Abstract
Purpose
Materials and Methods
Results
Electronic Supplementary Material
Notes
Ethical Statement
The study protocol was approved by the Peking University People’s Hospital Ethics Committee (No. RDJP 2023-18) and conducted in accordance with the principles of the Declaration of Helsinki. Written informed consent was obtained from all patients or their guardians.
Author Contributions
Conceived and designed the analysis: Wang Y, Xue YJ, Zeng HM, Zhang LP.
Collected the data: Wang Y, Xue YJ, Zuo YX, Jia YP, Lu AD.
Contributed data or analysis tools: Wang Y, Xue YJ, Zuo YX, Jia YP, Lu AD.
Performed the analysis: Wang Y, Xue YJ, Zeng HM, Zhang LP.
Wrote the paper: Wang Y, Xue YJ.
ACKNOWLEDGMENTS
References



















Fig. 1.
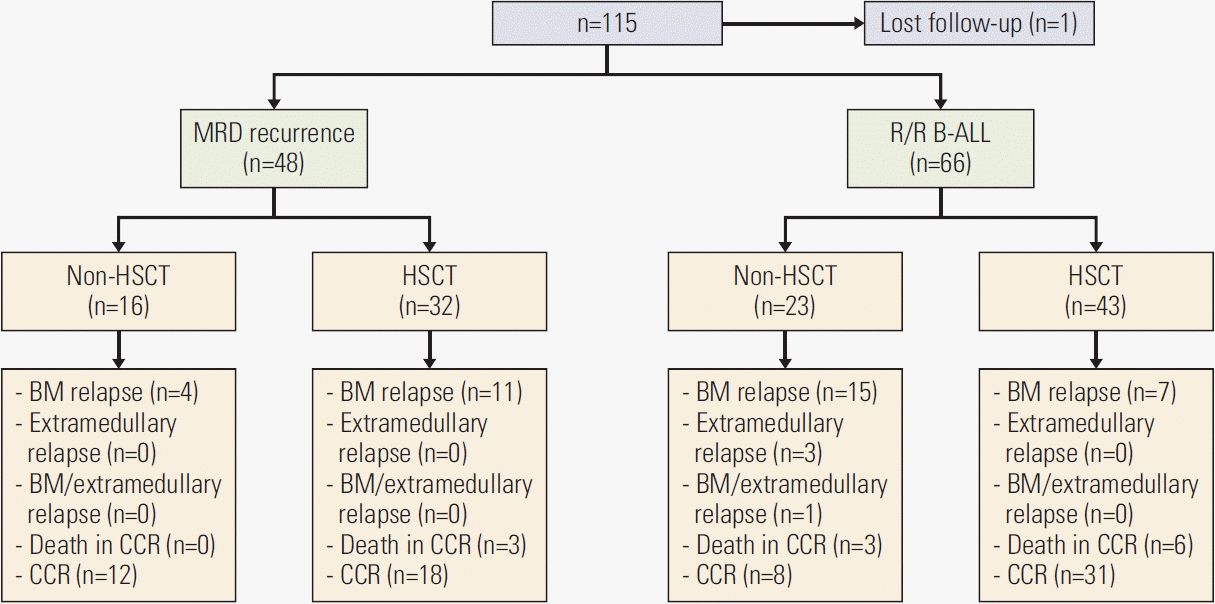
Fig. 2.
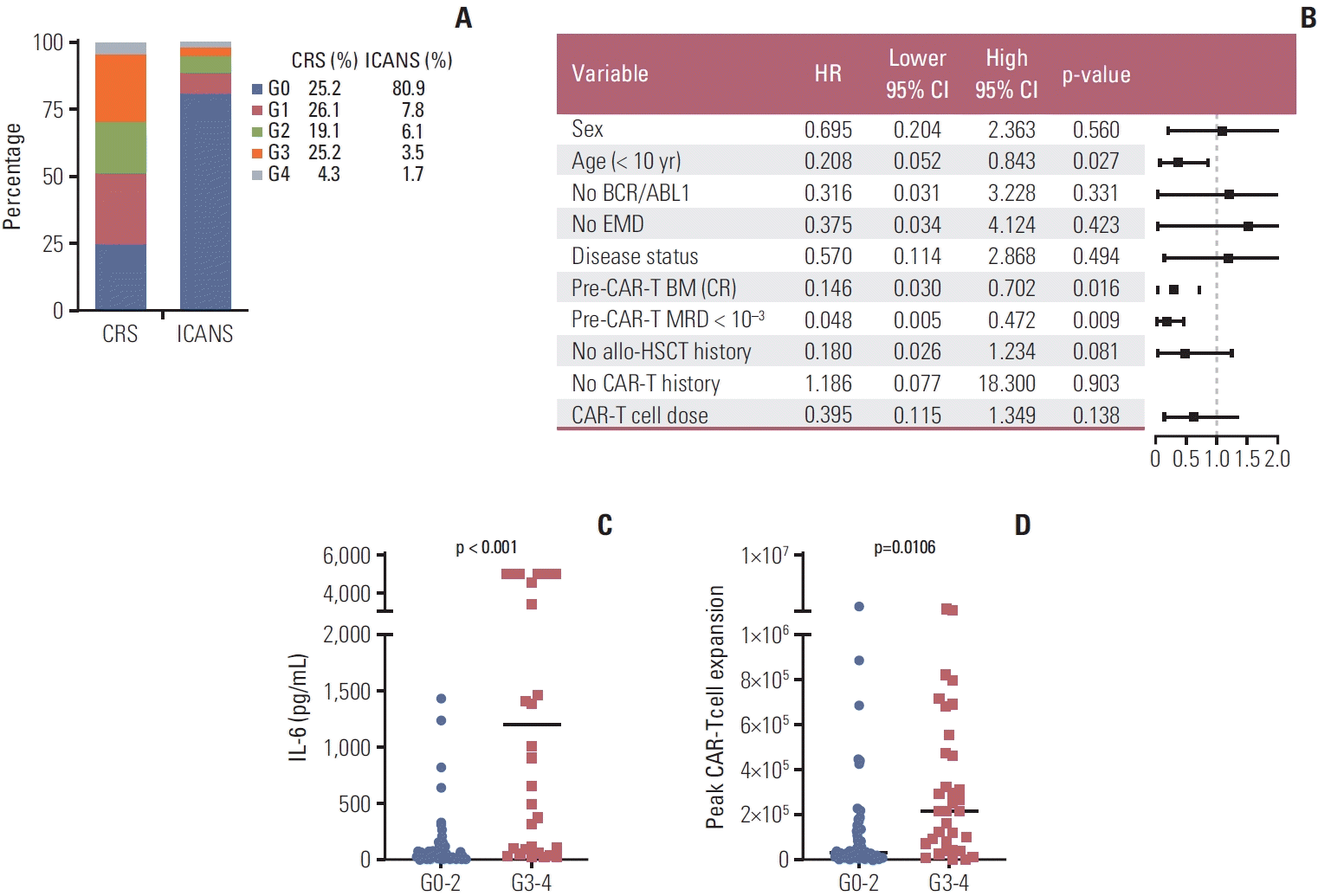
Fig. 3.
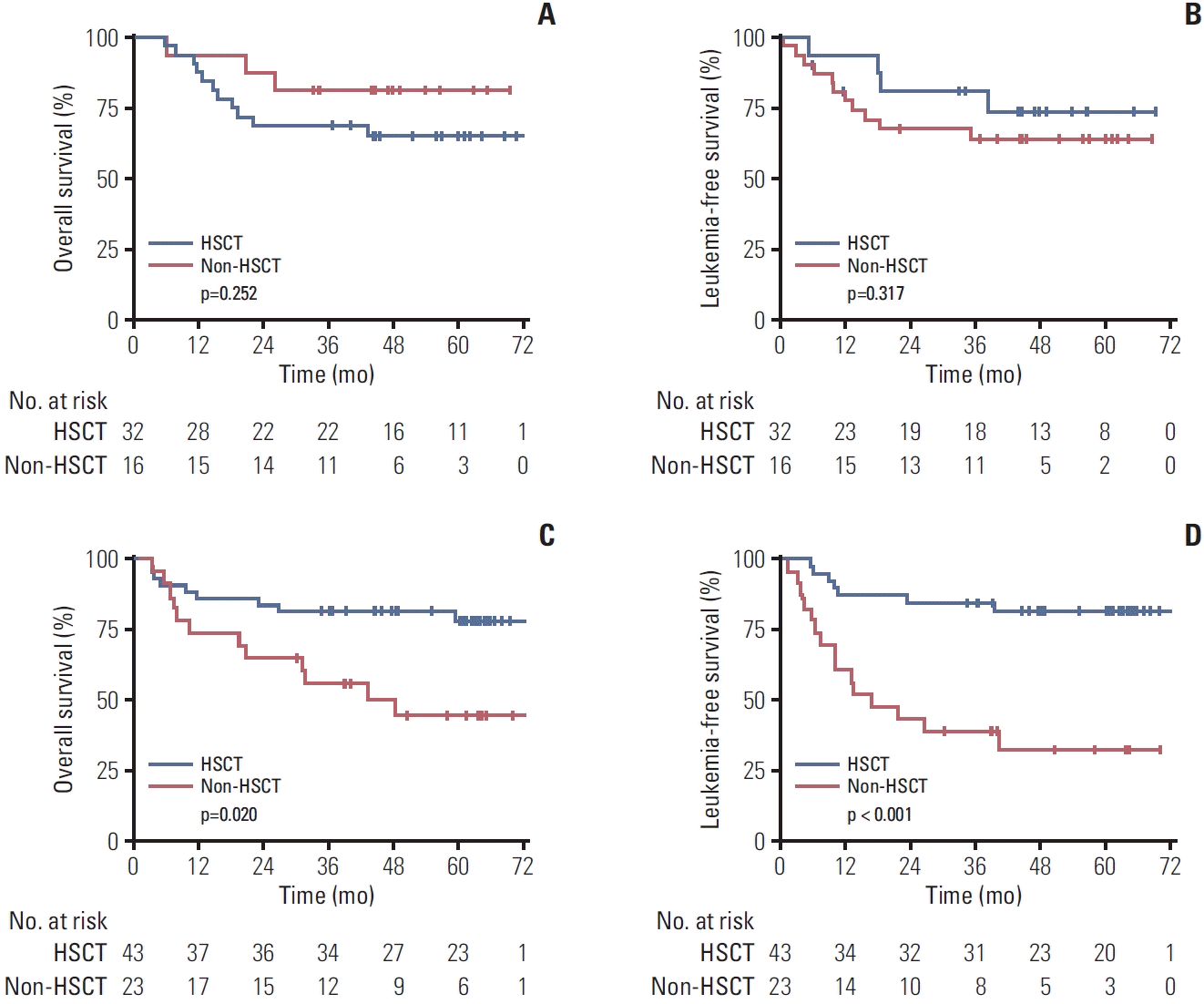
Table 1.
Characteristic | No. (%) | MRD re-emergence | R/R B-ALL | p-value | Bridging into allo-HSCT | Not bridging into allo-HSCT | p-value |
---|---|---|---|---|---|---|---|
No. | 115 (100) | 48 (41.7) | 67 (58.3) | - | 75 (65.8) | 39 (34.2) | - |
Sex | |||||||
Male | 63 (54.8) | 27 (56.2) | 36 (53.7) | 0.789 | 44 (58.7) | 18 (46.2) | 0.203 |
Female | 52 (45.2) | 21 (43.8) | 31 (46.3) | 31 (41.3) | 21 (53.8) | ||
Age (yr) | |||||||
< 10 | 69 (60.0) | 29 (60.4) | 40 (59.7) | 0.938 | 49 (65.3) | 20 (51.3) | 0.145 |
≥ 10 | 46 (40.0) | 19 (39.6) | 27 (40.3) | 26 (34.7) | 19 (48.7) | ||
Fusion genes | |||||||
BCR::ABL | 7 (6.1) | 2 (10.5) | 5 (22.7) | 0.259 | 5 (16.7) | 2 (18.2) | 0.813 |
ETV6::RUNX1 | 12 (10.4) | 6 (31.6) | 6 (27.3) | 8 (26.7) | 4 (36.4) | ||
KMT2Ar | 10 (8.7) | 7 (36,8) | 3 (13.6) | 7 (23.3) | 3 (27.3) | ||
TCF3::PBX1 | 12 (10.4) | 4 (21.1) | 8 (36.4) | 10 (33.3) | 2 (18.2) | ||
Complex cytogenetics | |||||||
Yes | 12 (10.4) | 6 (13.3) | 6 (10.7) | 0.686 | 9 (13.6) | 3 (8.8) | 0.483 |
No | 89 (77.4) | 39 (86.7) | 50 (89.3) | 57 (86.4) | 31 (91.2) | ||
EMD | |||||||
Yes | 12 (10.4) | - | 12 (17.9) | - | 7 (9.3) | 4 (10.3) | 0.874 |
No | 103 (89.6) | - | 55 (82.1) | 68 (90.7) | 35 (89.7) | ||
Disease status | |||||||
MRD re-emergence | 48 (41.7) | 48 (100) | - | - | 32 (42.7) | 16 (41.0) | 0.866 |
R/R ALL | 67 (58.3) | - | 67 (100) | 43 (57.3) | 23 (59.0) | ||
Pretreatment BM blasts by morphology | |||||||
CR | 88 (76.5) | 48 (100) | 40 (59.7) | - | 60 (80.0) | 27 (69.2) | 0.199 |
Non-CR | 27 (23.5) | - | 27 (40.3) | 15 (20.0) | 12 (30.8) | ||
Pretreatment MRD | |||||||
< 0.1% | 43 (37.4) | 28 (58.3) | 15 (22.4) | < 0.001 | 28 (37.3) | 15 (38.5) | 0.906 |
≥ 0.1% | 72 (62.6) | 20 (41.7) | 52 (77.6) | 47 (62.7) | 24 (61.5) | ||
Allo-HSCT history | |||||||
Yes | 14 (12.2) | 5 (10.4) | 9 (13.4) | 0.626 | 3 (4.0) | 11 (28.2) | < 0.001 |
No | 101 (87.8) | 43 (89.6) | 58 (86.6) | 72 (96.0) | 28 (71.8) | ||
CAR-T historya) | |||||||
Yes | 6 (5.2) | 2 (4.2) | 4 (6.0) | 0.997 | 2 (2.7) | 4 (10.3) | 0.085 |
No | 109 (94.8) | 46 (95.8) | 63 (94.0) | 73 (97.3) | 35 (89.7) | ||
Lymphodepletion | |||||||
Flu/Cy | 111 (96.5) | 45 (93.8) | 66 (98.5) | 0.391 | 71 (94.7) | 39 (100) | 0.142 |
Other chemotherapy regimens | 4 (3.5) | 3 (6.3) | 1 (1.5) | 4 (5.3) | - | ||
CAR-T origins | |||||||
Patients | 101 (87.8) | 43 (89.6) | 58 (86.6) | 0.626 | 72 (96.0) | 28 (71.8) | < 0.001 |
Donor | 14 (12.2) | 5 (10.4) | 9 (13.4) | 3 (4.0) | 11 (28.2) | ||
CAR-T cell dose | |||||||
< 4.03×106 cells/kg | 57 (49.6) | 27 (56.3) | 30 (44.8) | 0.225 | 40 (53.3) | 16 (41.0) | 0.212 |
≥ 4.03×106 cells/kg | 58 (50.4) | 21 (43.8) | 37 (55.2) | 35 (46.7) | 23 (59.0) | ||
CRS grade | |||||||
0-2 | 81 (70.4) | 40 (83.3) | 41 (61.2) | 0.010 | 60 (80.0) | 21 (53.8) | 0.003 |
3-4 | 34 (29.6) | 8 (16.7) | 26 (38.8) | 15 (20.0) | 18 (46.2) | ||
ICANS grade | |||||||
0-1 | 102 (88.7) | 47 (97.9) | 55 (82.1) | 0.008 | 67 (89.3) | 34 (87.2) | 0.731 |
2-4 | 13 (11.3) | 1 (2.1) | 12 (17.9) | 8 (10.7) | 5 (12.8) |
BM, bone marrow; CAR-T, chimeric antigen receptor-T lymphocytes; CR, complete remission; CRS, cytokine release syndrome; EMD, extramedullary disease; HSCT, hematopoietic stem cell transplantation; ICANS, immune effector cell-associated neurotoxicity syndrome; MRD, minimal residual disease; R/R B-ALL, refractory/relapse B-cell acute lymphoblastic leukemia.
Table 2.
Values are presented as mean±SE. BM, bone marrow; CAR-T, chimeric antigen receptor-T lymphocytes; CR, complete remission; CRS, cytokine release syndrome; EMD, extramedullary disease; HSCT, hematopoietic stem cell transplantation; ICANS, immune effector cell-associated neurotoxicity syndrome; LFS, leukemia-free survival; MRD, minimal residual disease; OS, overall survival; R/R B-ALL, refractory/relapse B-cell acute lymphoblastic leukemia; SE, standard error.
Table 3.
BM, bone marrow; CAR-T, chimeric antigen receptor-T lymphocytes; CI, confidence interval; CR, complete remission; CRS, cytokine release syndrome; HR, hazards ratio; HSCT, hematopoietic stem cell transplantation; ICANS, immune effector cell-associated neurotoxicity syndrome; LFS, leukemia-free survival; MRD, minimal residual disease; OS, overall survival.