrar L, et al. 2020; Anti-inflammatory, analgesic activity, and toxicity of Pituranthos scoparius stem extract: an ethnopharmacological study in rat and mouse models. J Ethnopharmacol. 258:112936. DOI: 10.1016/j.jep.2020.112936. PMID: 32376367.


30. Ferraz CR, Carvalho TT, Manchope MF, Artero NA, Rasquel-Oliveira FS, Fattori V, et al. 2020; Therapeutic potential of flavonoids in pain and inflammation: mechanisms of action, pre-clinical and clinical data, and pharmaceutical development. Molecules. 25:762. DOI: 10.3390/molecules25030762. PMID: 32050623. PMCID: PMC7037709.


31. Bagdas D, Ozboluk HY, Cinkilic N, Gurun MS. 2014; Antinociceptive effect of chlorogenic acid in rats with painful diabetic neuropathy. J Med Food. 17:730–2. DOI: 10.1089/jmf.2013.2966. PMID: 24611441.


32. dos Santos MD, Almeida MC, Lopes NP, de Souza GE. 2006; Evaluation of the anti-inflammatory, analgesic and antipyretic activities of the natural polyphenol chlorogenic acid. Biol Pharm Bull. 29:2236–40. DOI: 10.1248/bpb.29.2236. PMID: 17077520.


33. Shan J, Fu J, Zhao Z, Kong X, Huang H, Luo L, et al. 2009; Chlorogenic acid inhibits lipopolysaccharide-induced cyclooxygenase-2 expression in RAW264.7 cells through suppressing NF-kappaB and JNK/AP-1 activation. Int Immunopharmacol. 9:1042–8. DOI: 10.1016/j.intimp.2009.04.011. PMID: 19393773.
34. Pinheiro MM, Boylan F, Fernandes PD. 2012; Antinociceptive effect of the Orbignya speciosa Mart. (Babassu) leaves: evidence for the involvement of apigenin. Life Sci. 91:293–300. DOI: 10.1016/j.lfs.2012.06.013. PMID: 22749864.


35. Aziz N, Kim MY, Cho JY. 2018; Anti-inflammatory effects of luteolin: a review of in vitro, in vivo, and in silico studies. J Ethnopharmacol. 225:342–58. DOI: 10.1016/j.jep.2018.05.019. PMID: 29801717.


36. Miño J, Acevedo C, Moscatelli V, Ferraro G, Hnatyszyn O. 2002; Antinociceptive effect of the aqueous extract of Balbisia calycina. J Ethnopharmacol. 79:179–82. DOI: 10.1016/S0378-8741(01)00372-5. PMID: 11801379.


37. Filho AW, Filho VC, Olinger L, de Souza MM. 2008; Quercetin: further investigation of its antinociceptive properties and mechanisms of action. Arch Pharm Res. 31:713–21. DOI: 10.1007/s12272-001-1217-2. PMID: 18563352.


38. Toker G, Küpeli E, Memisoğlu M, Yesilada E. 2004; Flavonoids with antinociceptive and anti-inflammatory activities from the leaves of Tilia argentea (silver linden). J Ethnopharmacol. 95:393–7. DOI: 10.1016/j.jep.2004.08.008. PMID: 15507365.


39. Yousofvand N, Moloodi B. 2023; An overview of the effect of medicinal herbs on pain. Phytother Res. 37:1057–81. DOI: 10.1002/ptr.7697. PMID: 36585701.
40. Bodnar RJ. 2020; Endogenous opiates and behavior:. Peptides. 132:170348. DOI: 10.1016/j.peptides.2020.170348. PMID: 32574695.
41. Eisenach JC. 1999; Muscarinic-mediated analgesia. Life Sci. 64:549–54. DOI: 10.1016/S0024-3205(98)00600-6. PMID: 10069522.


42. Naser PV, Kuner R. 2018; Molecular, cellular and circuit basis of cholinergic modulation of pain. Neuroscience. 387:135–48. DOI: 10.1016/j.neuroscience.2017.08.049. PMID: 28890048. PMCID: PMC6150928.


43. Goudet C, Magnaghi V, Landry M, Nagy F, Gereau RW 4th, Pin JP. 2009; Metabotropic receptors for glutamate and GABA in pain. Brain Res Rev. 60:43–56. DOI: 10.1016/j.brainresrev.2008.12.007. PMID: 19146876.


44. Mahdian Dehkordi F, Kaboutari J, Zendehdel M, Javdani M. 2019; The antinociceptive effect of artemisinin on the inflammatory pain and role of GABAergic and opioidergic systems. Korean J Pain. 32:160–7. DOI: 10.3344/kjp.2019.32.3.160. PMID: 31257824. PMCID: PMC6615442.


45. Hara K, Haranishi Y, Kataoka K, Takahashi Y, Terada T, Nakamura M, et al. 2014; Chlorogenic acid administered intrathecally alleviates mechanical and cold hyperalgesia in a rat neuropathic pain model. Eur J Pharmacol. 723:459–64. DOI: 10.1016/j.ejphar.2013.10.046. PMID: 24184666.
46. Obara I, Telezhkin V, Alrashdi I, Chazot PL. 2020; Histamine, histamine receptors, and neuropathic pain relief. Br J Pharmacol. 177:580–99. DOI: 10.1111/bph.14696. PMID: 31046146. PMCID: PMC7012972.


47. Li P, Zhuo M. 2001; Cholinergic, noradrenergic, and serotonergic inhibition of fast synaptic transmission in spinal lumbar dorsal horn of rat. Brain Res Bull. 54:639–47. DOI: 10.1016/S0361-9230(01)00470-1. PMID: 11403990.
48. Martinello K, Sucapane A, Fucile S. 2022; 5-HT3 receptors in rat dorsal root ganglion neurons: Ca2+ entry and modulation of neurotransmitter release. Life (Basel). 12:1178. DOI: 10.3390/life12081178. PMID: 36013357. PMCID: PMC9409985.


Fig. 1
Effect of HECS (50, 100 and 200 mg/kg) and morphine (5 mg/kg) on the formalin-induced pain responses (A). Effect of pretreatment with naloxone (non-selective opioid receptors antagonist, 2 mg/kg) (B), atropine (non-selective muscarinic receptor antagonist, 1 mg/kg) (C), chlorpheniramine (histamine H1-receptor antagonist, 20 mg/kg) (D), cimetidine (histamine H2-receptor antagonist, 12.5 mg/kg) (E), flumazenil (GABAA/BDZ receptor antagonist, 5 mg/kg) (F) and cyproheptadine (serotonin receptor antagonist, 4 mg/kg) (G) on the antinociceptive activity of HECS in formalin-induced pain responses. Data are expressed as mean ± standard error of mean (n = 6 mice in each group). Data were analyzed using one-way ANOVA followed by Tukey’s HSD post-hoc test. HECS: hydroethanolic extract of Cynara scolymus. *P < 0.05: compared with control group in each phase. #P < 0.05: compared with HECS (200 mg/kg)-treated group in each phase.
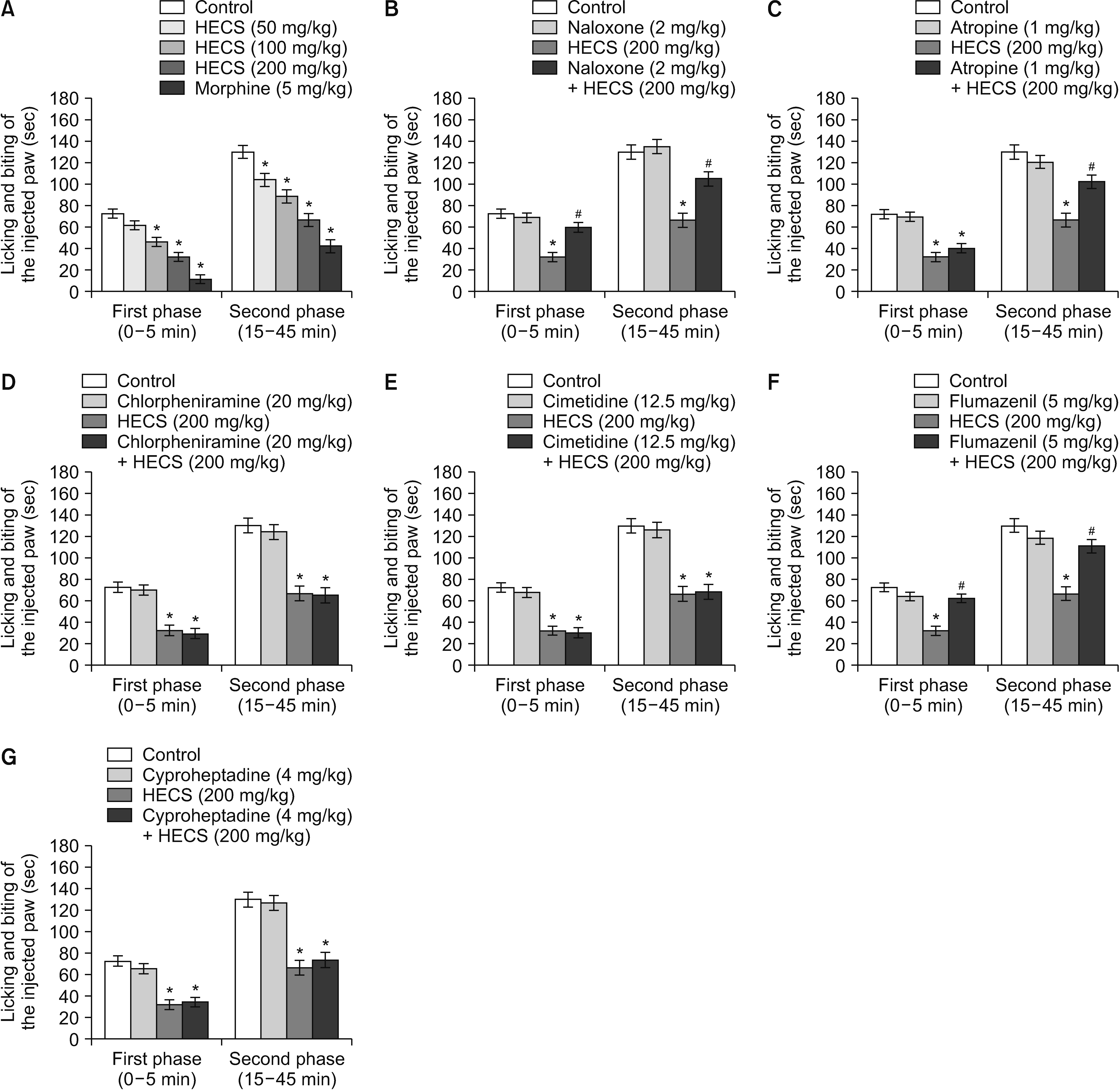
Fig. 2
Effect of HECS (50, 100 and 200 mg/kg) and indomethacin (5 mg/kg) on the acetic acid-induced writhing (A). Effect of pretreatment with naloxone (non-selective opioid receptors antagonist, 2 mg/kg) (B), atropine (non-selective muscarinic receptor antagonist, 1 mg/kg) (C), chlorpheniramine (histamine H1-receptor antagonist, 20 mg/kg) (D), cimetidine (histamine H2-receptor antagonist, 12.5 mg/kg) (E), flumazenil (GABAA/BDZ receptor antagonist, 5 mg/kg) (F) and cyproheptadine (serotonin receptor antagonist, 4 mg/kg) (G) on the antinociceptive activity of HECS in acetic acid-induced writhing. Data are expressed as mean ± standard error of mean (n = 6 mice in each group). Data were analyzed using one-way ANOVA followed by Tukey’s HSD post-hoc test. HECS: hydroethanolic extract of Cynara scolymus. *P < 0.05: compared with control group. #P < 0.05: compared with HECS (200 mg/kg)-treated group.
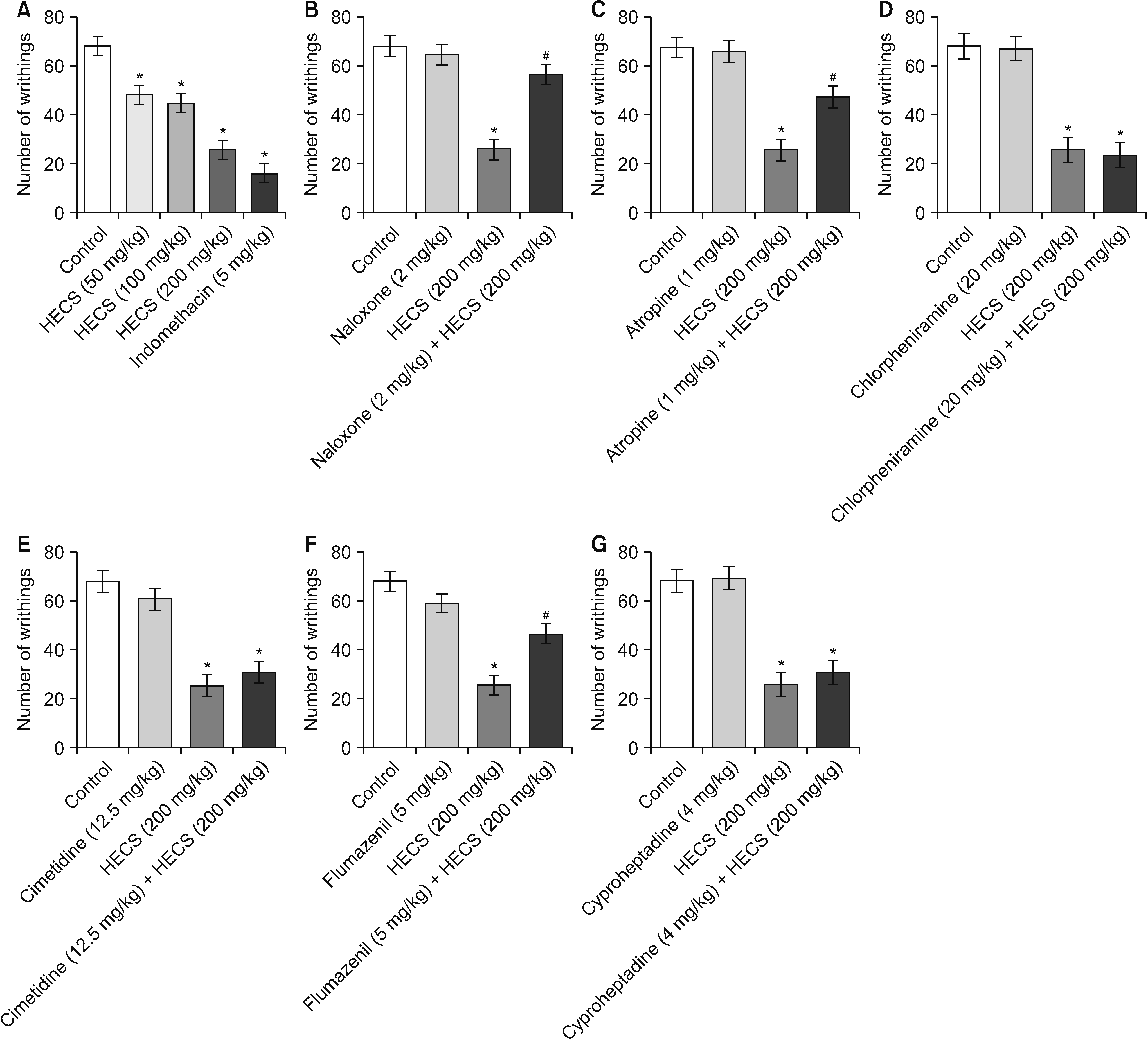
Fig. 3
Effect of treatment of mice with HECS (50, 100 and 200 mg/kg) on the number of crossings (A) and number of rearings (B) in the open-field test. Data are expressed as mean ± standard error of mean (n = 6 mice in each group). HECS: hydroethanolic extract of Cynara scolymus, i.p.: intraperitoneal.
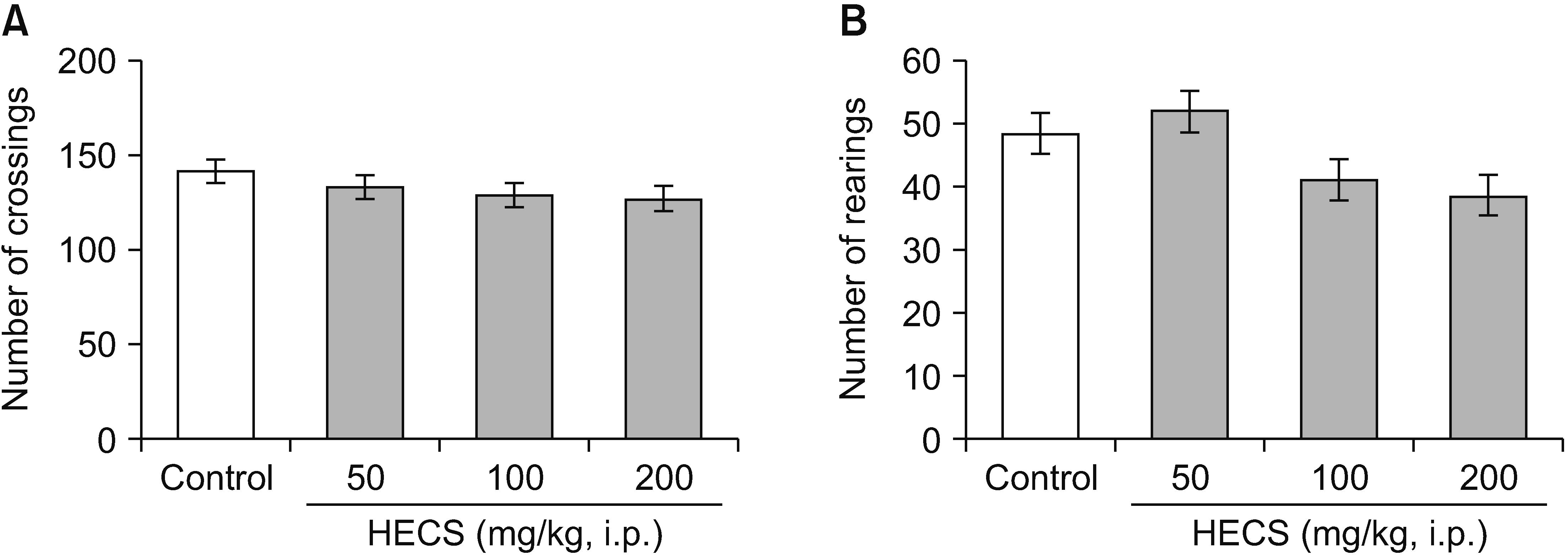